
Influence of hydrothermal temperature on structure and microstructure of boehmite
FU Gao-feng(付高峰)1, WANG Jing(王 晶)2, XU Bing(徐 冰)2,
GAO Hong(高 宏)2, XU Xiu-lin(徐秀林)2, CHENG Hao(成 豪)1
1. School of Materials and Metallurgy, Northeastern University, Shenyang 110004, China;
2. College of Materials Science and Engineering, Dalian Jiaotong University, Dalian 116028, China
Received 6 July 2009; accepted 30 December 2009
_____________________________________________________________________________________________________
Abstract: Boehmites (Al2O3·xH2O) were hydrothermally prepared from aluminum alkoxide. The effect of temperature on preparation was studied in the range of 100-180 ℃. The XRD analysis shows that with temperature increasing, the transformation of pseudo-boehmite into well-crystallized boehmite takes place. The micro-morphologies change also from irregular clew to thin cubic platelets. From the mass loss of the samples prepared at different temperatures, the value of x is estimated to vary between 1.06 and 1.67. γ-Al2O3 obtained by subsequent calcination of boehmite at 600 ℃ is also characterized by XRD and its morphology remains unchanged.
Key words: boehmite; hydrothermal synthesis; alkoxide; microstructure; morphology
_____________________________________________________________________________________________________
1 Introduction
Among metal oxides, alumina is the most common catalyst and catalyst support used in heterogeneous catalysis due to its low cost, good thermal stability, high specific surface area, surface acidity and interaction with deposited transitional metals[1-4]. Precipitation, drying and calcination of aluminum oxy-hydroxides are the most commonly used methods to produce alumina in industry. The applications of alumina materials require that their precursor hydroxides are free of impurities, like sodium or iron[5]. To understand the evolution of the alumina catalytic properties, it is necessary to prepare catalysts with well-known structure and texture. In this way, great efforts have been carried out for controlling the crystalline structure of the alumina as well as its surface properties. By changing the synthesizing conditions, for example, these properties can vary with the alumina hydration degree or the impurities incorporated into its crystalline structure[6].
Among the synthesis methods, the alkoxide sol-gel method allows the control of the textural and structural properties of the solid, i.e. the specific surface area, pore size and solid purity. Despite the efforts for using the technique to prepare materials of catalytic interest, they are not yet as mature as those obtained for glasses and ceramics[7].
Boehmite (γ-AlOOH) is the most commonly used precursor for preparing high-purity and high-strength monolithic α-Al2O3 ceramics by sol-gel technology. Microstructurally controlled boehmite-derived high- purity α-Al2O3 ceramics with enhanced mechanical and thermo-mechanical stability, chemical inertness, optical and electrical properties, are used in a variety of applications, such as substrates for electronic circuits, abrasive grains, high-temperature refractory materials, fibers and thin films[8-13]. Boehmite is also a crucial precursor for preparing γ-Al2O3, which is used as a high-temperature catalyst support and as a membrane due to its high surface area and mesoporous properties.
Synthesis under hydrothermal conditions offers some significant advantages over other chemical synthesizing techniques[14]. Firstly, it is easy to control particle size and morphology by varying the synthesizing conditions. Secondly, many materials can be synthesized directly in the desired crystalline phase at low temperature. Finally, a substance with an elemental oxidation state can be produced. Since the particles are produced in a sol form, the resultant sol can be used directly in producing green bodies using pressure filtration or extrusion. The chemically synthesized nanostructural powders using the hydrothermal route possess better chemical homogeneity and a finer particle size, together with better control of particle morphology due to nucleation and particle growth that occur under these conditions. These characteristics enhance the sinterability of ultra-fine ceramic powders at lower temperatures, and also produce a uniform fine grained sintered microstructure possessing better mechanical or electrical properties.
In this study, the boehmite is synthesized under hydrothermal conditions with water as mineralizer and the annealing temperature from 100 ℃ to 180 ℃. Samples are characterized by X-ray powder diffractometry, thermo-gravimetry and transmission electron microscopy.
2 Experimental
2.1 Sample preparation
Boehmite sol was prepared by hot water hydrolysis of aluminum isopropoxide. The molar ratio of alkoxide to water was maintained at 1?3. Hydrolysis was carried out with distilled water by stirring for 1 h at 80 ℃. The hydrolysis was performed under reflux conditions, resulting in no loss of the material. After hydrolysis the hydrothermal treatment was performed. The hydrolyzed product without any additive was put into the vessel of the autoclave and heated at different temperatures for 24 h under autogenous pressure. After hydrothermal annealing, the corresponding product was dried overnight at 100 ℃ in air. Then, the obtained gel was further annealed in air up to 600 ℃ at heating rate of 10 ℃/min. At this temperature, they were eventually calcined for 4 h to get γ-Al2O3.
2.2 Characterization
X-ray diffraction patterns of the samples packed in a glass holder were recorded at room temperature with Cu Kα radiation in a Bruker Advance D-8 diffractometer. Diffraction intensity was measured between 20? and 80?, with a 2θ step of 4 (?)/min.
The mass loss and temperatures associated with the phase transformations were determined by thermo- gravimetry with a Perkin-Elmer TG-7 apparatus from room temperature up to 800 ℃ with heating rate of 10 ℃/min.
The samples milled and dispersed in ethanol before supporting them in the copper grid covered with formvar were observed in H-600 transmission electron microscope (SEM) that had high-resolution polar pieces.
3 Results and discussion
3.1 XRD analysis
Fig.1 shows the XRD patterns of pseudo-boehmite hydrothermally treated in water at different temperatures for 24 h. The XRD patterns have two clear differences. Firstly, the peaks of the diffraction pattern of the samples annealed below 140 ℃ are broad but well-defined, and the (002) peak is absent, which is the characteristic for boehmite. This reflects a poor ordering along the b-axis. Secondly, new (220), (231) and (002) peaks of boehmite appear above 140 ℃ and the (231) peak at 64.66? spilt into double peaks.
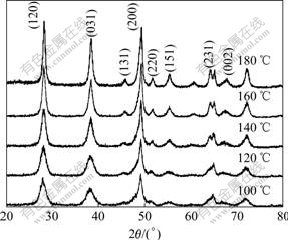
Fig.1 XRD patterns of boehmite prepared under different hydrothermal temperatures
The crystallite sizes were estimated using the Scherrer equation: D=0.9λ/B1/2cosθ, where λ is the wavelength of X-ray, θ is the Bragg angle and B1/2 is the pure full width of the diffraction line at half of the maximum intensity. The estimated values of the crystallite sizes are given in Tables 1 and 2.
Table 1 Crystallite dimensions d(120) perpendicular to planes
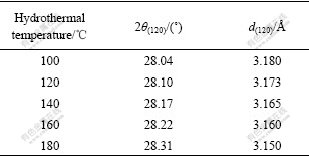
Table 2 Crystallite dimensions of planes (200) and (002)
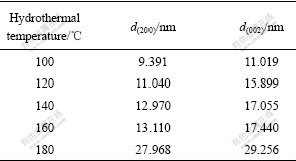
In Table 1, the (120) reflections shift to higher diffraction angles (Fig.1) with respect to the increased annealing temperature. Since (120) planes are related to the hydrogen bonding, a larger distance between (120) planes corresponds to a weaker hydrogen bonding and consequently to a lower transition temperature of boehmite into γ-Al2O3, because this temperature depends on the hydrogen bonding. This result is in accordance with the thermo-gravimetric analysis of the samples hydrothermally treated at different temperatures (Fig.2), which clearly shows that the transition temperature is much lower for the samples annealed at low temperature than that annealed at high temperature.
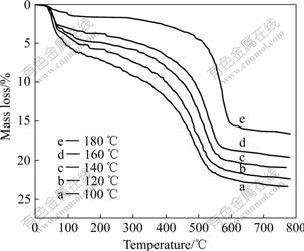
Fig.2 TG curves of samples hydrothermally treated at different temperatures
Boehmite is composed of layers. Planes parallel to the layers are perpendicular to b-axis and perpendicular to planes of (200) and (002) also. So, the layer plane size is d(200)×d(002). In Table 2, the size of d(200)×d(002) increases with the hydrothermal temperature increasing. After hydrothermal treatment at 180 ℃, the size of layer plane is about 28 cm×30 nm. Crystallization and the crystallite size of the sample depend on the hydrothermal temperature. The sample crystallization increases and the crystallites grow as temperature increases.
3.2 TG Analysis
Fig.3 shows the TG curves of the samples hydrothermally treated at 180 ℃ and unhydrothermally treated. For untreated sample, two distinct steps of mass loss are discernible, in the temperature ranges <250 ℃ and 250-600 ℃. Step Ⅰ (below 250 ℃) corresponds to the removal of water loosely bound to the material. Step Ⅱ(250-600 ℃) can be assigned to the dehydroxylation of boehmite. The total mass loss is about 30%. The chemical formula can be Al2O3?2.3H2O. For the sample treated at 180 ℃, only one dramatic mass loss takes place during 400-600 ℃. The total mass loss is about 16%. The chemical formula can be Al2O3?1.06H2O. Compared Fig.2 with Fig.3, it is observed that as the annealing temperature increases, step Ⅰ disappears and H2O content changes from 1.67 to 1.06. The results prove that the physical in adsorbed water decreases as the annealing temperature increases.
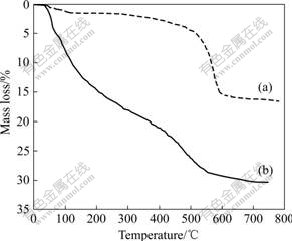
Fig.3 TG curves of samples hydrothermal treated at 180 ℃ (a) and unhydrothermal treated (b)
3.3 TEM analysis
The morphologies of samples untreated and treated at different temperatures are shown in Fig.4. The morphology of the untreated sample is irregular clew. After hydrothermal treatment, the morphology changes into cubic thin flat plates and the size increases with the treated temperature increasing. Heavy agglomeration takes place in the untreated sample. For the hydrothermally treated sample, single crystals separate well. Figs.5 and 6 show the XRD patterns and TEM images of the samples calcined at 600 ℃.
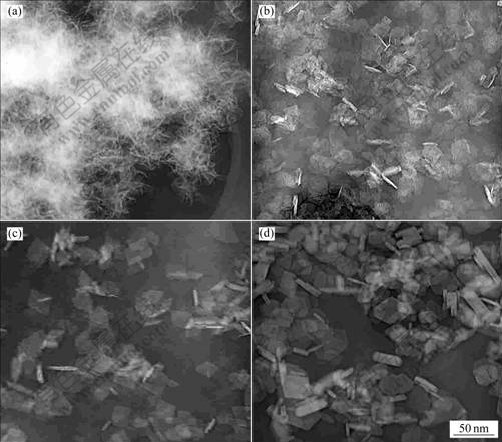
Fig.4 Morphologies of samples untreated and treated at different temperatures: (a) Unhydrothermally treated; (b) Hydrothermally treated at 140 ℃; (c) Hydrothermally treated at 160 ℃; (d) Hydrothermally treated at 180 ℃
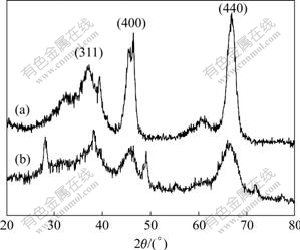
Fig.5 XRD patterns of samples calcined at 600 ℃: (a) Hydrothermally treated at 180 ℃; (b) Untreated
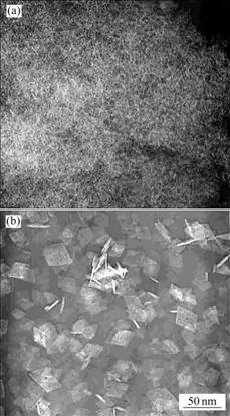
Fig.6 TEM images of samples calcined at 600℃: (a) Unhydro- thermally treated; (b) Hydrothermally treated at 180 ℃
When the sample is heated at 600 ℃, it transforms into γ-Al2O3 (Fig.5). For the unhydrothermally treated sample, the peak is broad. Peaks become sharp and some peaks disappear for the hydrothermally treated sample. The morphology of γ-Al2O3 crystallites is inherited from that of boehmite crystallites (Fig.6). The morphology of γ-Al2O3 for untreated sample still keeps the shape of irregular clew, while for treated samples the shape of thin cubic platelets remains.
4 Conclusions
1) Boehmite crystallizes when it is annealed under hydrothermal conditions with water as mineralizer. Boehmite’s crystallite dimensions grow with the annealing temperature increasing.
2) The samples without hydrothermal treatment consist of irregular clew-like pseudo-boehmite crystallites, characterized by a weak hydrogen bonding, which transform into thin flat crystalline plates perpendicular to the b-axis when they are annealed under hydrothermal conditions.
3) Hydrogen bonding length decreases as the crystallite size increases, which determines the variation of the transition temperature of boehmite into γ-Al2O3.
4) The morphology of γ-Al2O3 crystallites is inherited from that of boehmite crystallites.
References
[1] KOTANIGAWA T, YAMAMOTO M, UTIYAMA M, HATTORI H, TANABE K. The influence of preparation methods on the pore structure of alumina[J]. Applied Catalysis, 1981, 1(7): 185-200.
[2] BEGUIN B, GARBOWSKI E, PRIMET M. Stabilization of alumina toward thermal sintering by silicon addition [J]. Journal of Catalysis, 1991, 127(2): 595-604.
[3] PENG X S, ZHANG L D, MENG G W. Photoluminescence and infrared properties of α-Al2O3 nanowires and nanobelts[J]. J Phys Chem B, 2006, 106(43): 11163-11167.
[4] LEE H C, KIM H J, CHUNG S H. Synthesis of unidirectional alumina nanostructures without added organic solvents[J]. J Am Chem Soc, 2003, 125(10): 2882-2883.
[5] BOKHIMI X, S?NCHEZ-VALENTE J, PEDRAZA F. Crystallization of sol-gel boehmite via hydrothermal annealing[J]. Journal of Solid State Chemistry, 2002, 166(1): 182-190.
[6] ?EJKA J. Organized mesoporous alumina: Synthesis, structure and potential in catalysis[J]. Applied Catalysis A: General, 2003, 254: 327-338.
[7] MUSI? S, DRAG?EVI? ?, POPOVI? S. Hydrothermal crystallization of boehmite from freshly precipitated aluminium hydroxide[J]. Mater Lett, 1999, 40(6): 269-274.
[8] MISHRA D, ANAND S, PANDA R K, DAS R P. Hydrothermal preparation and characterization of boehmites[J]. Mater Lett, 2000, 42(1/2): 38-45.
[9] TSUCHIDA T. Hydrothermal synthesis of submicrometer crystals of boehmite[J]. Journal of the European Ceramic Society, 2000, 20(11): 1759-1764.
[10] GAO P, XIE Y, CHEN Y. Large-area synthesis of single-crystal boehmite nanobelts with high luminescent properties[J]. J Cryst Growth, 2005, 285(4): 555-560.
[11] ZHANG J, LIU S J, LIN J. Self-assembly of flowerlike AlOOH (Boehmite) 3D nanoarchitectures[J]. J Phys Chem B, 2006, 110(29): 14249-14252.
[12] LIU Y, MA D, HAN X W. Hydrothermal synthesis of microscale boehmite and gamma nanoleaves alumina[J]. Mater Lett, 2008, 62(8/9): 1297-1301.
[13] KUANG D, FANG Y, LIU H. Fabrication of boehmite AlOOH and γ-Al2O3 nanotubes via a soft solution route[J]. J Mater Chem, 2003, 13: 660-662.
[14] KAYA C, HE J Y, GU X, BUTLER E G. Nanostructured ceramic powders by hydrothermal synthesis and their applications[J]. Microporous and Mesoporous Materials, 2002, 54: 37-49.
____________________________
Foundation item: Project (50974026) supported by the National Natural Science Foundation of China
Corresponding author: WANG Jing; Tel: +86-411-84109776; Fax: +86-411-84106345; E-mail: wangjing@djtu.edu.cn
(Edited by YUAN Sai-qian)