J. Cent. South Univ. Technol. (2009) 16: 0392-0398
DOI: 10.1007/s11771-009-0066-7

Non-isothermal curing kinetics and thermal properties of
benzoxazine-phenolic copolymers
XU Li(徐 丽), SITU Yue(司徒粤), HU Jian-feng(胡剑峰), ZENG Han-wei(曾汉维), CHEN Huan-qin(陈焕钦)
(School of Chemistry and Chemical Engineering, South China University of Technology, Guangzhou 510640, China)
Abstract: Using novolac phenolic resin, aniline and formaldehyde as raw materials, benzoxazine-phenolic copolymers with different percentages of benzoxazine rings were prepared. FT-IR was adopted to characterize the molecular structure of the novolac-type phenolic resin and the benzoxazine-phenolic copolymer BP31. In order to understand the curing process of the copolymers, the curing behavior and curing kinetic characteristics were studied by differential scanning calorimetry (DSC), and the catalytical effect of phenolic hydroxyl on the curing behavior of copolymers was investigated. To investigate the thermal properties of this resin, the thermal degradation behaviors of the cured samples were studied by thermal gravimetric (TG) method, and glass-transition temperatures (Tg) of the cured copolymers were also evaluated by DSC. The dynamic Ozawa method was adopted to determine the kinetic parameters of the curing process as well. The activation energy is 78.8 kJ/mol and the reaction rate constant is in the range from 40.0 to 5.2 (K/min)n according to reaction temperatures. The Ozawa exponent decreases from 2.4 to 0.7 with the increase of reaction temperature, and curing mechanism is expounded briefly according to the results. TG result shows that the highest char yield of copolymers is 50.3%. The highest Tg of copolymers is 489 K, which is much higher than that of pure benzoxazine resin.
Key words: benzoxazine; novolac-type phenolic resin; copolymer; cure kinetics; thermal degradation; Ozawa method; crosslinking
1 Introduction
A series of polybenzoxazines obtained by the ring-opening polymerization of cyclic aromatic oxazines and benzoxazines, were developed as a novel type of phenolic resin [1-4]. The polymerization proceeds through ring-opening of oxazine rings without using strong acid catalyst and without generating any byproducts. Polybenzoxazines possess typical characteristics of the traditional phenolic resins such as good heat resistance, flame retardance and electronic properties. In addition, they provide unique characteristics like low water absorption and excellent dimensional stability due to the near-zero volumetric shrinkage upon cure, which cannot be found in the traditional phenolic resins. Benzoxazine monomers can be prepared from inexpensive raw materials including phenols, primary amines and aldehydes. Various raw materials allow considerable molecular-design flexibilities for the monomers. These flexibilities provide possibility of synthesizing a wide range of benzoxazine monomers with additional functional groups, such as acetylene, nitrile, propargyl and maleimide groups [5-11]. The introduction of functional groups can improve the physical properties of benzoxazine such as mechanical properties and thermal stability. But the application of this kind of resin is limited due to the high cost and complex synthetic routes. The obvious disadvantages of traditional benzoxazine-based resins are their high cure temperature and low crosslinking density. Some researchers try to catalyze the ring opening of oxazines and lower the cure temperature of benzoxazine by adding some compounds containing active hydrogen during the synthesis and curing processes [12-15]. However, only a few of people study on lowering the high curing temperature of benzoxazine by choosing suitable raw materials.
In this work, novolac phenolic resin was used as phenolic hydroxyl provider to synthesize benzoxazine- phenolic copolymers. The phenolic hydroxyl of novolac phenolic resin can lower the ring opening temperature of oxazines and the structure of phenolic resin can give the obtained benzoxazine higher crosslinking density. To make optimum application of the copolymers, it is important to understand the nature of their curing process. The final properties of the crosslinked copolymers significantly depend on the kinetics of the curing reaction concerned with extent of curing, and the curing conditions, etc [16-17]. Therefore, the study of the curing kinetics contributes to both a better knowledge of process development and an improvement of the quality of final products related to the structures of the polymer network. Under the condition of without external catalyst,the effect of unreacted phenolic hydroxyl on the curing behavior of the obtained copolymers was studied, and the curing kinetics of the resins with certain amount of oxazines was also investigated thoroughly. Finally, the thermal properties and glass-transition temperatures (Tg) of the cured copolymers were evaluated in details.
2 Experimental
2.1 Materials
Aniline was purchased from Tianjin Kermel Chemical Reagent Co Ltd, China; phenol and acetone were analytical grade, which were purchased from Guangzhou Guanghua Chemical Factory, China; formaldehyde (mass fraction of 37%, aqueous) was supplied by Chemical Factory of Hubei University, China; and novolac-type phenolic resin was prepared and purified in our laboratory.
2.2 Synthesis of benzoxazine-based phenolic resins
Novolac-type phenolic resin (10 g) was mixed with different amounts of aniline and formaldehyde aqueous solution to prepare benzoxazine-phenolic copolymers. The procedure was as follows: the molar ratio of aniline to formaldehyde was fixed at 1/2. Aniline was first uniformly mixed with novolac resin at the molar ratio of 3/1, 2/1 and 1/1 (the final products were designated as BP31, BP21 and BP11, respectively). Then the obtained mixture was added dropwise into formaldehyde solution contained in a 200 mL round-bottomed flask, and 70 mL of methanol was added subsequently. The mixture was heated at 373 K for 1 h. The methanol was removed at low pressure and the modified resin was washed with 3 mol/L NaOH aqueous solution and 1 mol/L HCl aqueous solution, and then washed several times with deionized water. The resin was filtered and dried under vacuum, and fine orange powder was obtained.
2.3 Synthesis of phenol-aniline-based benzoxazine monomer(PA)
The phenol-aniline-based monomer (PA) was synthesized by a solvent process [18]. 0.1 mol phenol and 0.2 mol formaldehyde dissolved in 50 mL 1, 4-dioxane were added to a 250 mL reaction vessel equipped with a thermometer, overhead stirrer, and a condenser, and cooled in an ice bath. Then a mixed solution of 0.1 mol aniline and 15 mL 1, 4-dioxane was added dropwise into the vessel. The reaction mixture was heated to reflux temperature and maintained at that temperature for 5 h to complete the reaction. The solvent was removed under vacuum, and the residual yellow solid was collected. The solid was dissolved in 150 mL ethyl ether and washed several times with deionized water after washing with 3 mol/L NaOH solution and 1 mol/L HCl solution, respectively. The yellowish powder of phenol-aniline-based benzoxazine was obtained.
2.4 Characterization
FT-IR measurement was carried out on a Perkin-Elmer IR2000 spectrometer. Infrared spectra of novolac-type phenolic resin and the copolymer were obtained in the range of 4000-450 cm-1.
NETZSCH STA499C (TG/DSC) simultaneous thermal analyzer was used to study the curing behavior of the benzoxazine-phenolic copolymers. A nonisothermal experiment was carried out at 5, 10, 15 and 20 K/min from 300 to 623 K and the area of exothermic peak was integrated. The differential scanning calorimetry (DSC) curves were analyzed according to Ozawa’s theory to obtain the kinetic parameters.
The thermal degradation behaviors of the cured samples were studied with thermal gravimetry (TG) from 300 to 973 K at a heating rate of 15 K/min in a nitrogen atmosphere. The samples were cured at 463 K for 2 h. NETZSCH STA499C (TG/DSC) thermal analyzer was used for recording TG traces.
3 Results and discussion
3.1 FT-IR spectroscopy analysis
Fig.1 shows the FT-IR spectra of novolac-type phenolic resin and benzoxazine-phenolic copolymers. Curve (a) is the spectrum of novolac-type phenolic resin, peaks at 819 and 756 cm-1 respectively correspond to the characteristic absorption bands of phenol with 2, 4 and 2, 6 substitution. In the FT-IR spectrum of benzoxazine-phenolic copolymer BP31 (Curve (b)), a new absorption band at 945 cm-1 corresponds to the benzoxazine ring appears [19]. Besides, the ortho position of phenol hydroxyl is occupied because of the
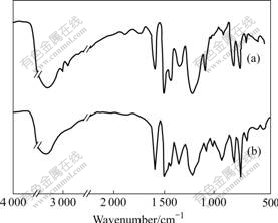
Fig.1 FT-IR spectra of novolac-type phenolic resin (a) and benzoxazine-phenolic copolymer BP31 (b)
formation of benzoxazine ring, so the strength of absorption at 756 cm-1 increases, and the peak of hydroxyl at 3 375 cm-1 becomes weakened.
3.2 Effect of phenolic groups on curing temperature
In order to investigate the phenol-initiated crosslinking of benzoxazine-phenolic copolymers, their curing behaviors were monitored by dynamic DSC. Fig.2 shows the maximum temperatures of the crosslinking exotherms for samples BP31, BP21, BP11 and PA at a heating rate of 10 K /min. Obviously, PA possesses the highest curing peak temperature. For the three copolymers, with increasing benzoxazine rings percentage, the peak temperature of the crosslinking exotherm becomes higher. The results suggest that free phenolic groups in the copolymer structure can catalyze the ring-opening of benzoxazine, and lower curing temperature of the copolymer [20]. Fig.2 also shows that the scanning peak of curing temperature becomes wider with decreasing content of oxazine ring. Explanation of this phenomenon is that curing process of the copolymer includes not only ring-opening of benzoxazine, but also curing reaction of novolac phenolic resin. The curing of phenolic resin is catalyzed by tertiary amine group generated after the ring-opening of benzoxazine, so the smaller the content of benzoxazine, the longer the curing process. This means that intense heating discharge can be avoided during the reaction, and the reaction system can be controlled much more easily under long curing process.
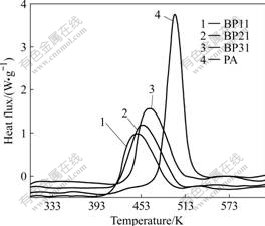
Fig.2 Dynamic DSC thermograms of benzoxazine-phenolic copolymers
3.3 Curing kinetic analysis of benzoxazine-based novolac-type phenolic resins
Curing of thermosetting materials generally involves the transformation of low-molecular monomers and prepolymers to high-molecular amorphous solids by chemical reactions. It is supposed that the exothermic heat released during cure is proportional to the chemical conversion. Based on this assumption, the relative degree of conversion (α) is normally defined as
(1)
where ?Hp denotes the partial heat of reaction up to time t, and ?H0 denotes the total heat of the reaction.
In this work, copolymer BP31 was chosen as the sample for curing kinetic study. Fig.3 shows the DSC thermograms for copolymer BP31 recorded at heating rates of 5, 10, 15, and 20 K/min. From this figure, mechanism parameters of the curing reaction such as initial curing temperatures, peak temperatures, and the curing ranges of copolymer BP31 at different heating rates can be derived. It can be observed that the exothermic peak shifts to a higher temperature with a higher heating rate. Fig.4 shows the variation of the degree of conversion (α) as a function of curing temperature obtained from dynamic DSC. All curves are in typical sigmoid shape, which indicates that the curing reaction of the system follows autocatalytic mechanism.
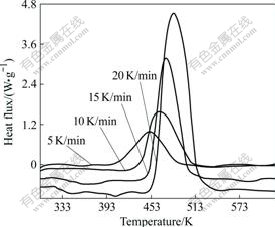
Fig.3 Dynamic DSC thermograms of copolymer BP31
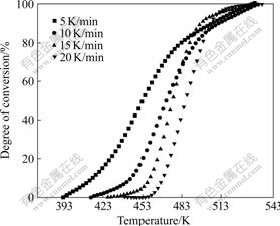
Fig.4 Relative degree of conversion as function of curing temperature and heating rates
Because the change of peak temperature in DSC experiments is closely related to the corresponding heating rates [21], the kinetic parameters can be determined by Flynn-Wall-Ozawa (FWO) method, which is widely used to the dynamic kinetic analysis. In this method, it is assumed that conversion function f(α) does not change with the alteration of the heating rate for all values of α. With this assumption, the reliable activation energy can be determined without a precise understanding of the reaction mechanism. Its general expression can be represented by
(2)
where β is the heating rate, A is the preexponential factor, E is the activation energy, Tp is the peak temperature obtained from multiple rate scans, and R is the gas constant. A straight line is obtained by plotting ln β vs 1/Tp (see Fig.5). The value of the slope yields -E/R and the activation energy is calculated to be 78.8 kJ/mol, while the activation energy of pure benzoxazine cured without additional catalyst is calculated to be about 102-116 kJ/mol [22], which means that the phenolic-hydroxyl makes the curing reaction much easier. This conclusion also validates that the curing reaction of copolymer BP31 is auto-catalyzed by the phenolic-hydroxyl.
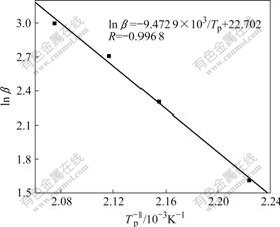
Fig.5 Curve of ln β vs 103/Tp from FWO method for thermal curing of copolymer BP31
The most common approach used to describe the crystallization kinetics of polymers is the Avrami equation that is based on the phase change theory:
(3)
where α(t) is the relative degree of curing as a function of time, k is the effective overall reaction rate, t is the time taken during the curing process (incubation time t0 is excluded), and n is the Avrami exponent or reaction order that depends on the mechanism of crystal growth.
Broadly speaking, the crystallization is a process of physical crosslinking [23-24]. From certain point of view, the curing process of thermosetting polymer is similar to the crystallization process. Therefore, Avrami equation can be used to represent curing process of thermosetting resin. OZAWA [25-26] extended the Avrami theory to describe the nonisothermal case. Kinetic parameters (reaction order n and the activation energy E) of this kind of resin were determined by the relationship between peak temperature of the reaction exotherms and heating rates. If the temperature is changed by a constant heating rate, the variation in degree of curing can be written as a function of the heating rate as follows
(4)
where? k0 is the Ozawa reaction rate constant, n0 is the Ozawa exponent that is similar to the Avrami exponent.
The data analysis can be performed through a double-logarithmic plot of ln {-ln[1-α(t)]} vs ln β at a fixed temperature. Parameter k0 is taken as the antilogarithmic value of the y-intercept and n0 is the negative value of the slope calculated from least square lines drawn through plots of at least three points. This means at least three different heating rates should be used in DSC experiments to obtain the Ozawa parameters. As shown in Fig.6, a straight line is obtained by plotting ln {-ln[1-α(t)]} vs ln β, which is based on the data in Fig.4. The Ozawa exponent n0 is calculated from the negative values of the lines’ slopes. Fig.7 shows the Ozawa exponent variation as a function of temperature.
It is observed that the Ozawa exponent decreases from about 2.4 to 0.7 with increasing temperature, which implies that the fast initial stage of curing is replaced by a diffusion-controlled reaction. As the curing temperature increases, the curing reactions start and the benzoxazine ngs open under the catalysis of phenol hydroxyl. The intermediates of ring-opened benzoxazine can react with free ortho position on benzene ring of the copolymer system to extend the polymer chain and form crosslink with high density. Initially, these intermediates behave like microgels that grow without restraint during the initial stage of curing process. As the reaction proceeds, microgel particles grow and become densely distributed. Eventually, they are forced to impinge on each other, and the inter-microgel crosslinking becomes predominant, which results in a marked increase in viscosity. The curing process becomes diffusion-controlled and the development of the microgels is restrained, and thus decreasing n0 value [27-28].
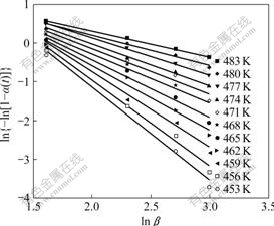
Fig.6 Curves of ln{-ln[1-α(T)]} vs ln β from Ozawa method
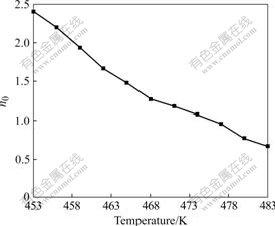
Fig.7 Curve of Ozawa exponent vs temperature
The reaction rate constant obtained by Ozawa theory from anti-logarithmic values of the y-intercept decreases with the increase of temperature up to 483 K (see Table 1). This behavior suggests that the initial curing stage occurs at a higher reaction rate whereas the final stage occurs at a lower reaction rate. As mentioned previously, the microgels grow without restraint at the beginning of the curing process until the impingement reaches the climax, and thereafter the rate constant decreases when the curing reaction becomes diffusion-controlled.
3.4 Thermal degradation behaviors and glass- transition temperature
The thermal degradation behaviors of the copolymers were examined with TG at a heating rate of 15 K/min in
Table 1 Reaction rate constant k0 obtained by Ozawa theory
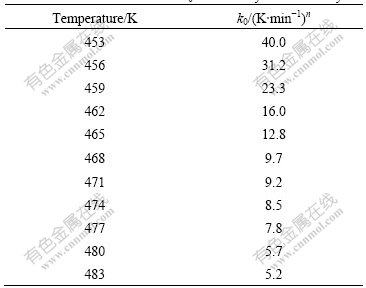
a nitrogen atmosphere. The results are listed in Table 2. From Table 2, it can be confirmed that the thermal stability of the cured copolymers is much better than that of the cured phenolic resin. The char yields indicate that benzoxazine-modified novolac resins have higher char yield, and with the amount of benzoxazine ring growing, the char yield increases. The char yield of copolymer BP31 at 973 K is 50.3 %, which is much higher than that of novolac phenolic resin. This result is as expected for a crosslinked thermoset resin.
The data of derivative thermal gravimetry(DTG) (see Fig.8) clearly show that more than one process occurs during thermal degradation. As the temperature increases, phenolic structure degrades at earlier stage, and benzoxazine structure degrades later. The degradation occurs at a lower rate and in a wider temperature range. This broad range is advantageous for the viewpoint of flame retardance [29].
The crosslinking density of polymers increases with the proceeding of curing reaction. The increase of crosslinking density shortens the average length of chain between the adjacent crosslinks, and increases the glass-transition temperature(Tg). Tg of the crosslinked copolymers with different benzoxazine ring contents is measured with a dynamic DSC scan. The related data are shown in Table 2. Tg increases with the growth of the benzoxazine ring content, suggesting that the higher the content of reactive moieties is, the higher the crosslinking density can be obtained [30]. Tg of pure benzoxazine
Table 2 Thermal stability and Tg of cured benzoxazine-phenolic copolymers and NP

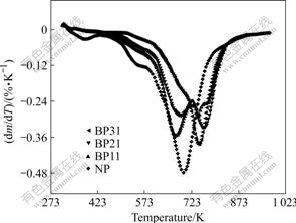
Fig.8 DTG traces of benzoxazine-phenolic copolymers and phenolic resin
resin reported in Ref.[31] is about 446 K, which is much lower than that of copolymers BP31 and BP21, that is to say, with the growth of benzoxazine content, the crosslinking point between phenolic resin and benzoxazine increases, and the crosslinking density is improved effectively. The crosslinking density of pure benzoxazine is too low for wide application, while the combination of benzoxazine and phenolic resin overcomes this shortcoming effectively.
4 Conclusions
(1) Using novolac phenolic resin as phenolic hydroxyl provider to implant the benzoxazine ring into phenolic resin structure, benzoxazine-phenolic copolymer is obtained. The curing temperature of the copolymer is lower than that of pure benzoxazine, and there are no byproducts evolved during the polymerization. The cured copolymers also show good thermal stability and high crosslinking density.
(2) The catalytic effect of phenolic hydroxyl on the benzoxazine ring opening is investigated. The results show that catalytic effect become more significant with the increase of the content of phenolic hydroxyl in the polymer structure. The curing kinetics of copolymer BP31 is investigated, and some kinetic parameters are obtained. The activation energy is 78.8 kJ/mol. The Ozawa exponent n0 decreases from 2.4 to 0.7 when the reaction temperature increases and k0 decreases from 40.0 to 5.2 (K/min)n with temperature up to 483 K, implying the change of the reaction mechanism from microgel growth into diffusion-controlled reaction.
(3) Thermal degradation behaviors and crosslinking properties are studied. Among the tested samples, the highest char yield of copolymers is 50.3%, which suggests that the combination of benzoxazine and phenolic resin enhances the thermal stability of phenolic resin. Tg of the copolymers increases with the increase of benzoxazine content. Tg of copolymer BP31 is 489 K, which is much higher than that of pure benzoxazine resin. This also means that the crosslinking property of the pure benzoxazine resin is improved by the copolymerization of benzoxazine and phenolic resin.
References
[1] ISHIDA H, LOW H Y. A study on the volumetric expansion of benzoxazine-based phenolic resin [J]. Macromolecules, 1997, 30(4): 1099-1106.
[2] KIM H J, BRUNOVSKA Z, ISHIDA H. Synthesis and thermal characterization of polybenzoxazines based on acetylene-functional monomers [J]. Polymer, 1999, 40(23): 6565-6573.
[3] KIM H J, BRUNOVSKA Z, ISHIDA H. Molecular characterization of the polymerization of acetylene-functional benzoxazine resins [J]. Polymer, 1999, 40(7): 1815-1822.
[4] ISHIDA H, ALLEN D J. Physical and mechanical characterization of near-zero shrinkage polybenzoxazines [J]. Journal of Polymer Science (Part B): Polymer Physics, 1996, 34(6): 1019-1030.
[5] CHOI S W, OHBA S, BRUNOVSKA Z, HEMVICHIAN K, ISHIDA H. Synthesis, characterization and thermal degradation of functional benzoxazine monomers and polymers containing phenylphosphine oxide [J]. Polymer Degradation and Stability, 2006, 91(5): 1166-1178.
[6] AGAG T, TAKEICHI T. Synthesis and characterization of novel benzoxazine monomers containing allyl groups and their high performance thermosets [J]. Macromolecules, 2003, 36(16): 6010-6017.
[7] ISHIDA H, OHBA S. Synthesis and characterization of maleimide and norbornene functionalized benzoxazines [J]. Polymer, 2005, 46(15): 5588-5595.
[8] KISKAN B, GACAL B, TASDELEN M A, COLAK D, YAGCI Y. Design and synthesis of thermally curable polymers with benzoxazine functionalities [J]. Macromolecular Symposia, 2006, 245/246(1): 27-33.
[9] LIU Y L, CHOU C I. High performance benzoxazine monomers and polymers containing furan groups [J]. Journal of Polymer Science (Part A): Polymer Chemistry, 2005, 43(21): 5267-5282.
[10] TANG Yan, ZHAO Qing-lai, ZENG Ke, MIAO Pei-kai, ZHOU Ke, TANG Wen-rui, ZHOU Hong-fei, LIU Tao, WANG Yi-peng, YANG Gang. Synthesis of a benzoxazine monomer containing maleimide and allyloxy groups [J]. Chinese Chemical Letters, 2007, 18(8): 973-976. (in Chinese)
[11] LIU Y L, YU J M, CHOU C I. Preparation and properties of novel benzoxazine and polybenzoxazine with maleimide groups [J]. Journal of Polymer Science (Part A): Polymer Chemistry, 2004, 42(23): 5954-5963.
[12] MEN W W, LU Z J, ZHAN Z R. Synthesis of a novel benzoxazine precursor containing phenol hydroxyl groups and its polymer [J]. Journal of Applied Polymer Science, 2008, 109(4): 2219-2223.
[13] ANDREU R, REINA J A, RONDA J C. Carboxylic acid-containing benzoxazines as efficient catalysts in the thermal polymerization of benzoxazines [J]. Journal of Polymer Science(Part A): Polymer Chemistry, 2008, 46(8): 6091-6101.
[14] DUNKERS J, ISHIDA H. Reaction of benzoxazine-based phenolic resins with strong and weak carboxylic acids and phenols as catalysts [J]. Journal of Polymer Science (Part A): Polymer Chemistry, 1999, 37(13): 1913-1921.
[15] ANDREU R, REINA J A, ROND J C. Studies on the thermal polymerization of substituted benzoxazine monomers: Electronic effects [J]. Journal of Polymer Science (Part A): Polymer Chemistry, 2008, 46(10): 3353-3366.
[16] RO?U D, MITITELU A, CA?CAVAL C N. Cure kinetics of a liquid-crystalline epoxy resin studied by non-isothermal data [J]. Polymer Testing, 2004, 23(2): 209-215.
[17] RO?U D, CA?CAVAL C N, MUSTATA F, CIOBANU C. Cure kinetics of epoxy resins studied by non-isothermal DSC data [J]. Thermochimica Acta, 2002, 383(1/2): 119-127.
[18] RIMDUSIT S, ISHIDA H. Gelation study of high processability and high reliability ternary systems based on benzoxazine, epoxy, and phenolic resins for an application as electronic packaging materials [J]. Rheologica Acta, 2002, 41(1/2): 1435-1528.
[19] TAKEICHI T, KANO T, AGAG T. Synthesis and thermal cure of high molecular weight polybenzoxazine precursors and the properties of the thermosets [J]. Polymer, 2005, 46(26): 12172-12180.
[20] RAO B S, REDDY K R, PATHAK S K, PASALA A R. Benzoxazine–epoxy copolymers: Effect of molecular weight and crosslinking on thermal and viscoelastic properties [J]. Polymer International, 2005, 524(10): 1371-1376.
[21] PARK B D, RIEDL B, HSU E W, SHIELDS J. Differential scanning calorimetry of phenol-formaldehyde resins cure-accelerated by carbonates [J]. Polymer, 1999, 40(7): 1689-1699.
[22] ISHIDA H, RODRIGUEZ Y. Curing kinetics of a new benzoxazine-based phenollic resin by differential scanning calorimetry [J]. Polymer, 1995, 36(16): 3151-3158.
[23] POLLARD M, KARDOS J L. Analysis of epoxy resin curing kinetics using the Avrami theory of phase change [J]. Polymer Engineering and Science, 1987, 27(11): 829-834.
[24] LU M G, SHIM M J, KIM S W. The macrokinetic model of thermosetting polymers by phase-change theory [J]. Materials Chemistry and Physics, 1998, 56(2): 193-197.
[25] OZAWA T. Kinetic analysis of derivative curves in thermal analysis [J]. Journal of Thermal Analysis and Calorimetry, 1970, 2(3): 301-324.
[26] OZAWA T. Thermal analysis—Review and prospect [J]. Thermochimica Acta, 2000, 355(1/2): 35-42.
[27] LU M G, SHIM M J, KIM S W. Curing behavior of an unsaturated polyester system analyzed by Avrami equation [J]. Thermochimica Acta, 1998, 323(1/2): 37-42.
[28] LI Zhi-hua, ZHENG Zi-qiao, REN Dong-yan, HUANG Yao-peng. Curing mechanism of TDE-85MeTHPA epoxy resin modified by polyurethane [J]. Journal of Central South University of Technology, 2007, 14(3): 296-300.
[29] ZUO Jian-dong, LI Rong-xun, FENG Shao-hua, LIU Guang-ye, ZHAO Jian-qing. Flame retardancy and its mechanism of polymers flame retarded by DBDPE/Sb2O3 [J]. Journal of Central South University of Technology, 2008, 15(1): 64-68.
[30] PAN Chun-yue, GAO Jin-huan, ZHANG Qian, FENG Qing, CHAO meng. Preparation and properties of PEO/LiClO4/KH560-SiO2 composite polymer electrolyte by sol-gel composite-in-situ method [J]. Journal of Central South University of Technology, 2008, 15(3): 295-300.
[31] SU Y C, YEI D R, CHANG F C. The kinetics of b-a and p-a type copolybenzoxazine via the ring opening process[J]. Journal of Applied Science, 2005, 95(3): 730-737.
(Edited by CHEN Wei-ping)
Foundation item: Project (20050106) supported by the Key Science and Technology Item of Guangdong Province, China
Received date: 2008-10-30; Accepted date: 2008-12-05
Corresponding author: XU Li, PhD; Tel: +86-13570592575; E-mail: xuliy@yahoo.cn