
Wear mechanism and serious wear position of casing pipe in vertical backfill drill-hole
ZHANG Qin-li1, CUI Ji-qiang1, 2, ZHENG Jing-jing1, WANG Xin-min1, WANG Xian-lai1, 2
1. School of Resources and Safety Engineering, Central South University, Changsha 410083, China;
2. Jinchuan Nonferrous Metal Corporation, Jinchang 737100, China
Received 23 June 2011; accepted 1 August 2011
Abstract: Vertical backfill drill-hole is usually a key project in an underground mine with backfill method and can be easily damaged by impact of backfill slurry. Observation of the damaged vertical backfill drill-holes in Jinchuan Nonferrous Metal Corporation (JNMC), Gansu Province, China, given by a digital drill-hole video camera, indicated that there usually exist serious wear zones in casing pipe in vertical backfill drill-hole (CVBH). It was suggested that serious wear position of CVBH should be located at an interface between air and solid-liquid mixture within CVBH. Backfill slurry falls freely and impacts the wall of CVBH near the interface with great momentum and energy coming from high speed free fall of backfill slurry. The depth of serious wear position of CVBH, i.e., free fall height of backfill slurry in CVBH, can be estimated by the height of vertical backfill drill-hole, the length of horizontal pipeline, the density of slurry and the hydraulic gradient of pipeline system. A case study indicated that the estimation equation of serious damage depth of CVBH was of enough accuracy and was helpful for daily maintenance and management of vertical backfill drill-hole.
Key words: vertical backfill drill-hole; backfill pipeline; impact; wear mechanism; serious wear position
1 Introduction
Backfill method is one of the most important mining techniques in underground mines due to high recovery rate of mineral resources and qualified safety control [1]. Binding material, aggregate and water are mixed and usually transported through casing pipe in vertical backfill drill-hole (shortly, CVBH) and horizontal or inclined pipeline into stope by gravity flow. Pressure from slurry weight in CVBH is the reason of slurry movement in this transportation system [2-4]. Vertical backfill drill-hole, obviously, plays the most important role in the transportation of backfill slurry from surface to underground stope [5-6], and is easily damaged by erosion and abrasion of the slurry. Mining cycles may be disrupted once CVBH is broken.
It is very common for inspection and repair of pipeline in municipal transportation system of natural gas, oil and water. The most common method is to reveal broken pipeline and then to carry out inspection and repair process [7]. Repair techniques include welding, sealing, pressure reducing, partial replacement, paint spraying and tube lining [8-11]. Inspection and maintenance of damaged CVBH are, however, more difficult because the damage condition of CVBH is influenced by many factors and cannot be easily observed. It is known that the influencing factors on the abrasion of CVBH include features of backfill slurry, drilling quality of the hole, size, materials and installation quality of pipeline in the hole, and ratio of total length to vertical height of pipeline [12-13], but the abrasion mechanism of CVBH is still not clear because many influencing factors can not be quantitatively determined. Wear types of CVBH are clarified in this work, based on the investigation of damaged CVBH in Jinchuan Nonferrous Metal Corporation (JNMC), Gansu Province, China, and on the analysis of flow regime of backfill slurry in CVBH. The physical transportation model of backfill slurry is set up and the wear mechanism of backfill pipeline in drill-hole is discussed based on the change of backfill slurry’s momentum and energy. In addition, an estimation approach of the most serious wear position of backfill hole is suggested.
2 Flow regime of backfill slurry and wear pattern of CVBH
2.1 Flow regime of backfill slurry
Static head of backfill slurry in vertical hole is the motive power of slurry flow in gravity transportation system. Assuming that the inner diameter of backfill hole is fixed and that the vertical height of the hole, the length of horizontal pipeline, the density of slurry, the hydraulic gradient of transportation system are respectively H, L, γj and i, the backfill slurry is transported at one of the following three patterns:
1) Static head is less than the resistance to flow of backfill slurry and slurry cannot be transported while γjH
2) Static head is equal to the resistance to flow of backfill slurry and slurry fills the total vertical pipe while γjH=i(H+L);
3) Static head is greater than resistance to flow of backfill slurry and slurry freely falls in upper part of backfill hole while γjH>i(H+L).
It is a common case in backfill system that backfill slurry in vertical hole initially falls to interface between air in upper part of the hole (H1) and solid-liquid mixture in lower part of the hole (H2), as shown in Fig. 1 [14]. In other words, backfill slurry in vertical hole usually flows in two regimes, free fall movement and smooth flow movement.
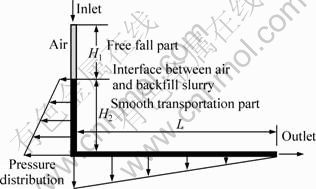
Fig. 1 Common sketch of backfill transportation system
2.2 Wear pattern of CVBH
Backfill drill-holes in Loushou Mine of JNMC were detected by using digital drill-hole video camera, and it was found that there usually exists a special range where CVBH is seriously damaged as shown in Table 1. For example, serious wear depths of holes No.W2 and No.E5 in JNMC are 27-31 m and 10-15 m below the surface, respectively. Partially serious wear is caused by impacting of backfill slurry in free fall part of CVBH to partial pipe wall at a high speed. Wear of pipe wall is greatly lessened in smooth transportation part of CVBH because slurry flows at a stable velocity in this area.
Table 1 Serious wear depth of damaged backfill holes in JNMC given by digital drill-hole video camera
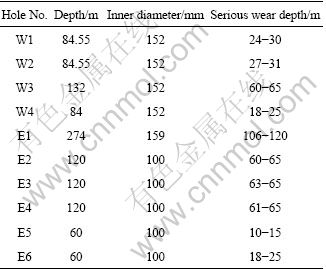
3 Wear mechanism of CVBH
The analysis mentioned above suggests that impact of backfill slurry in free fall part of CVBH is the main reason of CVBH damage. The wear mechanism of CVBH can be clarified by changes of backfill slurry’s momentum and energy when it flows in CVBH (Fig. 2).
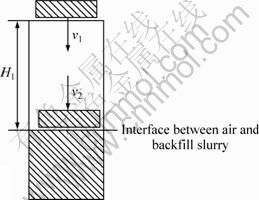
Fig. 2 Impact wear model of backfill slurry in CVBH
3.1 Wear caused by backfill slurry’s momentum
The movement of backfill slurry in free fall part of CVBH can be approximately regarded as uniformly accelerated linear motion. According to the characteristics of the motion, the following equations can be educed:
(1)
(2)
where H1 is the vertical height of the free fall part; v1 is the initial velocity of backfill slurry in unit mass when it enters into the pipeline; v2 and t are the velocity of the backfill slurry and time when it arrives at interface between air and backfill slurry; and g is the gravity acceleration. Then, v2 can be calculated from Eqs. (1) and (2) as follows:
(3)
where c is a speed correction coefficient depending on diameter and roughness of the pipe and some other factors. It is usually determined by experiment, varying form 0.7 to 0.8. It can be seen from Eq. (3) that v2 increases with increasing H1.
The faster the speed of backfill slurry is, the greater the momentum is. The momentum of backfill slurry reaches a maximum when it drops to the interface between air and backfill slurry, and then reduces almost to zero in a short time because of the collision at the interface. The rapid decrease of momentum produces a huge impact. Assuming that the density of backfill slurry is ρ, the sectional area of pipe is A and the impact time is Δt, the total mass of backfill slurry (Δm) impacting on the interface can be presented as:
(4)
and the momentum change (ΔP) of backfill slurry is equal to

The impact (F) of slurry to the interface defined as momentum change in unit time can be calculated as:
(5)
Combining Eqs. (4) and (5), F can be shown as:
(6)
where a is a correction coefficient, here a=0.7-0.8. Eq. (6) shows that impact F has direct proportion relation to the square of v2. It can be known that the faster the terminal speed of slurry is, the more serious the impact wear of the pipeline would be. It is necessary, therefore, to reduce the height of free fall part (H1) in order to lessen wear degree of CVBH.
3.2 Wear caused by backfill slurry’s energy
The total energy (E) needed when backfill slurry moves from the pipeline inlet to the interface between air and backfill slurry is the sum of initial kinetic energy of backfill slurry and the work done by gravity. Assuming that the unit mass of backfill slurry entering into pipeline in the unit time (Δt) is Δm, the total energy E can be computed as:
(7)
Substituting
into Eq. (7) , Eq. (7) can be rewritten as:
(8)
where Q is the flux of backfill slurry. The energy (E) of backfill slurry mainly acts on the wall of the pipeline. That is to say, backfill slurry consumes the energy in the process of friction to the pipeline wall. Energy acted on unit surface area of pipe wall (Ew), therefore, can reflect the degree of the pipeline’s abrasion. Great Ew means serious wear of CVBH. Ew can be calculated from Eq. (8) as:
(9)
where S denotes the effective abrasion area that slurry contacts with the wall of pipeline. The smaller the effective abrasion area that unit mass slurry contacts the pipeline wall, the more serious the abrasion of the pipeline would be because of the increase of the energy consumption on the unit area. The relation between the deflection rate of drill-hole and the wear of CVBH can be well explained by Ew. For example, Ew is large and wear is serious for CVBH because of lower effective abrasion area while the drill-hole is greatly inclined.
4 Estimation of serious wear position of CVBH
The flow regime of backfill slurry and wear pattern of CVBH suggest that the interface between air and backfill slurry in vertical backfill drill-hole is the weakest area where pipe wall is impacted by backfill slurry at a high speed. Therefore, serious wear position of CVBH can be estimated by the computation of interface depth. Interface depth, H2 in Fig. 1, can be calculated according to the following equation:
(10)
where
is the density of backfill slurry; i1 and i2 are the hydraulic gradient of backfill slurry in both vertical and horizontal pipelines; L is the length of horizontal pipe. The depth of easy damaged position, H1, can be estimated from Eq. (10) as follows:
(11)
where H denotes the height of vertical backfill drill-hole. The hydraulic gradient of backfill slurry in both vertical and horizontal pipelines are usually only unknown variables in Eq. (11). The hydraulic gradient of backfill slurry relates to many factors [15-16] and can be calculated by [17]:
(12)
where Cv is the volume fraction of backfill slurry; D is the inner diameter of pipe; i0 is the hydraulic gradient of fresh water; Cx is the settlement resistance factor of solid particles; v is the speed of backfill slurry. The speed of backfill slurry can be computed according to the flux of backfill slurry (Q) and the inner diameter of pipe (D) as follows:
(13)
The following equation can be used to approximate i0:
(14)
where λ is the friction resistance factor estimated as:
(15)
where K1 and K2 are the installation and joint quality factors of the pipeline, respectively, each taken as 1.1 here.
The settlement resistance factor of solid particles (Cx) can be calculated as:
(16)
where dcp is the average size of the mixture; ω is the average settlement rate of solid particles which can be calculated according to the temporary parameter
.
When dcp<0.3A,
(17)
when 0.3A≤dcp
(18)
when A≤dcp<4.5A,
(19)
and when dcp≥4.5A,
(20)
5 Case study
JNMC, a worldwide famous nickel-copper producer in China, uses downward cut and fill method to extract its weak deposit. Backfill slurry formed by water and a mixture of portland cement and milled gobi sand with mass ratio of 1:4 is transported into underground stope by gravity through a combination of vertical backfill hole and horizontal pipeline. The designed flow capacity is 100 m3/h. The volume fraction (Cv) and density (γj) of backfill slurry are 56.6% and 1.98 t/m3, respectively. The average size of the mixture (dcp) of cement and gobi sand is 0.079 cm. Since
dcp=0.079 is greater than A but less than 4.5A. The average settlement rate of solid particles (ω) can be estimated by Eq. (19) as follows:
cm/s, and consequently Cx=1.582 can be calculated from Eq. (16). Substituting the parameters mentioned above, the hydraulic gradients of pipes with different diameters can be given by Eq. (12). Serious wear depths (H1) of damaged CVBH in JNMC mentioned in Table 1 can be estimated easily by Eq. (11) as shown in Table 2, because the height of backfill hole, the length of horizontal pipeline, the density of slurry and the hydraulic gradients of both vertical and horizontal pipelines have been known. It can be found that the estimated serious wear depths of damaged CVBH are similar with the survey results by digital drill-hole video camera, based on the comparison of Tables 1 and 2.
Table 2 Estimated wear depth of damaged CVBH in JNMC
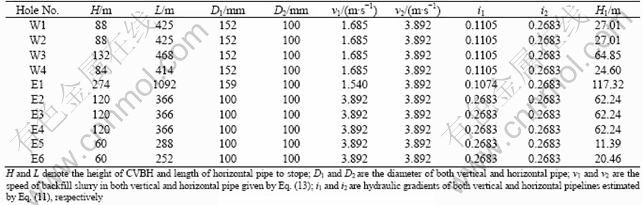
6 Conclusions
1) Observation of digital drill-hole video camera indicated that the wear condition of casing pipe of vertical backfill drill-hole (CVBH) was not the same along whole depth direction. There usually existed a serious wear zone for each CVBH.
2) It is a common case that backfill slurry falls freely in CVBH until it reaches an interface between air in upper part of the hole and solid-liquid mixture in lower part of the hole, and then flows smoothly. Impact from free fall of backfill slurry is the primary damage pattern of CVBH.
3) The terminal speed that backfill slurry arrives at the interface increases with increasing the vertical height of free fall part in CVBH. The wear degree of CVBH depends on momentum and energy of backfill slurry in CVBH which have close relation to the terminal speed of backfill slurry. Generally speaking, the faster the backfill slurry falls in CVBH is, the greater the momentum and energy consumption acting on the wall of CVBH are and the more serious the damage of CVBH would be.
4) Serious wear position of CVBH lies in interface between air and backfill slurry. Serious wear depth of CVBH can be estimated by energy balance principle. Preventive measures can be, therefore, taken in order to avoid serious local wear of vertical backfill hole and to prolong backfill hole’s service life.
References
[1] PENG Xin, LI Xi-bing, ZHANG Qin-li, WANG Xin-min. Quality evaluation of layerlike backfilling and flow pattern of backfill slurry in stope [J]. Journal of Central South University of Technology, 2007, 14(4): 580-583.
[2] WANG Pei-xun. Hydraulic gradient calculation of mine filling paste [J]. Nonferrous Mines, 2003, 32(1): 8-11. (in Chinese)
[3] ZHANG Qin-li, WANG Xin-min. Performance of cemented coal gangue backfill [J]. Journal of Central South University of Technology, 2007, 14(2): 216-219.
[4] LIL, USUIH, SUZUKIH. Study of pipeline transportation of dense fly ash-water slurry [J]. Coal Preparation, 2002, 22(2): 65-80.
[5] ZHENG Jing-jing, ZHANG Qin-li, WANG Xin-min, WANG Xian-lai. The prediction of backfilling drill-hole’s life based on BP neutral networks [J]. Joumal of Xiangtan Normal University: Natural Science Edition, 2008, 30(4): 40-44. (in Chinese)
[6] LI Yao-wu, WANG Xin-min, ZHAO Bin, ZHANG Qin-li. Analysis of abrasion factors of drill holes for filling [J]. Metal Mine, 2008(6): 27-30. (in Chinese)
[7] McNEILL D, SCOTT B, McCOY J, THIESON D. An overview of Enbridge’s pipeline repair program [C]//Proceedings of the International Pipeline Conference. Alta, IPC, American Society of Mechanical Engineers, v B, 2002: 1903-1911.
[8] HRRIS I D. Internal repair of gas pipelines survey of operator experience and industry needs report [R]. Department of Energy, Washington, D.C. Sep, 2003: 64.
[9] PHILLIPS D, FEARON P. Similkameen river pipeline crossing the repair process [C]//Proceedings of the International Pipeline Conference. Alta, IPC, American Society of Mechanical Engineers, v B, 2002: 1363-1369.
[10] CHABURKIN V F, IVANOV A A, STEKLOV O I. Repairability and repair technologies to remedy trunk pipeline SCC damages [J]. Welding in the World, 2007, 51: 733-740.
[11] LAZZARIN D, COLOMBO A. ARCOS pipeline repair system [C]//Proceedings of the International Offshore and Polar Engineering Conference. Stavanger: International Society of Offshore and Polar Engineers, v 1, 2001: 27-31.
[12] STEWARD N R, SPEARING A J S. Wear of backfill pipelines in South African gold mines [C]//Simultaneous Convening of the 4th International Conference on Bulk Materials Storage, Handling and Transportation and the 7th International Symposium on Freight Pipelines. National Conference Publication- Institution of Engineers, Australia: IE Aust, 1992: 115-121.
[13] Steward N R, Spearing A J S. Effect of particle sharpness of the wear of backfill pipelines [J]. Journal of the South African Institute of Mining and Metallurgy, 1993, 93(5): 129-134.
[14] WANG Xin-min, XIAO Wei-guo, ZHANG Qin-li. Deep mining backfill theory and technology [M]. Changsha: Central South University Press, 2005. (in Chinese)
[15] NI F, ZHAO L, MATOUSEK V. Two-phase flow of highly concentrated slurry in a pipeline [J]. Journal of Hydraulics: Series B, 2004, 16(3): 325-331.
[16] TADASHI M. Hydraulic flow tests of APWR reactor internals for safety analysis [J]. Nuclear Engineering and Design, 2008, 238(3): 469-481.
[17] ZHANG Qin-li, HU Guan-yu, WANG Xin-min. Hydraulic calculation of gravity transportation pipeline system for backfill slurry [J]. Journal of Central South University of Technology, 2008, 15(5): 645-649.
充填钻孔内管道磨损机理及易破损位置的确定
张钦礼1,崔继强1, 2,郑晶晶1,王新民1,王贤来1, 2
1. 中南大学 资源与安全工程学院,长沙 410083;
2. 金川集团有限公司,金昌 737100
摘 要:充填钻孔是充填法矿山的关键工程之一,容易受到充填料浆的冲击而造成破损。金川公司数字化钻孔电视观测结果表明,充填钻孔存在一个严重破损区域,该区域位于充填钻孔内空气和料浆的接触面上。充填料浆自由降落,具有较高的末期下落速度,而以较高的动量和冲量冲击充填钻孔的内套管管壁。因此,充填钻孔的严重磨损深度,即空气与料浆的接触面高度,可以根据充填钻孔的高度、水平管道的长度、浆体的密度和水力坡度进行估算。实例研究结果表明,该估算方法具有足够的精度,有利于充填钻孔的日常管理和维护。
关键词:充填钻孔;充填管道;冲击;磨损机理;易破损位置
(Edited by YUAN Sai-qian)
Foundation item: Project (2008BAB32B03) supported by the National Key Technology Research and Development Program, China
Corresponding author: ZHANG Qin-li; Tel: +86-731-88879612; E-mail: zhangqinlicn@126.com
DOI: 10.1016/S1003-6326(11)61042-X