
Electrochemical behavior of dental implant system before and after clinical use
Chae-Heon CHUNG1, Hee-Jung KIM1, Yong-Tae JEONG1, Mee-Kyoung SON1,
Yong-Hoon JEONG2, Han-Cheol CHOE2
1. Department of Prosthodontics, College of Dentistry, Chosun University, Gwangju, 520-825, Korea;
2. Department of Dental Materials & Research Center of Nano-Interface Activation for Biomaterials,
College of Dentistry, Chosun University, Gwangju, 520-825, Korea
Received 18 June 2008; accepted 10 March 2009
Abstract: Electrochemical behavior of dental implant system before and after clinical use (in vivo and in vitro) was researched by using abutment and titanium fixture. To simulate an oral environment, the samples of clinically used and non-used implant systems as a working electrode were exposed to artificial saliva at (36.5±1) ℃. Electrochemical tests were carried out using a potentiostat. After electrochemical test, the corrosion morphology of each sample was investigated by FE-SEM and EDS. The corrosion potential and pitting potential of clinically used implant system are lower than those of non-used implant system, and clinically used implant system exhibits a lower range of passivation, indicating a less degree of inherent resistance against chloride ion. The polarization resistance decreases in the case of clinically used implant system, whereas, Rp for clinically non-used implant system increases compared with clinically used implant system.
Key words: dental implants; electrochemical test; titanium fixture; pitting and crevice corrosion
1 Introduction
One of the most important problems caused by the defects in the components of implants and their manufacturing process, is corrosion. Corrosion can be defined as the destruction of a certain substance, especially metal, in reaction with the environment[1]. The issue of corrosion has begun to attract attention since the presentation of a scientific research about the galvanic corrosion of dental compound about 40 years before[2], and corrosion due to the classical combination of amalgam and gold was mentioned in most of the early researches. For the corrosion of implants, researches on the galvanic corrosion due to the different metal between the fixture and the upper structure have been actively conducted[3], and recently, research reports were made about the galvanic corrosion due to the materials of the abutment and the upper structure[4].
However, there were few researches conducted about the surface phenomena or stability in the failed implant system after clinical use.
Therefore, in this study, we carried out corrosion test for the implant system in artificial saliva to observe the electrochemical behavior of dental implant system before and after clinical use, and observed the phenomena on the surface as well as inside the implant.
2 Experimental
To evaluate the stability of the implant system before and after clinical use, the potentiodynamic test and AC impedance test were conducted using an electrochemical method (Model PARSTAT 2273, EG&G, Co., USA) in artificial saliva electrolyte of (36.5 ±1) ℃.
To observe the general corrosion characteristics of the specimen, the potentiodynamic tests were carried out in the artificial saliva electrolyte with scanning rate of 1.66 mV/s. The surface of the tested specimen was stabilized by applied potential of -1 500 mV for 10 min in de-aerated solution. The foreign matter, oxide and dissolved oxygen on the surface of the test specimen were removed, and the density, passive current density and φpit of each test specimen were measured under the same conditions. The voltage from -1 500 to 2 000 mV was applied to specimen for polarization test.
The AC impedance test was carried out in the artificial saliva electrolyte of (36.5±1) ℃ using frequency response detector (Model 1025, EG&G, USA). The range of frequencies used for the measurement was from 10 mHz (low) to 100 kHz (high). Nyquist plot, Bode plot and Bode-phase plot were obtained, and the polarization resistance(Rp) value and the value of solution resistance(RΩ) were analyzed for metal ion released from the implant system before and after clinical use.
3 Result and discussion
Fig.1 shows SEM images and EDS of the implant that has not been clinically used. The cast defects or defects due to sand blasting were observed in the upper structure, and precious metal elements such as Au and Ag were found as a result of EDS. A cleavage can be found at the abutment of the implant fixture. The EDS analysis resulted in findings of Ti, the ingredient of the fixture. Mechanical processing defects were observed on the surface of the fixture, and only Ti was found in the EDS analysis.
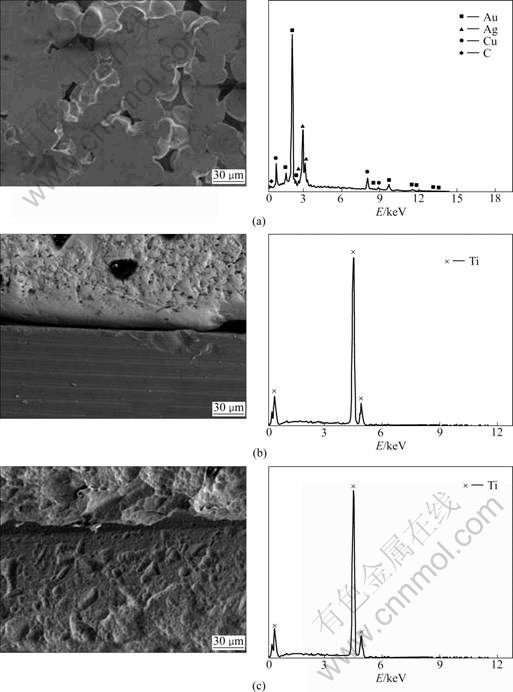
Fig.1 SEM images and EDS showing surface morphology of non-clinically used implant: (a) Abutment; (b) Abutment/fixture;
(c) Fixture
Fig.2 shows SEM images and EDS of the surface of the clinically used implant. Precious metal elements such as Au and Ag were also found in the clinically used implant, but Na and Cl were found as shown in Fig.2(a), revealing severe corrosion in the cleavages. In Fig.2(c), only Ti, the ingredient of the implant fixture, was found. It can be seen that debris were attached to the upper structure as shown in Figs.2(a), (b) and (c), as well as the abutment and the surface of the fixture.
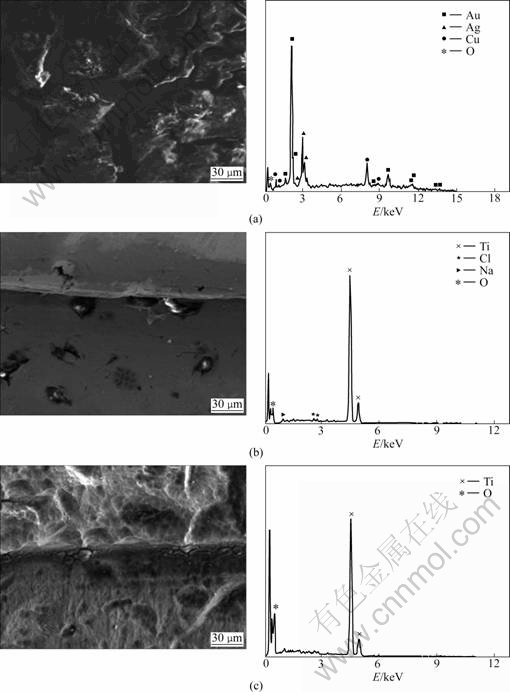
Fig.2 SEM images and EDS showing surface morphology of clinically used implant: (a) Abutment; (b) Abutment/fixture; (c) Fixture
Fig.3 shows the anodic polarization curves of implants before and after clinical use in artificial saliva at (36.5±1) ℃. In evaluating the stability only with φcorr, it can be thought that the release of metal ions easily occurs in the mouth if φcorr is low. φcorr of the implant system before clinical use was -300 mV, and that of the implant after clinical use was -750 mV, showing that the stability of the unused implant system is higher. The potential under which a passive coating is generated on the Ti compound and destroyed is φpit, and the stability rises in proportion to the φpit. The evaluated φpit was about 1 000 mV in the implant before clinical use and 100 mV after clinical use (which is very low), showing that the pit resistance is very low. Especially, in implant systems connected with screws, crevice corrosion easily occurs. The evaluation of the crevice corrosion is indicated by the absolute value, |φpit-φcorr| [5]. It can be concluded that if the value is big, cleavage corrosion seldom occurs, but if the value is small, occurrence is frequent. Based on this observation, the |φpit-φcorr| of the implant before clinical use was 1300 mV and that of the used implant was 850 mV, showing that the resistance of the cleavage corrosion is very low. This appears because cleavage corrosion is active in progress inside. Also, stability can be evaluated depending on how easily the passive coating is generated. Since stability increases with the decease of current density, the current density was 4.0×10-5 A/cm2 in unused implants, which was about 1 order lower than that of the used implant (1.5×10-4 A/cm2), showing superior stability. It can be deduced through the potentiodynamic test that used implant systems have much corrosion products inside, and that the metal ion at the upper part is eluded.
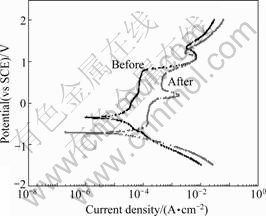
Fig.3 Anodic polarization curves of implant before and after clinical use during potentiodynamic test in artificial saliva solution at (36.5±1) ℃
For the precise evaluation, we conducted the AC impedance test[6] instead of DC, and the result is shown in the Nyquist plot (Fig.4). It can be seen that the AC impedance increases in proportion to the trace of the semicircle. The trace of the unused implant increases more than that of the used one[6], demonstrating more stability. The polarization resistance, Rp, that enables the evaluation of the corrosion resistance of the implant system, can be measured on the Nyquist plot. However, the method of calculating the difference of the impedance in the low and high frequency area is more commonly used[7] due to the diffusion in the low frequency area. In finding the Rp value on the Bode plot (Fig.5), the RΩ, indicating the solution resistance, is about 10 Ω?cm2 at the intersection on the right before and after clinical use, while the RΩ+Rp value is 104 Ω?cm2 and 103 Ω?cm2 each before and after clinical use. Therefore, it can be seen that the stability of the implant system is good. In other words, the Bode plot shows a similar tendency of being characterized into two sectors in all test specimens as shown in Fig.5. First, as for the Bode plot in the high frequency area, the value of lg|Z| according to lg f was consistent as the phase angle got closer to zero. This area is the solution resistance(RΩ), which is the resistance of the electrolyte. The lg|Z| value in the frequency area where the phase angle is close to -90? means the sum of the polarization resistance(Rp) and the solution resistance(RΩ)[8-9]. Also, in the unused test specimen, the value of lg|Z| according to lg f in mid/low frequency areas was found to have an inclination close to -1. This indicates the capacitance(Cp) [8-9] of the elaborate passive coating due to Ti in the pure capacity area. Generally, the resistance of the compound to the electrolyte is seldom influenced. Therefore, the polarization resistance is most important in the implant system. Naturally, it can be seen that used implant systems cannot form a stable coating on the surface.
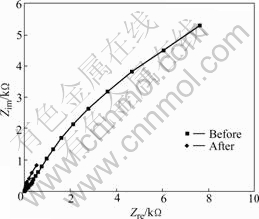
Fig.4 Nyquist plot of implant before and after clinical use during AC impedance test in artificial saliva solution at (36.5±1) ℃
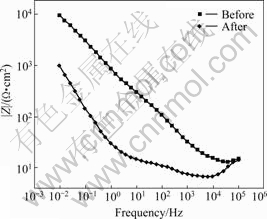
Fig.5 Bode plot of implant before and after clinical use during AC impedance test in artificial saliva solution at (36.5±1) ℃
The stability of the implant can be found through the phase—frequency plot. It could be seen from Fig.6 that the phase angle in all the implant systems dropped towards 0? in the high frequency area, and rose again. On the contrary, in the mid and low frequency area, the phase angle rose again in the clinically unused test specimen. This appears because the impedance is influenced by the resistance of the surface film. The phase angle is constant, close to -90? in a wide frequency area. This phenomena means that a passive coating[8-9] is formed on the surface. Therefore, it appears that as the phase angle area close to -90? became wider, a very stable passive coating is formed on the surface of the compound, greatly improving the stability[10]. Therefore, upon observing the above figure based on this interpretation, it can be seen that the section of the passive coating is the smallest in the clinically used implant. And, as for the unused implant, it can be seen that the section of the passive coating increases, which means the stability has been improved.
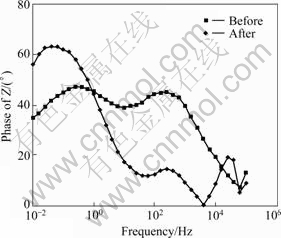
Fig.6 Bode-phase plot of implant before and after clinical use during AC impedance test in artificial saliva solution at (36.5±1) ℃
Fig.7 shows the corrosion surface morphologies of implants before and after clinical use. The EDS results on the surface after conducting the corrosion test to the implants before and after clinical use at the upper structure, show that many pits were generated. At the corrosion surface, the Cl- ion content is 4.60% (mass fraction), Ti 79.69%, and Ag 15.73%. Especially, in the middle part, Cl- ion content is 19.08%, Ti 22.32%, Ag 46.36%, and Au 12.24%, where the abutment and the fixture meet, and the amount of Cl- ions on the surface increases. In below part, Cl- ion content is 6.68%, Ti 72.57%, and Ag 20.75% as a result of EDS analysis of the surface. And Cl- ions and Ag are also found at the fixture shown below. After conducting the potentiodynamic test with the clinically used and unused implants, the surfaces were observed by FE-SEM and EDS. Corrosion occurred in unused implants along the dendritic structure at the abutment, which is the cast structure, and Cl ions in the artificial saliva solution with Au, Ag (compound elements) were found from EDS analysis, which shows that severe corrosion occurred along the cast structure. Also, cleavage corrosion was severe between the abutment and the fixture. This shows that the crevice acted as the nest of the corrosion, accelerating the corrosion with the invasion of the Cl- ion[9]. Especially, corrosion occurs at the groove part of the fixture screw, and is even more severe where there are defects due to mechanical processing.
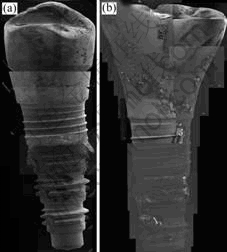
Fig.7 SEM images of implants after potentiodynamic corrosion test: (a) Before clinical use; (b) After clinical use
In the clinically used implant (Fig.7(b)), many pits can be seen in the upper structure; erosions are seen where there are pits made during the casting, or where there are foreign matters. Especially, Cl- ion content was found to be 4.60% at the corrosion surface, proving that corrosion was severe. Most importantly, the Cl- ion content was 19.08% in the cleavage, proving that corrosion was very severe. There is a high possibility that the Cl- ion, which invaded through this cleavage, had accelerated the internal corrosion. Also, the Cl- ion content was 6.68% and the Ag 20.75% on the corrosion surface of the fixture, showing that the eluded ion dripped on the lower fixture surface, causing severe corrosion on the upper prosthetic materials.
4 Conclusions
The result of the potentiodynamic test conducted with the clinically used and unused implants showed that the φpit of the implant system was about 1 000 mV and 100 mV in unused and used implants, and the φcorr was -300 mV and -750 mV, respectively. The value of |φpit-φcorr|, which is the resistance of the crevice corrosion, was 1 300 mV and 850 mV, respectively. The result of the AC impedance test conducted to clinically unused and used implants showed that the Rp was 9.27×103 Ω?cm2 and 6.6×102 Ω?cm2, respectively, in the unused and used implants. After corrosion, the investigation on the internal surface of the implant systems revealed that the internal fixture, screw thread and groove parts were corroded in both implants, as well as the crevice between the upper structure/fixture.
References
[1] HHERBERT H U, REVIE R W. Corrosion and corrosion control [M]. New York: Jhon Willey & Sons, 1985: 1.
[2] GEIS-GESTORFER J, WEBER H. Corrosion resistance of the implant materials Contimet 35, Memory, and Vitallium in artificial physiological fluids [J]. Int J Oral Maxillofac Implants, 1988, 3: 135-140.
[3] GEIS-GESTORFER J, WEBER H. In vitro substance loss due to galvanic corrosion in Ti implant/Ni-Cr supraconstruction systems [J]. Int J Oral Maxillofac Implants, 1989, 4: 119-123.
[4] GEIS-GESTORFER J. In vitro corrosion measurements of dental alloys [J]. Int J of Dentistry, 1994, 22: 247-251.
[5] SON M K, CHOE H C, CHUNG C H. Corrosion behavior between dental implant abutment and cast gold alloy [J]. Metals and Materials International, 2004, 10: 153-159.
[6] JHONES D A. Principles and prevention of corrosion [M]. Maxwell Macmillan International Editions, 1992: 243-285.
[7] IBRIS N, ROSCA J C M. EIS study of Ti and its alloys in biological media [J]. Journal of Electroanalytical Chemistry, 2002, 526: 53-62.
[8] SHUKLA A K, BALASUBRAMANIAM R, BHARGAVA S. Properties of passive film on CP titanium, Ti-6Al-4V and Ti-13.4Al-29Nb alloys insimulated human body conditions [J]. Intermetallics, 2005, 13: 631-637.
[9] CHOE H C, HONG K S, KO Y M, KIM B O. Surface analysis of clinically failed dental implant fixture [J]. J Kor Inst Met & Mater, 2006, 44: 400-411.
[10] GONZALEZ J E G, MIRZA-ROSCA J C. Study of the corrosion behaviorof titanium and some of its alloys for biomedical and dental implant applications [J]. Journal of Electroanalytical Chemistry, 1999, 471: 109-115.
Corresponding author: Han-Cheol CHOE; Tel: +82-62-230-6896; Fax: +82-62-230-6896; E-mail: hcchoe@chosun.ac.kr
DOI: 10.1016/S1003-6326(08)60362-3
(Edited by YUAN Sai-qian)