
Behavior of silver and lead in selective chlorination leaching process of gold-antimony alloy
LIU Wei-feng(刘伟锋), YANG Tian-zu(杨天足), XIA Xing(夏 星)
School of Metallurgical Science and Engineering, Central South University, Changsha 410083, China
Received 29 December 2008; accepted 23 March 2009
Abstract: The behavior of silver and lead in the selective chlorination leaching process of gold-antimony alloy was analyzed in detail and appropriate recovery methods were developed. A reduction method by adding gold-antimony alloy powder was adopted to recover silver according to the thermodynamics calculation. The reducing rate of silver can exceed 99% at 80 ℃ for 1.5 h when the dosage of gold-antimony alloy powder is 10%. The dissolution equilibrium curved surfaces of PbSO4 and PbCl2 under different conditions were drawn. The experimental results are well consistent with theoretical analysis that indicate lead may be precipitated in the form of lead chloride. The grade of gold in the residue can be further concentrated to 94.5% after being washed with hot water. These two methods have been applied successfully in the practice.
Key words: silver chloride; lead chloride; gold-antimony alloy; chlorination leaching
1 Introduction
Gold-antimony alloy is an intermediate when gold-antimony concentrate is smelted in the blast furnace. Then the gold-antimony alloy is treated by the oxidation-blowing, antimony electrolysis, crucible smelting and muffle-blowing, and the gold-silver alloy is finally produced. With the development of the antimony metallurgical technology in recent years, the grade of antimony in the gold-antimony concentrate is becoming lower and the contents of impurities are becoming higher, which will be further enriched in the gold-antimony alloy in the process, and finally the impurities in the gold-antimony alloy make the original operations run more and more difficultly [1-4].
On the basis of the problems in the original process and the developing demands of comprehensive recovery of valuable metals, a new hydro-technology of separating precious metals from base metals in gold-antimony alloy has been developed by Central South University in China. The new method of selective chlorination leaching under controlled potential is adopted to treat gold-antimony alloy. In order to choose appropriate silver recovery method, it is necessary to understand the existing form of silver. Meanwhile, lead must be removed for raising the grade of gold in the leaching residue. The selecting method must rely on the lead phase in the leach residue[3-5].
According to the properties of the selective chlorination leaching process, the form of silver in the solution and lead phase in the leach residue can be determined. Based on the calculation results, the reduction method by adding gold-antimony alloy was adopted to recover silver in the solution, and lead was removed in the leach residue by washing with hot water. The experimental results show that the two methods are feasible and the expected effect is achieved. These two methods have been applied successfully in the practice.
2 Selective chlorination leaching of gold- antimony alloy
The raw material is the gold-antimony alloy (particle size ≤147 μm) produced by an antimony smelter of Hunan Province, China. The chemical compositions of the gold-antimony alloy are listed in Table 1.
Table 1 Chemical compositions of gold-antimony alloy (mass fraction, %)

In order to separate the precious metals from the base metals in the gold-antimony alloy, gold-antimony alloy was leached in the mixture solution of hydrochloric acid and sulfuric acid. The oxidation potential of the solution was controlled by adding hydrogen peroxide to oxidize the base metals, such as copper, nickel and antimony. These base metals entered into the solution while the precious metals and lead remained in the residue[5].
Gold-antimony alloy was leached under the following conditions: the concentration of hydrochloric acid [HCl]=4.0 mol/L, the concentration of sulfuric acid [H2SO4]=1.2 mol/L, liquid-solid ratio (L?S)=7.5?1, the ending oxidation potential 400 mV (relative to calomel electrode), temperature 80 ℃, time 2 h and stirring speed 200 r/min. The compositions of the solution and residue are listed in Table 2.
Table 2 Compositions of leaching solution and residue
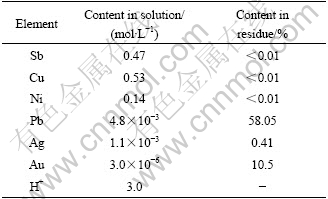
Table 2 shows that the base metals such as copper, nickle and antimony can be removed effectively in the leaching procedure. The leaching rates of copper, nickel and antimony are more than 99%.
However, it can be seen from Table 2 that part of silver is dissolved in the leaching solution. So, it is necessary to understand the exsiting form of silver in the solution for recycling it. The grade of gold in the residue is low because of high lead content. So, lead must be removed for concentrating gold further, and the method must rely on the form of lead phase in the leaching residue.
3 Behavior of silver in solution
Though silver in the gold-antimony alloy will be oxidized and precipitated as silver chloride in the leaching procedure, part of silver can be dissolved as the complex ions of Ag+ and Cl- due to the complexation of chloride ion.
3.1 Calculation of equilibrium concentration of chloride ion
When more complex ions of metals and chloride are formed in the solution, the equilibrium concentration of chloride ion ([Cl-]) and the total concentration of chloride ion ([Cl-]T) are different. The equilibrium concentration of chloride ion [Cl-] can be calculated [6-7].
There are many known conditions, such as the total concentration of each metal ion ([Mj]T), the total concentration of chloride ion ([Cl-]T), accumulated equilibrium constant of complex ions βi. According to the mass balance of chloride ion, the following equation can be obtained:
(1)
and
[MjCli]=βi[Mj][Cl-]i (2)
(3)
Substituting Eqs.(2) and (3) into Eq.(1), then
(4)
It can be seen from Eq.(4) that when [Cl-]T is constant, [Cl-] is affected by two factors. They are the concentration of metal ions, [Mj]T, and the accumulated equilibrium constant of complex species of each metal, βi.
There are many metal ions that can combine with chloride ion in the solution, mainly as Ag+, Pb2+, Sb3+, Cu2+ and Au+. Because the concentration of Au+, Ag+ and Pb2+ in the solution is too low, the complexation reaction of Au+, Ag+ and Pb2+ with chloride ion can be neglected. Therefore, the complexation reactions of Sb3+ and Cu2+ with chloride ion are considered.
When the accumulated equilibrium constant of complex ions of each metal shown in Table 3 and the concentration of each metal ion shown in Table 2 are substituted into Eq.(4), [Cl-] is obtained as
=0
(5)
Table 3 Accumulated equilibrium constant of complexing ions of each metal[8-9]

The equilibrium concentration of chloride ion ([Cl-]) is 2.40 mol/L when Eq.(5) is calculated with Matlab software. If lead is precipitated in the form of PbCl2 in the leaching process, the equilibrium concentration of chloride ion will decrease by 0.20 mol/L. Therefore, [Cl-] may be 2.20 mol/L or 2.40 mol/L.
3.2 Distribution of
complex ion
The silver ion (Ag+) can react with chloride ion (Cl-) in the solution to form a series of complex ion
(i=1, 2, 3, 4). The total complexation reaction is shown as follows:
(i=1, 2, 3, 4)
(6)
The total concentration of silver ion ([Ag+]T) in the solution is calculated as follows:
(7)
The proportion of Ag+, AgCl, AgCl2-, AgCl32- and AgCl43- to [Ag+]T in the solution is represented by φi (i=0, 1, 2, 3, 4), respectively. They are described as
(8)
It can be seen from Eq.(8) that the proportions of AgCli1-i complex ions relate to the equilibrium concentration of chloride ion ([Cl-]) in the solution. The correlation of all complex ions with equilibrium concentration of chloride ion is shown in Fig.1.
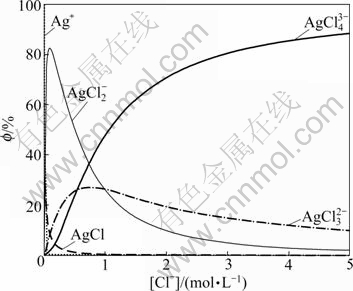
Fig.1 Distribution of complex ions of Ag+ and Cl-
Fig.1 shows that the proportions of all complex ions of Ag+ and Cl- are varied with [Cl-]. When [Cl-]=2.20 mol/L or 2.40 mol/L, the silver in the solution mainly exists in the form of AgCl43-, and it accounts for about 70% or 75 %.
3.3 Thermodynamic analysis and preliminary experiment
3.3.1 Thermodynamic analysis of silver reduction
According to the principle of simultaneous equilibrium, when metallic silver is in equilibrium with the various complex ion of Ag+ and Cl- in solution, the equilibrium electrode potential can be expressed by one of them[10-11]. The electrode potential is given by Nernst equation
Ag++e=Ag,
(9)
The equilibrium concentration of silver ion in the solution is given as Eq.(6) when the complexation reaction of Ag+ and Cl- is carried out. Substituting Eq.(6) into Eq.(9), the equilibrium potential of silver in the solution can be described as

(10)
It can be seen from Eq.(10) that the equilibrium electrode potential of silver in solution is affected by those factors, such as accumulated equilibrium constant (βi), concentration of complex ion
and equilibrium concentration of chloride ion ([Cl-]).
The equilibrium electrode potential of silver in solution is 0.220 8 V or 0.213 6 V when [Cl-]=2.20 mol/L or 2.40 mol/L. The equilibrium electrode potential of silver in solution decreases little when the equilibrium concentration of chloride ion increases. It is possible to reduce the silver in solution if the standard electrode potential of some metals is below 0.213 6 V. The standard electrode potentials of lead, antimony and nickel in gold-antimony alloy powder are -0.126, 0.1 and -0.25 V, respectively. So, gold-antimony alloy powder can be adopted to reduce silver in solution.
3.3.2 Preliminary experiment
When 150 mL of leaching solution (as shown in Table 2) was heated up to 80 ℃, 2 g of gold-antimony alloy powder was charged into. The liquid was cooled and filtrated after stirring for 2 h. The concentration of silver ion in the solution is decreased from 122 mg/L to 1.25 mg/L, and the reducing rate of silver can exceed 99%.
3.4 Results and discussion
It could be seen from the result of preliminary experiment that silver ion in the solution can be reduced by adding gold-antimony alloy powder. In order to establish the optimum conditions, the effects of the dosage of gold-antimony alloy powder, temperature and time on the reducing rate of silver ion have been investigated[12-13].
3.4.1 Effect of dosage of gold-antimony alloy powder on reducing rate of silver
The effect of dosage of gold-antimony alloy powder on the reducing rate of silver was investigated at 80 ℃ for 1.5 h. The result is shown in Fig.2.
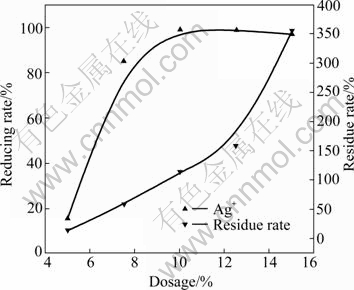
Fig.2 Effect of dosage of gold-antimony powder on reducing rate of silver ion
It can be seen from Fig.2 that the reducing rate of silver ion increases with increasing dosage of gold- antimony alloy powder. When the dosage of gold- antimony alloy powder is 10%, the reducing rate of silver ion can reach 99%. When the dosage of gold- antimony alloy powder is increased to 15%, the reducing rate of silver ion still keeps about 99%, but the residue rate increases sharply. The residue rate is the mass fraction of residue and gold-antimony alloy powder. This is because antimony chloride in the solution is hydrolyzed when the acidity decreases by adding excess gold-antimony alloy. Therefore, the dosage of gold- antimony alloy powder is chosen to be 10%.
3.4.2 Effect of temperature on reducing rate of silver ion
When the dosage of gold-antimony alloy powder was 10% and reaction time was 1.5 h, the effect of temperature on the reducing rate of silver ion was investigated. The result is shown in Fig.3.
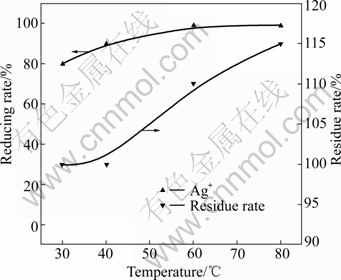
Fig.3 Effect of temperature on reducing rate of silver ion
Fig.3 shows that the reducing rate of silver ion gradually increases with increasing temperature. The reducing rate of silver ion can reach 99% when the temperature is 80 ℃. So, increasing temperature is benefical to improving the reducing rate. Therefore, 80 ℃ is the appropriate temperature in the reduction process.
3.4.3 Effect of time on reducing rate of silver
With the dosage of gold-antimony alloy powder of 10% and temperature of 80 ℃, the effect of reaction time on the reducing rate of silver ion was investigated. The result is shown in Fig.4.
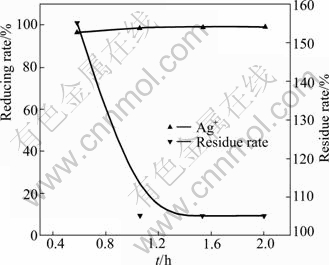
Fig.4 Effect of time on reducing rate of silver ion
Fig.4 shows that when the time is prolonged, the reducing rate of silver ion keeps about 99% and does not change, but the residue rate decreases constantly. When the time exceeds 1 h, the residue rate of silver ion maintains at about 105%. Prolonging time is beneficial to the oxidation-reduction equilibrium in the reducing process. So, the time is fixed at 1.5 h.
3.4.4 Summary
The optimum reduction conditions are determined as follows: dosage of gold-antimony alloy powder of 10%, temperature of 80 ℃ and time of 1.5 h. Under these conditions the reducing rate of silver ion can reach 99%. Therefore, it is feasible to recycle the silver in the solution by adding gold-antimony alloy powder.
4 Behavior of lead
When there are two or more kinds of slightly soluble compounds or insoluble compounds, the compound with less solubility product will be precipitated firstly. So, PbSO4 will be precipitated according to the solubility product of PbSO4 and PbCl2. But, the solubility of PbCl2 and PbSO4 will change in the acidic solution containing Cl- and SO42-, and the precipitates of PbCl2 or PbSO4 will be gained under different conditions. Because there are more influential factors, such as common ion effect, complexation reaction of Pb2+ and Cl-, protonation equilibrium of SO42- [14].
4.1 Ion reaction equilibrium
4.1.1 Solubility product of PbCl2 and PbSO4
The precipitates of PbCl2 and PbSO4 may be formed in the leaching procedure.
PbCl2=Pb2++2Cl-, Ksp(PbCl2)=[Pb2+][Cl-]2 (11)
PbSO4=Pb2++SO42-, Ksp(PbSO4)=[Pb2+][SO42-] (12)
The solubility products of PbCl2 and PbSO4 at 298 K are 10-4.68 and 10-7.42, respectively, and are 10-4.18 and 10-6.94 at 348 K, respectively[15].
4.1.2 Complexation equilibrium of Pb2+ and Cl-
Pb2+ can react with Cl- to form a series of complexes ions (PbCli2-i). Their equilibrium equations are shown as follows:

(i=1, 2, 3, 4, 5) (13)
The accumulated equilibrium constants of PbCli2-i at 25 ℃ and 75 ℃ are shown in Table 3.
4.1.3 Protonation equilibrium of SO42-
The proton reaction of SO42- and H+ will occur to form HSO4- in the acidic solution, and the concentration of sulfate ion ([SO42-]) will vary with the pH value[16]. The proton equilibrium of sulfate ion is shown as
(14)
where K1H is the protonation constant of SO42-, which is 101.997 at 298 K and 102.546 at 348 K, respectively.
(15)
So, the equilibrium concentration of sulfate ion in the solution is described as
(16)
4.2 Dissolution equilibrium of PbSO4 in solution
If the precipitate of PbCl2 does not form, the equilibrium concentration of lead ion ([Pb2+]) in the acidic solution containing Pb2+, Cl- and SO42- is offered only by the dissolution of PbSO4. This can be expressed by Eq.(12). Meanwhile, considering the complexation of chloride ion (Cl-), the total concentration of lead ion in the solution is described as
(17)
And the equilibrium concentration of sulfate ion is described as Eq.(16). Substituting Eqs.(12) and (16) into Eq.(17), the total concentration of lead ion is obtained as

(18)
As Eq.(18) shown, when the dissolution equilibrium of PbSO4 in this solution is achieved, the dissolution equilibrium curved surface of [Pb2+]T with [SO42-] and [Cl-] under specific concentration of hydrogen ion can be drawn as the curved surface 1 in Fig 5.
4.3 Dissolution equilibrium of PbCl2 in solution
Provided that the precipitate of PbSO4 is not formed, the equilibrium concentration of lead ion ([Pb2+]) in the acidic solution is offered only by the dissolution of PbCl2. This can be expressed by Eq.(11).
Meanwhile, considering the complexation of chloride ion, the total concentration of lead ion in the solution is shown as Eq.(17).
Substituting Eq.(11) into Eq.(17), the total concentration of lead ion is obtained as
(19)
As Eq.(19) shown, when the dissolution equilibrium of PbCl2 in this solution is achieved, the dissolution equilibrium curved surface of [Pb2+]T with [SO42-] and [Cl-] under specific concentration of hydrogen ion can be drawn as the curve surface 2 in Fig 5.
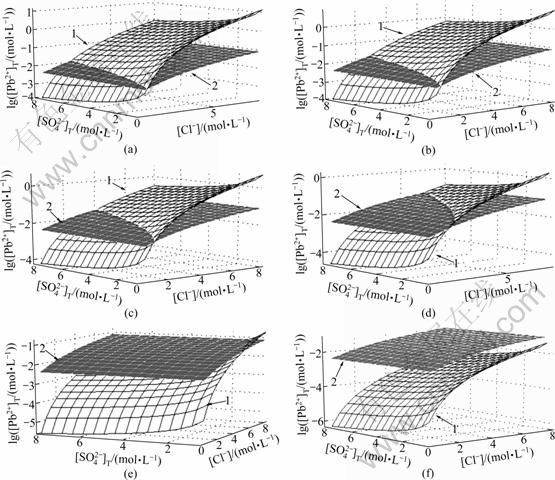
Fig.5 Dissolution equilibrium curved surfaces of PbSO4 and PbCl2 at 298 K in different [H+] (1—PbSO4; 2—PbCl2): (a) [H+]=7.5 mol/L; (b) [H+]=5.0 mol/L; (c) [H+]=2.5 mol/L; (d) [H+]=1.0 mol/L; (e) [H+]=0.1 mol/L; (f) [H+]=0.01 mol/L
4.4 Analysis of precipitate conditions
The dissolution equilibrium curved surfaces of the solubility of PbSO4 and PbCl2 with [SO42-] and [Cl-] under different concentration of hydrogen ion [H+] at 298 K are shown in Fig.5. The curved surfaces 1 and 2 represent the compound of PbSO4 and PbCl2, respectively. According to the principle of solubility product, the compound with less solubility product will be precipitated firstly when there are more than two kinds of insoluble compounds. So, the compound represented with the lower curved surface in Fig.5 will be precipitated firstly.
The intersection line of the two curved surface indicates that the dissolution equilibrium of PbSO4 and PbCl2 is achieved simultaneously. The area is divided into the precipitation zone of PbSO4 and the precipitation zone of PbCl2 by this curve projected from the intersection line. When [H+] values are 7.5, 5.0, 2.5, 1.0 and 0.1 mol/L, respectively, and temperature is 298 K and 348 K, respectively, the dissolution equilibrium curves of PbSO4 and PbCl2 are drawn in Fig.6.
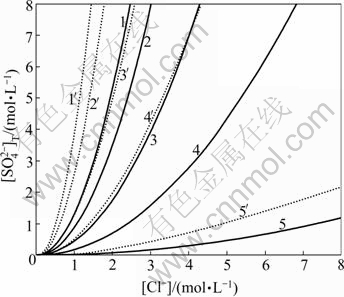
Fig.6 Dissolution equilibrium curves of PbCl2 and PbSO4 under different conditions: 1—298 K, [H+]=7.5 mol/L, 1′—348 K, [H+]=7.5 mol/L; 2—298 K, [H+]=5.0 mol/L, 2′—348 K, [H+]=5.0 mol/L; 3—298 K, [H+]=2.5 mol/L, 3′—348 K, [H+]=2.5 mol/L; 4—298 K, [H+]=1.0 mol/L, 4′—348 K, [H+]=1.0 mol/L; 5—298 K, [H+]=0.1 mol/L, 5′—348 K, [H+]=0.1 mol/L
It can be seen from Fig.6 that the precipitation zones of PbSO4 and PbCl2 are above and below the curves, respectively. The precipitation zone of PbSO4 will reduce gradually when [H+] increases. The precipitation zone of PbCl2 will enlarge with increasing temperature, which is beneficial to precipitation of PbCl2.
4.5 Example analysis for removing lead
When [Cl-] is 2.4 mol/L and [H+] is 3.0 mol/L, it can be found from Fig.6 that lead may be precipitated in the form of lead chloride. The lead phases in the leaching residue are listed in Table 4.
Table 4 Lead phase of leaching residue in leaching process
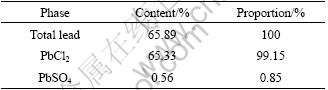
Table 4 shows that more than 99% of lead in the leaching residue is lead chloride and only about 1% is lead sulfate. It can be concluded that the results of practice are consistent with theoretical analysis. Lead sulfate in the residue is formed because the concentration of hydrogen ion decreases when the leaching residue is washed with hot water.
Lead in the leaching residue must be removed for further concentrating gold. Based on the solubility of lead chloride at different temperatures, the method of washing with hot water is adopted to remove lead chloride. The leaching residue is washed firstly by hot hydrochloric acid of 4 mol/L for avoiding hydrolysis of SbCl3, and then washed with hot water of 95 ℃ for removing PbCl2 in the leaching residue. The chemical components change of the leaching residue before and after washing is listed in Table 5.
Table 5 Chemical components change of residue before and after washing
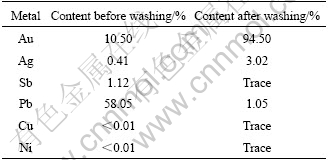
It can be seen from Table 5 that the grade of gold increases to 94.50% in the washed residue from 10.50% in the leaching residue. Therefore, the aim of concentrating gold is achieved. The content of lead in the crystallized residue from washing water can reach 64.51%.
5 Conclusions
1) A novel selective chlorination process is developed to treat gold-antimony alloy. The base metals in the gold-antimony alloys, such as copper, nickel and antimony, can be effectively removed by the leaching process. The behavior of silver and lead in the solution is investigated.
2) According to the thermodynamics calculation, the silver complex species in the solution mainly exist in the form of AgCl43-, whose proportion is 75% when [Cl-] is 2.40 mol/L. A reduction method by adding gold- antimony alloy powder is adopted to recover silver. The reducing rate of silver ion can exceed 99% at 80 ℃ for 1.5 h when the dosage of gold-antimony alloy powder is 10%.
3) The dissolution equilibrium curved surfaces of PbSO4 and PbCl2 at different conditions are drawn through calculation. It is found that lead is precipitated in the form of lead chloride, which is perfectly consistent with the theoretical analysis. The grade of gold is increased up to 94.50% by washing residue with hot water.
References
[1] MYZENKOV F A, TARAOV A V. Development and creation of modern metallurgical technology for antimony production with gold recovery from domestic gold-antimony concentrates in Russia [C]// KONGOLI F. Proceedings of Sohn International Symposium. San Diego: TMS, 2006: 121-128.
[2] LIU Wei-feng. Study on the selective chlorination separation of precious metals from base metals in gold-antimony alloy by controlling potential [D]. Changsha: Central South University, 2006. (in Chinese)
[3] YANG Tian-zu, LIU Wei-feng, JIANG Ming-xi. The separation of precious metals from base metals in gold-antimony alloy by selective chlorination leaching under controlling potential [C]// KONGOLI F. Proceedings of Sohn International Symposium. San Diego: TMS, 2006: 279-292.
[4] LIU Yong, YANG Tian-zu, CHEN Fang-bin, LIU Wei-feng. A method for concentrating precious metals from gold-antimony alloy: CN200510032598.3 [P]. 2005. (in Chinese)
[5] LIU Wei-feng, YANG Tian-zu, JIANG Ming-xi, LIU Wei, DOU Ai-chun. Practice of separating precious metals from base metals in gold-antimony alloy by selective chlorination leaching under controlling potential [C]// HOWARD S M. Proceeding of Sessions and Symposia Sponsored by EPD of TMS. New Orleans: TMS, 2008: 479-485.
[6] YANG Tian-zu, LIU Zhao-hui, BIN Wan-da, TANG Jian-jun. The behavior of impurities during gold reduction with oxalic acid from chlorination solution [J]. Gold, 1999, 20(3): 31-34. (in Chinese)
[7] YANG Xian-wan, LI Dong-fang. Thermodynamic analysis for the selected chlorination leaching at controlled potential [J]. Precious Metals, 1990, 11(4): 1-7. (in Chinese)
[8] MARTELL E, SMITH R M. Critical stability constants (Inorganic complex) [M]. New York: Plenum Press, 1974.
[9] DEAN J A. Lange’s chemistry handbook [M]. 15th Version. New York: McGran-Hill Education Co., 1999.
[10] GUO Zhao-hui, LIAO Bo-han, HUANG Chang-yong. Leaching potential and changes in components of metals in two acidic ferrisols [J]. Journal of Central South University of Technology, 2006, 13(6): 631-636.
[11] ZHUANG Du-chao, DU Xin-ling, YANG Tian-zu, DU Zuo-juan, DOU Ai-chun. Study on leaching of silver chloride in sodium sulfite solution containing chloride ions [J]. Precious Metals, 2007, 28(3): 10-14. (in Chinese)
[12] DUTRIZAC J E. The leaching of sulphide minerals in chloride media [J]. Hydrometallurgy, 1992, 22(2): 40-45.
[13] CANAL J P, RAMNIAL T, LANGLOIS L D, ABERNETHY C D, CLYBURNE J A C. A three-step laboratory sequence to prepare a carbene complex of silver chloride [J]. Journal of Chemical Education, 2008, 85(3): 416-419.
[14] XIA Xing, YANG Tian-zu, LIU Wei-feng, DOU Ai-chun, DU Zuo-juan. Study on the behavior of lead in the process of selective chlorination leaching of precious metals from base metals in gold-antimony alloy [J]. Precious Metals, 2008, 29(1): 5-10. (in Chinese)
[15] TANG Mo-tang, CHEN Jin-zhong, CAI Chuan-suan. A new technology for treating the residues from chlorination leaching [J]. Journal of Central South University of Technology: Nature Science, 1996, 27(2): 164-167. (in Chinese)
[16] PENG Xiao-yu, WANG Yun-yan, CHAI Li-yuan, SHU Yu-de. Thermodynamic equilibrium of CaSO4-Ca(OH)2-H2O system [J]. Transaction of Nonferrous Metals Society of China, 2009, 19(1): 249-252.
Foundation item: Project(Hunan 2006104) supported by the Key Project Science and Technology Program of Hunan Province of China
Corresponding author: LIU Wei-feng; Tel: +86-731-88836791; E-mail: liuweifeng1214@163.com
DOI: 10.1016/S1003-6326(09)60141-2
(Edited by YUAN Sai-qian)