
Fractal analysis of crack paths in Al2O3-TiC-4%Co composites
LI Jing(李 静)1, 2 , YIN Yan-sheng(尹衍升)1 , LIU Ying-cai(刘英才)1, MA Lai-peng(马来鹏)3
1. Institute of Materials Science and Engineering, Ocean University of China, Qingdao 266003, China;
2. Department of Materials Science and Engineering,
Shandong Institute of Architecture and Engineering, Ji’nan 250101, China;
3. Key Laboratory for Liquid Structure and Heredity of Ministry of Education,
Shandong University, Ji’nan 250061, China
Received 26 October 2005; accepted 27 February 2006
Abstract: Al2O3-TiC-4%Co(volume fraction) composites(ATC) with high toughness (7.8±0.8 MPa·m1/2) and strength (782±60 MPa) were fabricated. In comparison with Al2O3-TiC composites(AT), the fracture toughness was significantly improved by 60%. The crack paths, generated by Vickers indentation on the polished surfaces of both composites, were analyzed from a fractal point of view to distinguish the possible toughening mechanisms involved. Quantitative evaluation of indentation cracks indicates that the crack deflection plays a more effective role. Cracks of the ATC composites show higher deflection angles and more deflections along the path. ATC composites present higher fractal dimension (D=1.07) than AT composites (D=1.02), which is directly related to the higher fracture toughness. A significant relationship between crack path and toughness is evident: the more irregular the geometry of the crack, the higher the fracture toughness.
Key words: Al2O3-TiC-Co; Al2O3-TiC; fractal; toughening mechanism; indentation crack
1 Introduction
Al2O3-TiC composite (denoted by AT) is an important material for structural components due to the high strength, hardness, as well as chemical stability and wear resistance. However, the low fracture toughness still cannot match the command of many practical fields, which greatly limits its applications[1,2]. SHI[3] and YIN et al[4] prepared a novel Al2O3-TiC-Co composite (denoted by ATC). In comparison with the conventional AT composites, significant improvement in fracture toughness and fracture strength was obtained in this novel composite. In this paper, preliminary investigation was carried out to obtain a better understanding of the fracture behavior from a fractal point of view.
Fractal geometry, developed by MANDELBROT [5], has been successfully used to describe quantitatively complex shapes such as fracture surfaces in metal, ceramics and polymeric materials. This approach represents a new way to quantitatively characterize the topography of a surface. Fractal parameter provides a measurement for the irregularity of curves described. Fracture surface analysis, by fractal geometry, has been applied to distinguish the geometrical effects of different fracture mechanisms[6]. For several ceramic materials, a direct correlation between fracture toughness and the fractional part of the fractal dimension has been found [7,8]. Recently, it is found that the fractal analysis of crack profile also proves to be an effective way to characterize the geometrical effects of different fracture mechanisms. Thus, the present study involved the investigation of fracture processes by analyzing the geometrical features of crack profiles instead of fracture surfaces. The crack paths, generated by Vickers indentation on the polished surfaces of both materials, were analyzed to attain information about the toughening mechanisms involved. It is well known that the fracture strength and toughness of Al2O3 ceramics can be increased by the addition of TiC particles. The resulting toughening effect is largely due to crack deflection mechanism besides grain refinement effects. The crack profile (or irregularity) then is closely related to the crack deflection in this system. Since fractal analysis allows one to quantify crack irregularity, it is possible to evaluate the contribution of the crack deflection mechanism in controlling crack propagation.
2 Experimental
Al2O3 (average particle size about 70 nm) and TiC (average particle size about 130 nm) were coated with cobalt film by chemical deposition, respectively. The coated powders with the mass ratio of 70:30 (Al2O3:TiC) were then homogenized by ball milling as the starting powders, which were supplied by the coworkers of Zhejiang University. General description of the coating process has been described in Refs.[9,10]. Cobalt coated powders were hot pressed (30 MPa) at 1 650 ℃ in vacuum for 30 min (Model HIGH MULTI 5000, FUJIDENPA). By contrast, Al2O3-TiC composites were also prepared by hot pressing in vacuum at 1 700 ℃ for 30 min and 30 MPa using the uncoated powders[11].
Sintered specimens were cut into bars. The final dimensions of these samples were 3 mm×4 mm×36 mm and 4 mm×2 mm×36 mm for measuring bending strength and fracture toughness, respectively. The bending strength of samples was measured by a three-point bending test with a support roller span length of 20 mm and a crosshead speed of 0.5 mm/min. The fracture toughness(KIC) was measured by the single- edge notch beam(SENB) method with the span length of 20 mm and crosshead speed of 0.05 mm/min.
The density was measured by the Archimedes method. The grain sizes of Al2O3-TiC matrix were estimated by the linear intercept method using micrographs of thermally etched surfaces. Cracks were induced on polished surfaces by Vickers indentation at a load of 294 N to observe the crack propagation. The fracture surfaces and indented cracks were investigated using a scanning electron microscope (Model S-2500, Hitachi) equipped with an EDX system for elemental analysis.
3 Result and discussion
The properties of two materials are listed in Table 1. With the addition of cobalt phase into the Al2O3-TiC matrix from the coated ceramic powder, significant improvement in both toughness and strength has been realized[12]. The fracture toughness of ATC composites is as high as 7.8±0.8 MPa?m1/2, 60% higher than that of AT composites. The bending strength (782±60 MPa) is also improved by 40%. For the ATC composites, the average grain size (2.8 mm) is smaller than that (3.1 mm) of the AT composites, revealing the drag effect of cobalt particles on the grain boundary mobility. The ATC composites can reach nearly full density (99.2%) at lower temperature. It is thought that the favorable wettability between cobalt and TiC promotes the densification process through liquid phase sintering (the melting point of cobalt is 1 493 ℃)[13].
Fig.1 shows the fracture surface of AT composite and ATC composite. Previous studies have indicated that the fracture of AT composites proceeded by the typical intergranular fracture[14]. By contrast, the fracture surface of ATC composites is a mixture of transgranular and intergranular type, presenting a more complicated fracture mode. From the fractal point of view, the irregularity of this complicated fracture mode should be higher than the single intergranular fracture in AT composites, which is in favor of the improvement in toughness.
Table 1 Properties of ATC and AT composites

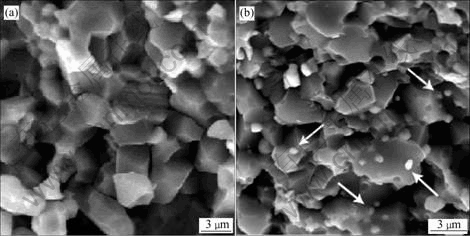
Fig.1 Fracture surfaces of AT(a) and ATC(b) (Arrows indicate cobalt particles)
Further investigations were carried out on the crack paths produced by Vickers indentation, as shown in Fig.2. In AT composites, the crack proceeds without obvious deflection, generating a planar fracture path. This is consistent with previous results that the cracks tend to propagate directly through the TiC particles with little crack deflection[14]. For ATC composites, however, it is very common to see that crack deviates obviously from the direction of pre-crack and the crack deflection appears to be more effective. Crack bridging is observed in both composites. On the whole, the crack propagation in the ATC composites is characterized by a more tortuous path, which will increase the propagating resistance of cracks.
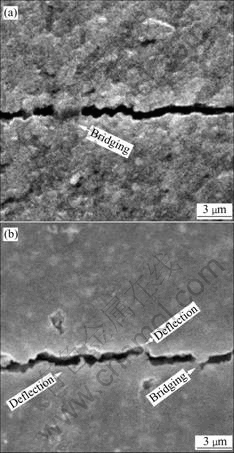
Fig.2 SEM images of Vickers indentation crack on polished surfaces of AT(a) and ATC(b)
To obtain a quantitative understanding of this result, the crack profiles were analyzed according to their geometric characteristics. The crack deflection angles and corresponding distribution of deflection number were measured within selected areas, as shown in Table 2. Meanwhile, the percent frequency of deflection angles in the range from 0? to 90? is plotted in Fig.3. For the AT composites, cracks are characterized by a high percentage of low deflection angles (0?-60?) and a small number of deflections along the path. Cracks of the ATC composite, in contrast, show a high percentage of deflection angles in the range of 30?-75?, together with more deflections. On the whole, the cracks appear to be geometrically different due to the addition of Co phase.
Table 2 Crack deflection angles and corresponding number of deflection
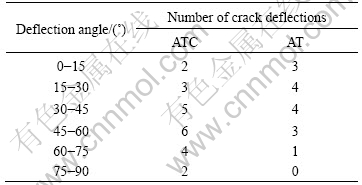
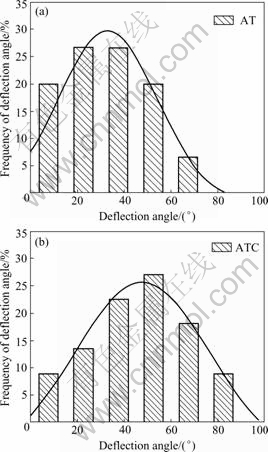
Fig.3 Frequency of crack deflection angles for AT(a) and ATC(b)
The fractal parameter D allows one to distinguish different crack profiles on the basis of their particular geometry (or irregularity). The method for characterizing the coastline by MANDELBROT is applied to the calculation of fractal dimension[5]. When the magnification increases or the size of the measuring unit decreases, the length of an irregular curve increases. This effect is described by the following equation:
L(e)=L0e –[D–1] (1)
where L is the real length, L0 is a constant with dimension of L, e is the size of the measuring unit and D, varying between 1 and 2, represents the fractal dimension of the curve. (D-1) =D* is the fractional part of the fractal parameter D of the irregular profile. The linear form of equation (1) is
lnL(e)=lnL0-[D-1]lne (2)
The parameter D can be obtained from the slope of the straight line obtained in the plot lnL(e) vs lne.
The processes are as follows:
First, the lengths of crack were measured from the SEM images of cracks that were magnified by different magnifications (1.0×, 1.5×, 2.0×, 2.5×, 3.0×), as shown in Table 3. Next, the crack lengths were converted into the real crack lengths with different measuring units at the same magnification, as shown in Table 4. Then the corresponding ln(e) and lnL(e) values were calculated, as shown in Table 5. Last, the D value was calculated from Eqn.(2) by linear fitting, as shown in Fig.4. The resultant D values of AT and ATC composites are 1.02 and 1.07, respectively. Apparently, the irregularity of cracks in ATC is larger than that in AT composites.
Table 3 Lengths of cracks by direct measurement (correspond- ing to different magnifications)
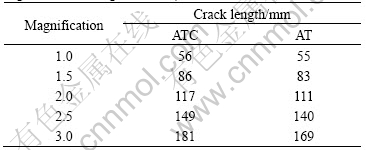
Table 4 Real crack lengths after conversion (corresponding to different measuring units)
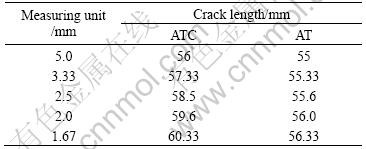
In view of the above results, it is obvious that the increase in fracture toughness is closely related to the
Table 5 Natural logarithm values of data in Table 4
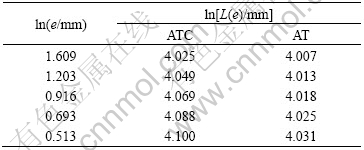
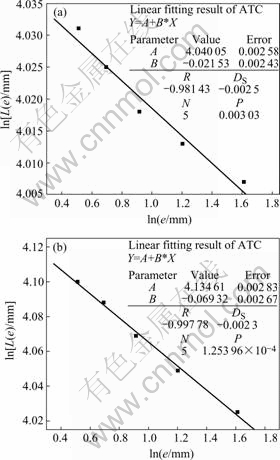
Fig.4 Linear fitting results by equation (2), giving D values of AT(a) and ATC(b)
increase in fractal dimension D. Previous studies indicate that fracture toughness (KIC) is positively proportional to D value for most ceramic materials, which is expressed as
KIC=K0+A(D-1)1/2 (3)
where K0 is the toughness value for a smooth planar fracture and A is a constant. The materials are divided into classes, characterized by the same value of A, corresponding to similar microstructural features and fracture behaviors. In this study, the results confirm this relationship. The higher fracture toughness of ATC composites corresponds to a higher fractal dimension D.
The toughening mechanisms of the Al2O3-TiC composites are commonly attributed to the crack deflection effect of TiC particles[1]. For ATC composites, the Co content amounts to only 4%(volume fraction), which is not enough to offer direct deflection or bridging. Therefore, we might infer from these results that the improvement in fracture behavior should be mainly attributed to the improved crack deflection effect of TiC particles instead of Co phase. In other words, the added Co phase promotes the crack deflection mechanism of TiC particles, resulting in the toughness improvement. This can also be expressed by the apparently increased irregularity of ATC composites. It is well known that the fracture strength improvement can result from the fracture toughness increment and the grain size reduction according to Griffith fracture theory[15]. For the ATC composites, the average grain size is smaller than that of the AT composites (Table 1). Therefore, it is reasonable to assume that a combination of the improvement in the fracture toughness and the reduction in the grain size should be responsible for the enhancement in fracture strength.
4 Conclusions
Al2O3-TiC-4%Co(volume fraction) composites with high toughness and strength were successfully hot pressed from cobalt-coated powders. As compared with the conventional Al2O3-TiC composites, ATC composites show a change of fracture mode from intergranular to a mixture of transgranular and intergranular type. Crack deflection plays an effective role in the ATC composites, generating a more irregular path with higher deflection angle and deflection number. Quantitative evaluation by fractal analysis of crack paths in both composites indicates that ATC composites show higher fractal dimension (or irregularity) than AT composites, which is positively proportional to the higher fracture toughness. The added Co phase promotes the crack deflection mechanism of TiC particles, resulting in the toughness improvement.
References
[1] WHAI R P, ILSCHNER B. Fracture behavior of composites based on Al2O3-TiC [J]. J Mater Sci, 1980, 15: 875-885.
[2] LEE J H, KO S K, WON C W. Sintering behavior of Al2O3-TiC composite powder prepared by SHS process [J]. Materials Research Bulletin, 2001, 36: 989-996.
[3] SHI Rui-xia. Preparation and study of Co-coated Al2O3/TiC composite [D]. Ji’nan: Shandong University, 2004
[4] YIN Y S, SHI R X, MA L P. Sintering and mechanical properties of Al2O3/TiCp composites [J]. Interceram, 2004, 53: 24-27.
[5] MANDELBROT B B. Fractal: Form, Chance and Dimension [M]. San Francisco: W H Freeman, 1977.
[6] CELLI A, TUCCI A, ESPOSITO L. Quantitative evaluation by fractal analysis of indentation crack paths in Si3N4-SiCw composites [J]. J Eur Ceram Soc, 1999, 19: 441-449.
[7] MECHOLSKY J J, PASSOJA D E, FEINBERG-RINGEL K S. Quantitative analysis of brittle fracture surfaces using fractal geometry [J]. Journal of American Ceramic Society, 1989, 72: 60-65.
[8] THOMPSON J Y, ANUSAVICE K J, BALASUBRAMANIAM B, MECHOLSKY J J. Effect of microcracking on the fracture toughness and fracture surface fractal dimension of lithia-based glass ceramics [J]. Journal of American Ceramic Society, 1995, 78: 3045-3049.
[9] HUANG L P, LI J. Properties of Cobalt-reinforced Al2O3-TiC ceramic matrix composite made via a new processing route [J]. Composites: Part A, 1999, 30: 615-618.
[10] LI J, SUN J L, HUANG L P. Effects of ductile cobalt on fracture behavior of Al2O3-TiC ceramic [J]. Mater Sci Eng A, 2002, 323: 17-20.
[11] MA Lai-peng. Mechanical properties and strengthening and toughening mechanism of Co-coated Al2O3/TiC composite [D]. Ji’nan: Shandong University, 2005.
[12] LI J, MA L P. Influence of cobalt phase on mechanical properties and thermal shock of performance of Al2O3-TiC composites [J]. Ceramic International, 2005, 31: 945-951.
[13] KINGERY W D, NIKI E, NARASHIMHAN M D. Sintering of oxide and carbide-metal compositions in presence of a liquid phase [J]. J Am Ceram Soc, 1961, 44: 29-35.
[14] GONG J, MIAO H, ZHAO Z. The influence of TiC-particle-size on the fracture toughness of Al2O3-30wt.%TiC composites [J]. J Euro Ceram Soc, 2001, 21: 2377-2381.
[15] LU J S, GAO L, SUN J. Effect of nickel content on the sintering behavior, mechanical and dielectric properties of Al2O3/Ni composites from coated powders [J]. Mater Sci Eng A, 2000, 293: 223-228.
(Edited by LI Xiang-qun)
Corresponding author: YIN Yan-sheng; Tel: +86-532-82031795; E-mail: yys2003@ouc.edu.cn