Electrochemical performances and structure characteristic of LiMn2O4-xYx (Y=F, Cl, Br) compounds
CHEN Zhao-yong(陈召勇), ZHU Hua-li(朱华丽), HU Guo-rong(胡国荣),
XIAO Jin(肖 劲), PENG Zhong-dong(彭忠东), LIU Ye-xiang(刘业翔)
(School of Metallurgical Science and Engineering,Central South University, Changsha 410083, China)
Abstract: Spinel LiMn2O4-xYx (Y=F, Cl, Br) compounds were prepared by solid-state reaction and the electrochemically galvanostatic charge-discharge cycles were performed using as-prepared compounds as cathode material. The influence of halogens on their lattice constants and the relation of electrochemical properties and their lattice constants were investigated. It is concluded that when the lattice constants are smaller than that of LiMn2O4, the reversible capacity fade is suppressed and the initial capacity sacrifice is observed. When the content of fluorine is 0.05, the lattice constant of LiMn2O3.95F0.05 is larger than that of LiMn2O4, the initial capacity is improved. An efficient method was found to control the lattice constants of LiMn2O4 through the addition of halogen, and to improve the electrochemical performance of LiMn2O4. The LiMn2O3.95F0.05 shows excellent electrochemical charge-discharge performance, with high initial capacity of 143mAh/g and nearly no capacity loss after 116 cycles.
Key words: spinel LiMn2O4-xYx; lattice constant; cathode materials; lithium-ion rechargeable battery; halogen CLC number: TM911.1
Document code: A
1 INTRODUCTION
Lithium-maganese-oxide compounds are the most promising candidates as cathode intercalation materials because of their nontoxicity, abundance and low cost[1-5]. They show extensive applications used as mobile power supplication for vehicles, rechargeable batteries for portable devices such as camcorder and laptop. But pure LiMn2O4 has some obvious disadvantages such as structural unstability and rapidly capacity fade, which can be included into three reasons[6-12]: crystal structure distortion induced by Mn3+ from cubic to tetrahedral structure, dissolution of manganese which causes the sacrifice of cathode mass, and the increase of resistance in electrode when oxidation of electrolyte takes place during the course of charge-discharge. Many efforts have been made to improve its electrochemical performance, such as cation substitution[13-19], anion substitution[ 20-22] and surface modification[23-26] of cathode materials and modification of electrolyte[27]. X-ray diffraction was used to investigate the influence of cation on lattice constants. But basically there was not systematical research on anion substitutions. Furthermore, most researches were basically limited to one sort of anion. In this work, we prepared spinel LiMn2O4-x-Yx (Y=F, Cl, Br) compounds, with the primary goals of evaluating their electrochemical behavior. In fact, it was expected that these additions have a key influence on their structure properties and electrochemical performances, which would provide a clue that anion should be select as a dopant. Moreover, we hoped to build up a correlation between the lattice constants and charge-discharge properties of spinel LiMn2O4-xYx (Y=F, Cl, Br). Finally, we also aimed to find an excellent performance cathode material compound for lithium-ion secondary battery.
2 EXPERIMENTAL
Spinel LiMn2O4-xYx (Y=F, Cl, Br) compounds were synthesized with electrolytic manganese dioxide, LiOH·H2O and LiY (Y=F, Cl, Br) by means of solid-state reaction. First, these mixtures were ball-milled in a planetary micro-mill with stainless steel balls. Alcohol, used as dispersing liquid, was added to form slurry, which was ground for 12h through combined shaking and rotation actions. Then, the fully mixed precursor slurry was dried to evaporate the alcohol under infrared lamp. Finally, the precursor was calcined in muffle at 730℃ for 36h and the products were obtained.
The positive electrode consisted of 80% as-prepared compounds and 15% acetylene black and 5% polytetrafluoroethylene (PTFE) as a binder, and metal Al was used as collector. The electrolyte solution was EC+DEC (1∶1)+1mol/L LiClO4. Lithium metal foil was used as the counter electrode during electrochemical measurements. All cells were assembled in an argon-filled glove box. The electrochemical tests were carried out in a DC-5 fully automatic program test instrument, with constant current (0.265-0.353mA/cm2, 3.0-4.35V).
X-ray powder diffraction (XRD) was carried out on a Rigaku D/max-rA with CuKα radiation and a graphite monochromator.
3 RESULTS AND DISCUSSION
3.1 Electrochemical results
The charge-discharge properties of spinel LiMn2O4-xYx (Y=F, Cl, Br) compounds were investigated in order to clarify the influence of these doped anions on their electrochemical performances. Meanwhile, for the same anion, we also found out the relation between the contents of dopant and performances of these compounds. The highest reversible capacities of LiMn2O4-xYx compounds are shown in Table 1. It is obvious that when fluorine was doped, the initial capacity was improved; but for the other halogens, it was just opposed to that. According to Kim and Manthlram[5], the anion with larger (ion) radius contributed to high initial capacity for amorphous lithium manganese oxyiodide. To spinel structure, it seemed not to be efficient. For the same halogen atom, it was observed that their initial capacities gradually decreased with the increase of contents of halogens except that it was not adaptable to LiMn2O4-xFx, whose initial capacity was improved at the beginning, and when the content of fluorine was continuously increased, they also exhibited the same tendency as other halogens did. In order to build up the correlation of the content of doped halogens with spinel structure stability, the influences of dopants on charge-discharge cycle life of spinel LiMn2O4-xYx (Y=F, Cl, Br) were also investigated. These relations are shown in Figs.1-3. For all the halogens, these dopants improved their cycle performances. Furthermore, with the increase of contents of halogens, their cycle behaviors were gradually improved with the initial capacity sacrifice, but there was an exception that LiMn2O3.95F0.05 compound exhibited excellent cycle stability with improved initial capacity. Its highest initial capacity reached 143mAh/g and nearly no capacity fade was observed after 116 cycles.
Table 1 Highest reversible capacity of spinel LiMn2O4-xYx (Y=F, Cl, Br) with different contents of halogens(mAh/g)
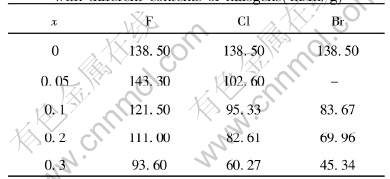
3.2 Powder X-ray diffraction
The XRD patterns of LiMn2O4-xYx (Y=F, Cl, Br) compounds are shown in Figs.4-6 and the lattice constants shown in Table 2 were calculated according to the data of powder X-ray diffraction. We find that almost all the peaks are attributed to the spinel structure. From Table 2, it can be concluded that when fluorine was doped, the lattice constants became larger. However, other halogen dopants caused the decrease in lattice constants. And for the same dopant element, the fluorine led to the increase in lattice constants with the increase of contents; while the other halogens such as Cl and Br caused the decrease in the lattice constants.
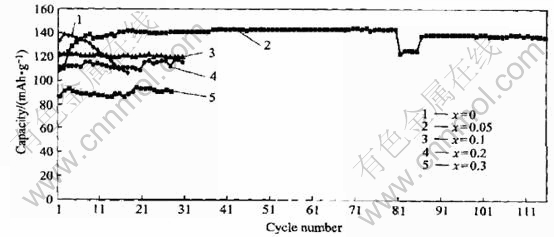
Fig.1 Relation of reversible capacity and charge-discharge cycles of spinel LiMn2O4-xFx
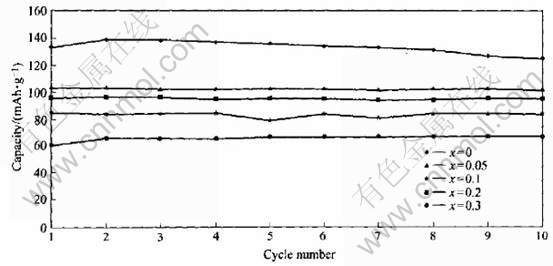
Fig.2 Relation of reversible capacity and charge-discharge cycles of spinel LiMn2O4-xClx
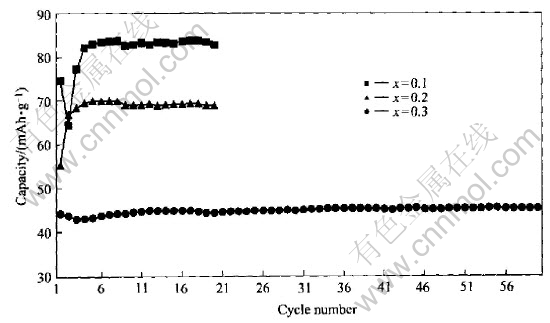
Fig.3 Relation of reversible capacity and charge-discharge cycles of spinel LiMn2O4-xBrx
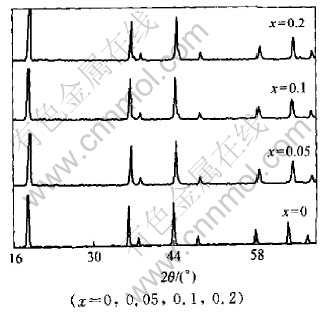
Fig.4 XRD patterns of LiMn2O4-xFx
3.3 Electrochemical properties and structure character
According to above results, we can efficiently correlate the electrochemical property and struc-
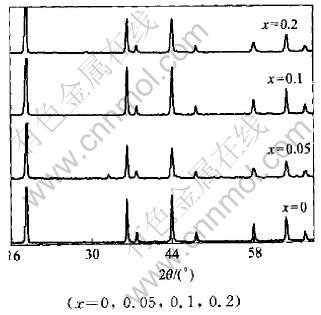
Fig.5 XRD patterns of LiMn2O4-xClx
ture character. It is obvious that the initial capacity was gradually changed with the change of lattice constant. When the lattice constants became larger, the initial capacities increased and when the
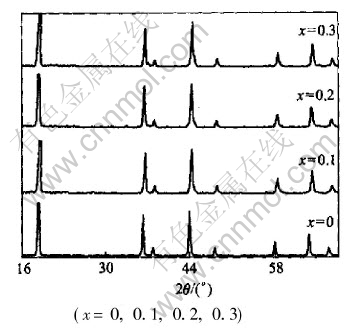
Fig.6 XRD patterns of LiMn2O4-xBrx
Table 2 Influences of halogen atoms on lattice constant of spinel LiMn2O4-xYx(Y=F, Cl, Br)(ù)
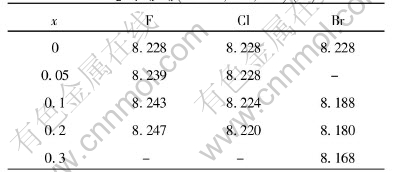
lattice constants decreased, the initial capacities also decreased. But this rule was not always adaptable to LiMn2O4-xFx compound. The lattice constant gradually became larger and its initial capacity was also higher than LiMn2O4 when x was 0.05. However, its initial capacity began to drop when the content of fluorine continued to increase. So we seemed to reach a conclusion that there was a limited lattice constant, beyond which we could not any more improve the initial capacity of spinel LiMn2O4-xYx by means of controlling the lattice constants through the dopants of halogens, although under which it was an efficient method to improve the initial capacity. When the lattice constant became smaller, the capacity fade was gradually lowered. The decrease of lattice constants contributed to suppress the capacity fade, but too smaller lattice constant sacrificed too much reversible capacity. Finally, it can be concluded that it is an efficient method to improve the initial capacity and suppress the capacity fade through controlling the lattice constant making use of the dopants of halogens.
4 CONCLUSION
The spinel LiMn2O4-xYx (Y=F, Cl, Br) compounds were synthesized by solid-state reaction. It was found that the dopant of halogens has significant influence on the lattice constants of these compounds. Furthermore, a rule was observed that the initial capacity could be improved and the capacity fade could be suppressed by controlling the lattice constant. In addition, a good cathode material LiMn2O3.95F0.05 compound was found. The LiMn2O3.95F0.05 showed excellent electrochemical charge-discharge performance, with high initial capacity of 143mAh/g and nearly no capacity loss after 116 cycles.
REFERENCES
[1]Tarascon J M, Guyonard D. The Li1+xMn2O4/C rocking-chair system: a review[J]. Electrochemical Acta 1993, 38(9): 1221-1231.
[2]Tarascon J M, Wang E, Shokoohi F K. The spinel phase of LiMn2O4 as a cathode in secondary lithium cells[J]. J Electrochem Soc, 1991, 138: 2859-2863.
[3]Yamada A, Miura K, Hinokuma K, et al. Synthesis and structural aspects of LiMn2O4±δ as a cathode for rechargeable lithium batteries[J]. J Electrochem Soc, 1995, 142: 2149-2155.
[4]Lee Y J, Wang F, Grey C P. 6Li and 7Li MAS NMR studies of lithium manganese cathode materials[J]. J Am Chem Soc, 1998, 120: 12601-12613.
[5]Kim J, Manthlram A. A manganese oxyiodide cathode for rechargeable lithium batteries[J]. Nature, 1997, 390: 265-267.
[6]Sanchez L, Tirado J L. Synthesis and electrochemical characterization of a new Li-Co-Mn-O spinel phase for rechargeable lithium batteries[J]. J Electrochem Soc, 1997, 144: 1939-1943.
[7]Robertson A D, Lu S H, Averill W F, et al. M3+-modified LiMn2O4 spinel intercalation cathodes 1.admetal effects on morphology and electrochemical performance[J]. J Electrochem Soc, 1997, 144(10): 3500-3504.
[8]Blyr A, Amatucci C, Guyomard D, et al. Self-discharge of LiMn2O4/C Li-ion cells in their discharge state[J]. J Electrochem Soc, 1998, 145(1): 194-209.
[9]Jang D H, Shin Y J, Oh S M. Dissolution of spinel oxides and capacity loses in 4 V Li/LixMn2O4 cells[J]. J Electrochem Soc, 1996, 143(7): 2204-2210.
[10]Xia Y Y, Zhou Y H, Yoshio M. Capacity fading on cycling of 4 V Li/LiMn2O4 cells[J]. J Electrochem Soc, 1997, 144: 2593-2600.
[11]Jang D H, Oh S M. Electrolyte effects on spinel dissolution and cathodic capacity losses in 4 V Li/LixMn2O4 rechargeable cells[J]. J Electrochem Soc, 1997, 144(10): 3342-3347.
[12]Pasquier A D, Blyr A, Courjal P, et al. Mechanism for limited 55℃ storage performance of Li1.05Mn1.95- O4 electrodes[J]. J Electrochem Soc, 1999, 146(2): 428-436.
[13]Arora P, Popov B N, White R E. Electrochemical investigations of cobalt-doped LiMn2O4 as cathode material for lithium-ion batteries[J]. J Electrochem Soc, 1998, 145(3): 807-814.
[14]Robertson A D, Lu S H, Howard W F. M3+-modified LiMn2O4 spinel intercalation cathodes Ⅱ.electrochemical stability by Cr3+[J]. J Electrochem Soc, 1997, 144(10): 3505-3512.
[15]Hayashi N, Ikuta H, Wakihara M. Cathode of LiMgyMn2-yO4 and LiMgyMn2-yO4-δspinel phases for lithium secondary batteries[J]. J Electrochem Soc, 1999, 146(4): 1351-1354.
[16]Amatucci G G, Ereira N P, Zheng T, et al. Enhancement of the electrochemical properties of LiMnO4 through chemical substitution[J]. J Power Sources, 1999, 81-82: 39-43.
[17]Haitao H, Vincent C A, Bruce P G. Correlating capacity loss of stoichiometric and nonstoichiometric lithium manganese oxide spinel electrodes with their structural intergrity [J]. J Electrochem Soc, 1999, 146(10): 3649-3654.
[18]Takashi O, Nobuo O, Keiichi K, et al. Electrochemical properties of spherical porous LiMn2-xMgxO4 powder prepared by ultrasonic spray pyrolysis [J]. Electrochemistry, 2000, 68: 162. (in Japanese)
[19]Liu W, Kowal K, Farrington G C. Electrochemical characteristic of spinel phase LiMn2O4-based cathode materials prepared by the pechini process. Influence of firing temperature and dopants [J]. J Electrochem Soc,1996, 143: 3590-3596.
[20]Xia Y J, Hadeshima Y, Kumada N, et al. Studies on Li-Mn-O spinel system(obtained from melt-impregnation method) as a cathode for 4 V lithium batteries(part Ⅴ): Enhancement of the elevated temperature performance of Li/LiMn2O4 cells[J]. J Power Sources, 1998, 74: 24-28.
[21]Palacin M R, Cras F Le, Seguin L, et al. In situ structural study of 4V-range lithium extraction/insertion in fluorine-substituted LiMn2O4[J]. J Solid State Chemistry, 1999, 144: 361-371.
[22]Amatucci G G. Lithium Manganese Oxy-fluorides for Li-ion Rechargeable Battery Electrodes[P]. US 5674645, 1997.
[23]Amatucci G G, Tarascon J M. Rechargeable Battery Cell Having Surface-treated Lithiated Intercalation Positive Electrode[P]. US 5705291, 1998.
[24]Amatucci G G, Blyr A, Tarascon J M. Chelation Treatment for Reduced Self-discharge in Li-ion Batteries[P]. US 5695887, 1997.
[25]Gnanaraj J S, Pol V G, Gedanken A G, et al. Improving the high-temperature performance of LiMn2O4 spinel electrodes by coating the active mass with MgO via a sonochemical method[J]. Electrochemistry Communications, 2003, 5: 940-945.
[26]Lee S W, Kim K S, Moon H S, et al. Electrochemical characteristics of Al2O3-coated lithium manganese spinel as a cathode material for a lithium secondary battery[J]. J Power Sources, 2004, 126: 150-155.
[27]Hamamoto, Toshikazu, Hitaka, et al. Electrolyte Solution for Lithium Secondary Battery[P]. US 6045945, 2000.
(Edited by YUAN Sai-qian)
Foundation item: Project (02JJY2081) supported by the Natural Science Foundation of Hunan Province
Received date: 2004-04-30; Accepted date: 2004-09-13
Correspondence: CHEN Zhao-yong, Associate Professor, PhD; Tel: +86-731-8876454; E-mail: chenzh@mail.csu.edu.cn