
A novel chain-extended urea containing hyperbranched polymer used as toughening modifier for epoxy resin
LU Shao-rong(陆绍荣), WEI Chun(韦 春), YANG Xiao-wang(杨小王)
Key Laboratory of Nonferrous Metal Materials and New Processing Technology Ministry of Education,
Guilin University of Technology, Guilin 541004, China
Received 10 April 2006; accepted 25 April 2006
Abstract: A new kind of reactive toughening accelerator for epoxy resin, amine-teminated hyperbranched polymer (H2O-NMe2) was synthesized and characterized by FT-IR spectroscopy. Dynamic mechanical analysis (DMA) was used to study the glass transition temperature (Tg), loss factor (tanδ) and activation energy (Ea) by using multiplexing frequency. The results show that the Ea at glassy relaxation process of modified system is about 70-80 kJ/mol higher than that of unmodified system, and the high modulus and good thermal properties are still maintained.
Key words: epoxy resin; hyperbranched polymer; multiplexing frequency mode; toughening; chain-extended urea
1 Introduction
Epoxy resins are thermosets combining attractive properties, such as high strength and stiffness and excellent dimensional, thermal and environmental stabilities. Epoxy-based materials are being applied as matrix resins of coatings, adhesives and composites. The well-known drawback associated with the application of highly cross-linked thermosetting polymer is related to their inherent brittleness,which increases with cross-link density [1]. In order to circumvent this toughness problem, impact modifiers are added to epoxy resins in order to produce multiphase blends with improved toughness/stiffness balance. Two types of impact modifiers are established: epoxy flexibilizers and epoxy toughening agents. Both are miscible with the uncured epoxy resin and should not deteriorate the low resin viscosity required to afford easy processing. In contrast to flexibilizers, toughening agents improve toughness without sacrificing stiffness and glass transition temperature. Prominent modifiers are based upon liquid rubbers such as carboxyl-terminated butadiene-acrilonitrile liquid rubber (CTBN) or amine-terminated butadiene- acrilonitrile liquid rubber (ATBN) [2,3]. All the approaches mentioned above have been achieved by improving in toughness.
Recently, new types of impact modifiers based upon hyperbranched polymers (HBPs), especially polyesters, have been introduced to improve toughness[4-8]. Hyperbranched hydroxy-, carboxyl- and epoxy-terminated polyesters give low viscosity blends. A few percent of such polyesters are sufficient to significantly improve toughess without sacrificing stiffness and glass temperature [9,10]. CLAESSON et al[11] used hyperbranched aliphatic polyesters as curing agents in the coating resin formula. The resin could be UV cured and the glass transition temperature of the cured films was correlated to the difference in the structure of terminal groups in the resin. M?NSON et al and later BOOGH et al[9,10] reported independently on the successful toughening of epoxy resins by epoxy-functional Bolton. According to these works, the stress intensity factor, KIc, values of the prepared resins were improved up to 300%, depending on the degree of modification of the HBP and the curing cycle. WEI et al[12] and JOHANSSON et al[13] and WAN et al[14] also studied hyperbranched methacrylated polyesters and their use in photo-polymerization of films and fiber-reinforced polymer composites. The resins exhibited low viscosities and short curing times.
Although the use of HBPs as curing agents has somewhat been carried out, there is very limited information concerning the cure kinetics and the cure echanism in the system. Therefore, this paper reports the synthesis of a new kind of chain-extended urea (H2O-NMe2 ) and the use of this new compound to modify the DDS-cured epoxy resin system. The dynamic mechanical properties, mechanical properties, as well as morphology of the fractured surfaces of the modified systems were investigated.
2 Experimental
2.1 Materials and measurements
BoltonTM20(H2O) provided by Perstorp AB company (Sweden) and used directly. Epoxy resin (diglycidyl ether of bisphenol A DGEBA (E-51), purchased from Yueyang Chemical Plant, China), Toluene 2,4-diisocyanate (TDI) ( 80% 2,4-mixture and 20% 2,6-mixture ) was purified by distillation under vacuum, 4,4′-diaminodiphenylsulphone(DDS) purchased from Shanghai Chemical Reagent Company, China. Dimethylamine was chemical agent, and distillated before use.
Fourier transformed infrared spectroscopy (FT-IR) was recorded on a Perkin-Elmer 1710 spectro- photomerter using KBr pellets at room temperature. The impact strength was measured on a tester of type XJJ-5, which is with no notch in the specimen according to china national standard GB1043-79. Tensile measurements were carried out on a tester of type RGT-5 (REGER instrument company, Shenzhen, China). Testing machine according to china national standard GB1040-92. Thermo-mechanical properties, modulus, and glass transition temperatures were determined using a linear rheometer (Rheometrics 902-50010 dynamic mechanical analyzer, was made with a TA instruments) in a three point bending, at a frequency of 0.1, 1, 5, 10 and 20 Hz from -120 ℃ to 250 ℃ at a heating rate of 5 ℃/min, respectively.
2.2 Preparation of hyperbranched chain-extended urea (H2O-NMe2)
Into a three-necked round-bottomed flask, equipped with a stirrer, an N2 inlet and a cooler 5.36 g (3.07 mmol) H2O dissolved in proper amount of acetone was added. Then the mixture of 7.0 mL(49.12 mmol) TDI and 10 mL acetone were dropped into the flask within 1 h. The solution was stirred for additional 20 h at 30 ℃, and the yellow solution was obtained. Then 3.22 mL(49.12 mmol) dimethylamine was dropped into above solution within 3 h, stirred at 30 ℃ for additional 1 d. The mixture solution was under vacuum at 50 ℃ to get rid of acetone and the chain-extended urea (H2O -NMe2) was obtained. Its chemical structure is shown in Fig.1.
2.3 Curing procedure
A mixture of epoxy (E-51) resin , DDS and H2O―NMe2 was degassed in vacuum at 100 ℃ for about 30 min. The resulting mixture was then cast into a preheat mold coated with silicone resin. All samples were cured at 120 ℃ for 2 h, 160 ℃ for 2 h and 180 ℃ for 2 h. All formulations were made by approximately 100 parts by weight epoxy resin with different parts of modifier and curing agent. Since all formulations were based on 100 parts of epoxy resin, the modifier or curing agent will be referred to on parts per hundred epoxy resin.
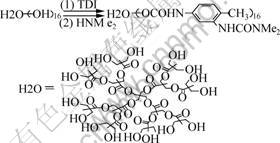
Fig.1 Preparation of chain-extended urea
3 Results and discussion
3.1 Characterization of step products
As mentioned in section 2, preparation of hyperbranched chain-extended urea(H2O-NMe2) included two steps. The possible reactions are shown in Fig.1.
Step1 is hydrogen shift reaction between Tolylene2,4-diisocyanate(TDI) and H2O, resulting in urethane coupling between their molecules and endcapped H2O as the product. Since the molar ratio of them was 16:1, so mainly the contrapuntal —NCO groups on TDI molecules took part in the reaction, with the neighboring groups remaining unreacted. Step 2 is a terminating reaction of dimethylamine with the endcapped H2O, in this step, HN== groups of dimethylamine reacted with remaining —NCO groups of endcapped H2O, forming carbamido linkage. The product of this step H2O -NMe2 contains —HN— groups on its molecules, so it can react with the epoxide group of epoxy resin and accelerate the curing reaction procedure of epoxy resin.
The products in every steps and curing reaction were characterized with FT-IR. Fig.2 shows the FT-IR spectra of H2O(a), endcapped H2O(b), H2O-NMe2(c) and H2O-NMe2 (d) modified epoxy. In curve (a) a distinct absorption peak at about 1 100 cm-1 is in the region of —C—O—C stretching for ether groups and the peak at 3 474 cm-1 belongs to —OH group. In curve (b) the peak at about 2 272 cm-1 confirms the existence of —NCO groups. In curve (c) the peak at about 2 270 cm-1 disappears, while the peak at about 3 324 cm-1 belongs to —NH— groups. Absorption bands at about 1 728 cm-1 are mainly due to —C==O asymmetric stretching peak from urethane groups. In curve (d) duple peaks appear, the peak at 3 474 cm-1 belongs to —OH group, which generates from epoxy and the ring opening of epoxy resin, and 3 340 cm-1 belongs to —NH— group.
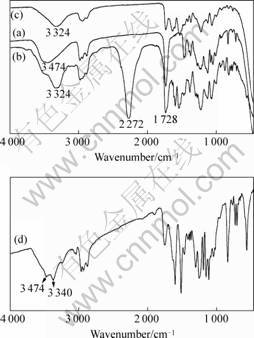
Fig.2 Spectra of H2O(a), endcapped H2O(b), H2O-NMe2 (c) and H2O-NMe2 modified epoxy(d)
3.2 Mechanical properties
The most common approach used to enhance the toughness of epoxy resins is to add a softer second phase to epoxy resin[15]. The dispersed rubber phase plays an important role in the toughness improvement of the material. Rubber particles, by acting stress concentrators,
allow the matrix between the particles to undergo both shear and craze deformation. In this study, the chain-extended urea with HBPs acts as the softer phase. The effect of H2O-NMe2 modification on the mechanical strength is listed in Table 1. The addition of H2O-NMe2 only leads to a small improvement in impact strength until the content of H2O-NMe2 is up to 6% and significant improvement in impact strength was found at higher content (18%). The blend containing 18% H2O -NMe2 shows very high impact strength (24.6 kJ/m2), which is almost 2-3 times higher than that of unmodified system (11.5 kJ/m2). The impact behavior of the modified epoxy networks can be explained by SEMs in terms of the morphological observation. From the photograph (Fig.3(a)), one can be seen that the fractural surface for unmodified system is quite different from that of the modified systems, it is very smooth and with uniform crack direction, and reveals the characteristics of brittle fracture and accounts for its poor impact strength. As for the modified systems as shown in Figs.3(b)-(d), which indicate tough fracture. The fracture surface in Fig.3(b) shows branches and appears rougher than that of the unmodified system, its impact strength gets considerable improvement correspondingly. Fracture surfaces of curves (c) and (d) present large and deep cavities, which are generally the characteristics of rubber-modified epoxy resins. These cavities represent the initial position of the rubber particles, which were pulled out or broken during the fracture process. These cavities are firmly attached to the epoxy matrix, resulting in a good adhesion and a strong interface between the H2O-NMe2 and epoxy matrix phase. A mechanism, proposed by RAYMOND et al[16], and reported by PEARSON and YEE[17], seems to fit relatively well with the results of the present study which suggests that the fracture resistance increases, arising from a great extent of energy-dissipating deformation occurring in the material in the vicinity of the crack tip. Such results explain the increase in the epoxy roughness, and the most probable mechanism is based on the internal cavitation of the H2O-NMe2 particles, which like the rubber particles, followed by localized plastic deforma
Table 1 Influence of amount of H2O-NMe2 on mechanical properties
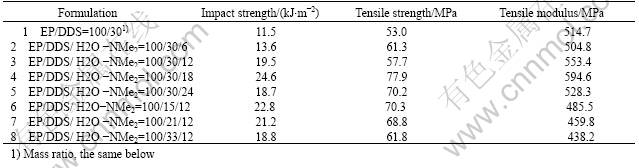
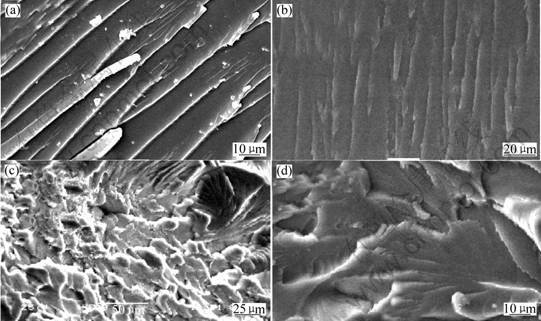
Fig.3 SEM images of fractured surface of curing samples: (a) EP/DDS=100/30; (b) EP/DDS/H2O-NMe2=100/30/6; (c) EP/DDS/H2O-NMe2 = 100 /30/18; (d) EP/ DDS/ H2O-NMe2=100/30/24
tion. The result shows that branches and cavitiesappearance on the fracture surface played an important role in improving impact strength.
Table 1 shows the tensile strength and modulus for the modified systems and unmodified system. The results demonstrate that the reinforcement effect reaches a maximum at 18% H2O-NMe2. A slightly higher effect of H2O-NMe2 treatment on the mechanical properties without treatment was expected, because the H2O-NMe2 treatment introduces a higher adhesive surface with epoxy resin. However, the tensile modulus results showed no significant difference for both materials and were possible due to the introduction of HBPs soft segments into the epoxy matrix. When H2O-NMe2 was introduced in a ratio larger than 18% into the EP/H2O-NMe2 co-polymer system, the mechanical strength and modulus decrease due to the introduction of more HBPs soft segments.
3.3. Dynamic mechanical behavior
The dynamic mechanical properties between -120 and 150 ℃ for the modified curing systems were investigated by using a Rheovibron dynamic viscoelastomer at a heating rate of 5 ℃/min and a frequency of 1 Hz.
Fig.4 shows the elastic modulus of the curing system modified by H2O-NMe2. It can be seen that below 100 ℃, the elastic modulus of the modified curing systems was approximate to that of the unmodified sample. As we know when rubbery components are used to modify the epoxy resin, modulus is usually sacrificed at elevated temperature. However, due to the novel H2O-NMe2 toughness agents synthesized in this work containing not only the hyperbranched polymer but also rigid units (benzene). The HBPs is aimed to improve impact strength, and the rigid units are intended to retain high dynamic modulus and thermal properties of the cured resin. It is the rigid units that play a great role in maintaining the high modulus properties of the cured epoxy resin or enhanced the high dynamic modulus of epoxy resin modified by the H2O-NMe2 toughness agents.
Fig.5 shows the tan δ plots of four different H2O-NMe2 content material specimens as function of
temperature. It was observed that Tg of all the four specimens increases with respect to increasing frequency. It can be seen from Fig.4 that all spectra of cured
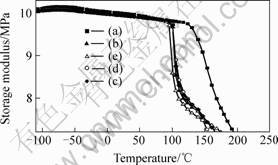
Fig.4 Modulus vs. temperature for EP/DDS/H2O-NMe2 curing systems: (a) EP/DDS=100/30; (b) EP/DDS/ H2O-NMe2= 100/30/6; (c) EP/DDS/ H2O-NMe2 =100 / 30 /12; (d) EP/DDS/ H2O-NMe2=100/30/18; (e) EP/DDS/ H2O-NMe2=100/30/24
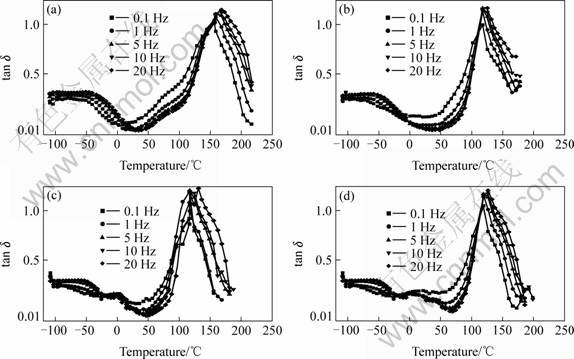
Fig.5 Plots of tanδ versus temperature of specimens: (a) EP/DDS=100/30; (b) EP/DDS/ H2O-NMe2=100/30/6; (c) EP/DDS/H2O-NMe2=100/30/12; (d) EP/DDS/H2O-NMe2 =100/30/18
systems exhibit α-transition andβ-relaxation peak. Compared with the unmodified system, the temperature range of β-relaxation for the modified system became broader and the position of β-relaxation gradually shifted to lower temperature. The β-relaxation was the contribution of the hydroxyether group [—CH2(CHOH)-CH2O—] in the amine–cured epoxy resins network. However, β-relaxation of the modified systems shifted to a lower temperature, which may be the contribution of the softer segment in HBPs. The Besides α-transition, there appears shoulder peak α-relaxation at around 30 ℃ and 0 ℃ of the tanδ curve in Figs.5(c) and (d). From the above results it can be inferred that H2O-NMe2 the toughening agent has been incorporated into the tight epoxy resin network and led to the formation of a two-phase microstructure in the modified systems, which contributed to the improvement of impact strength. It could also be confirmed further by impact and SEM observation.
3.4 Activation energy of Tg relaxation of process of curing system
A typical Arrhenius plot, logf versus 1/Tg absolute temperature yields an activation energy (Ea) for the Tg relaxation process. Activation energies of four epoxy materials were calculated from the slopes -Ea/R of each plot. Fig.6 is the Arrhenius plot of logf versus (1/Tg) of specimens containing 0, 6%, 12 %, 18% H2O-NMe2, respectively, yielding activation energies ( Ea ) of 191.7, 266.8, 278.7, 260.0 kJ/mol for the glassy relaxation processes. Coefficients of regression (R) for four specimen are all larger than 0.93. The ( Ea ) of the modified system were higher than the ( Ea ) of the unmodified system. It indicates the interaction between H2O-NMe2 and matrix, which greatly impede the chain mobility in epoxy resin. It is, therefore, observed that (Ea)of the modified system is about 1.4 times of that unmodified system.
4 Conclusions
1) The linear relationship between log f and 1/Tg was observed and glassy relaxation activation energy of the specimen with different H2O-NMe2 content was calculated. The specimen with 18% H2O-NMe2 has the Ea value about 1.4 times higher than that of the unmodified system.
2) The H2O-NMe2 can act as an effective toughening modifier for the epoxy resin. The impact strength of the cured system modified with H2O-NMe2 is two times higher than that of the unmodified system,
while the high modulus and good thermal properties are
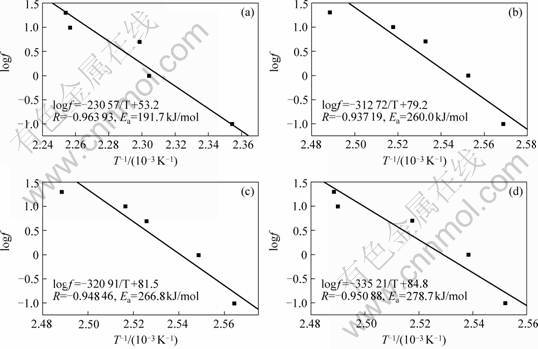
Fig.6 Arrhenius plots of specimens EP/DDS/=100/30(a), EP/DDS/H2O-NMe2=100/30/6(b), EP/DDS/H2O-NMe2=100/30/12(c) and EP/DDS/H2O-NMe2=100/30/18(d)
maintained. References
[1] MAY C A. Epoxy resins[M]. 2nd ed. New York: Marcel Dekker, 1988.
[2] PEARSON R A, YEE A F. Toughening mechanisms in Elastomer-modified epoxies[J]. J Mater Sci , 1989, 24: 2571-2580.
[3] KUNZ S C, SAYRE J A, ASSINK R A. Morphology and toughess characterization of epoxy resins modified with amine and carboxyl terminated rubbers[K]. Polymer, 1982, 23: 1897-1905.
[4] SIDORENKO A, ZHAI X W, SIMON E, PLEUL D, TSUKRUK V V. Hyperbranched molecules with epoxy-functionalized terminal branches:grafting to a solid surface[J]. Macromolecules, 2002, 35: 5131 -5139.
[5] MEZZENGA R, BOOGH L, JAN-ANDERS E. A review of dendritic hyperbranched polymer as modifiers in epoxy composites[J]. Composites Science and Technology, 2001, 61: 787-795.
[6] RATNA D, SIMON G P. Thermomechanical properties and morphology of blends of a hydroxy-functionalized polymer and epoxy resin[J]. Polymer, 2001, 42: 8833-8839.
[7] OH J K, JANG J, LEE S H.Curing behavior of tetrafunctional epoxy resin/hyperbranched polymer system[J]. Polymer, 2001, 42: 8339-8347.
[8] MEZZENGA R, PETTERSSON B, M?NSON J A E. Evaluation of solubility parameters during polymers polymerization of amine-cured epoxy resins[J]. J Polym Sci B, 2000, 38: 1883-1891.
[9] M?NSON J A E, PETTERSSON B, BOOGH L. Dendritic hyperbranched polymers as tougheners for epoxy resins[J]. Polymer, 1999, 40: 2249-2261.
[10] BOOGH L, PETTERSSON B, JAPON S, M?NSON J A E. Proceedings of the ICCM-10 Conf[J]. Whistler, 1995, 4: 389.
[11] CLAESSON H, MALMSTR?M E, JOHANSSON M, HULT A, M?NSON J A E. Rheological behaviour during UV-curing of a star-branched polyester[J]. Prog Org Coat, 2002, 44: 63-67
[12] WEI H, LU Y, SHI W, YUAN H, CHEN Y. UV curing behavior of methacrylated hyperbranched poly(amine-ester)s[J]. J Appl Polym Sci, 2001, 80: 51-57.
[13] JOHANSSON M, GLAUSER T, ROSPO G, HULT A. Radiation curing of hyperbranched polyester resins[J]. J Appl Polym Sci, 2000, 75: 612-618.
[14] WAN Q, SCHRICKER S R, CULBERTSON B M. Methacryloyl derivitized hyperbranched. 2.Photo-polymerization and properties for dental resins system[J]. J Macromol Sci,Pure Appl Chem, 2000, A37(11): 1317-1331.
[15] HE Shang-jin, SHI Ke-yu, BAI Jie, et al. Studieson the properties of epoxy resins modified with chain-extended ureas[J]. Polymer, 2001, 42: 9641-9647.
[16] PEARSON R A, ALBENT F. Toughening mechanismin thermoplastic modified epoxies: (1) Modification using poly(phenylene oxide)[J]. Polymer, 1993, 34: 36589-36604.
[17] PEARSON R A, YEE A F. Toughening mechanism in elastomer-modified epoxy resins microscopy studies[J]. J Mater Sci, 1986, 21: 2475-2482.
(Edited by LONG Huai-zhong)
Foundation item: Project (0447053) supported by the Natural Science Foundation of Guangxi Province, China; Project(50473060) supported by the National Natural Science Foundation of China; Project ([2004]20) supported by the Department of Education of Gunangxi Province, China
Corresponding author: LU Shao-rong; Tel: +86-773-5896438; Fax: +86-773-5896671; E-mail: gllushaorong@glite.edu.cn