Article ID: 1003-6326(2005)03-0619-07
Corrosion electrochemical behavior of brass tubes in circulating cooling seawater
LU Yu-zhuo(卢玉琢)1, SONG Shi-zhe(宋诗哲)1,2, YIN Li-hui(尹立辉)1
(1. School of Materials Science and Engineering, Tianjin University,
Tianjin 300072, China;
2. State Key Laboratory for Corrosion and Protection of Metals, Shenyang 110016, China)
Abstract: Electrochemical impedance spectroscopy(EIS) and electrochemical noise(EN) were used to study the corrosion electrochemical behavior of brass tubes in circulating cooling seawater using the developed sensor. EIS study shows that the inhibitor can lead to the formation of corrosion products on metal surface, which will then inhibit the corrosion process. When the flow rate of the seawater increases, the diffusion of oxygen speeds up and the action of filming on HAl77-2 tube accelerates, resulting in decrease of corrosion rate. EN analysis shows that the flow rate of the seawater has little effect on pitting susceptivity of HSn70-1 tube; however the pitting susceptivity of HAl77-2 tube increases with increasing flow rate. Good agreement is observed between the spectral noise resistance Rsn(f) calculated from EN data and the modulus of impedance. It is shown that the electrochemical noise technique can be used in corrosion monitoring.
Key words: brass tube; seawater; electrochemical impedance spectroscopy; electrochemical noise CLC
number: TG174 Document code: A
1 INTRODUCTION
Copper and its alloys possess excellent properties, which helps them to be widely applied in the fields of architecture, naval construction, electricity, petrochemical engineering and many other fields of great importance. The copper-based alloy in which zinc behaves as the main additional element is called brass. Brass tubes are often used as heat exchanger, in which fouling products are inclined to come into being and microbe is ready to grow[1, 2], thereby the tubes will fail by reason of perforation[3]. Up to now no efficient ways have been found to monitor the corrosion of brass tubes in flowing medium.
Electrochemical impedance spectroscopy(EIS) technique is very useful for evaluation of inhibitor performances, analysis of electrochemical mechanisms and corrosion processes. Equivalent circuit models provide a helpful way to interpret and quantify the EIS spectra if the physical models are understood[4]. So EIS is considered a well-established technique in corrosion research, which has been applied successfully in studies of many corrosion systems[5].
The potential and current spontaneous fluctuations occurring on metal electrodes immersed in aqueous solutions, have been extensively studied in the last twenty years. It has been demonstrated that these fluctuations, known as electrochemical noise(EN), can provide information about non-equilibrium systems of complex electrochemical reactions[6]. And the electrochemical noise analysis has become a very promising tool in localized corrosion studies[7].
In this paper, a sensor, with the diameter less than 1mm, to monitor the corrosion in the tubal metal, was developed[8], which is composed of Ag/AgCl reference electrode and Pt/Ir alloy assistant electrode. Using the sensor developed, the corrosion electrochemical behavior of brass tubes in circulating cooling system was studied.
2 EXPERIMENTAL
2.1 Experimental system
The circulating cooling system was designed and manufactured by Chemical Engineering Center of Heat Engineering Research Institute, State Power Company, China. It is composed of hot water case, cooling tower, water tank, and seawater pump. There were altogether six brass tubes in the circulating system, which were placed in the hot water case, arrayed in three layers. The structure is described elsewhere in detail[9].The sensors were placed in HSn70-1 and HAl77-2 tube respectively in the inferior layer and the compositions of the tubes are listed in Table 1. The cooling seawater is taken from Tanggu of Tianjin, China, where inhibitor was added thereafter to help the tubes to form protecting film in advance. The concentrating ratio of the seawater is in the range of 2.0-2.2. When the system was operated stably, the flow rate in the tube was 0.6m/s.
Table 1 Compositions of HSn70-1 and HAl77-2 tubes(mass fraction, %)
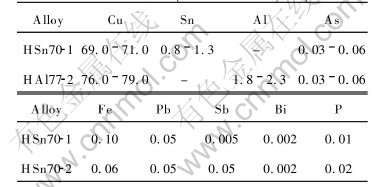
Outside the tubes the circulating distilled water was heated to 60℃. The sensor in HSn70-1 tube was placed along the flow, with the distance from the tube mouth of about 20cm and the water temperature of 35℃; while the sensor in HAl77-2 tube was placed against the flow, with the distance from the tube mouth of 20cm and the water temperature of 45℃. The experiment lasted for 17d. From the 4th day on, measurements were also conducted when the system ran stably at the flow rate of 1.2m/s and 1.8m/s respectively.
Some synchronizing tests were carried out on pre-filmed, non-filmed HAl77-2 and HSn70-1 specimens, with the exposed area of 1cm2, for contrast.
2.2 Experimental method
Electrochemical noise of brass tubes was recorded at φcorr. EIS measurements on brass tubes at φcorr were made with an applied sinusoidal voltage signal of 10mV over a frequency range of 20kHz to 20mHz, with the frequency sweep of eight steps in each decade.
Potentiodynamic test was also made on brass tubes, with the scanning velocity of 0.2mV/s, in the range of φcorr=±30mV.
In all the measurements made above, PC4/300 electrochemical system of GamryTM was used with a conventional three-electrode configuration applied with Ag/AgCl reference electrode and Pt/Ir alloy assistant electrode.
In the synchronizing experiments, PARSTAT2263, Advanced Electrochemical System of Princeton Applied ResearchTM was used to conduct EN measurements of specimens using zero resistance ammeter(ZRA) mode, with a saturated calomel electrode(SCE) used as reference electrode.
3 RESULTS AND DISCUSSION
3.1 EIS analysis
3.1.1 EIS features of HAl77-2 specimens
Electrochemical impedance spectra of HAl77-2 specimens immersed for 4d are shown in Fig.1. Nyquist plot of non-filmed specimen in natural seawater presents a single capacitive impedance arc, with one time constant, and the corresponding electrochemical equivalent circuit is shown in Fig.2(a). Nyquist plots of non-filmed and pre-filmed specimens in condensed seawater both exhibit a feature of two capacitive impedance arcs, with two
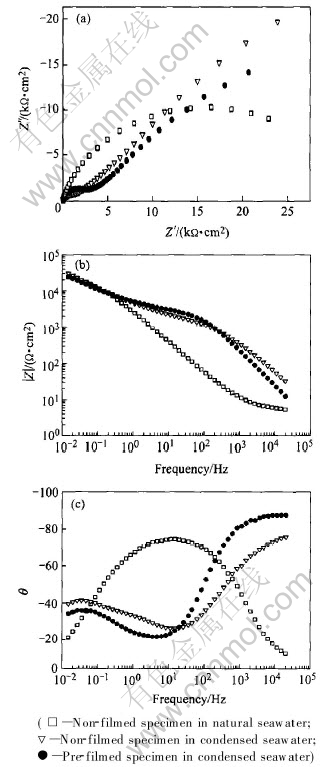
Fig.1 Electrochemical impedance spectroscopy of HAl77-2 specimens immersed for 4d
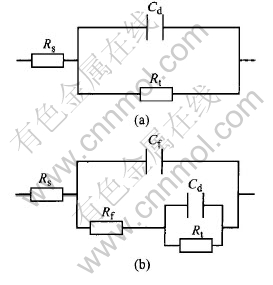
Fig.2 Electrochemical equivalent circuits of Nyquist plots for non-filmed specimen(a) and pre-filmed specimen(b)
time constants. The capacitive impedance arc at high frequency of Nyquist plot of the non-filmed specimen in condensed seawater indicates that the inhibitor can form protective film on the surface of the specimen beforehand, thereby restrain the corrosion process. The capacitive impedance arcs at high frequency in the Nyquist plot of the pre-filmed specimen are more evident, which shows that the corrosion resistance of the pre-filmed specimen is enhanced significantly.
The electrochemical equivalent circuit of Nyquist plot for pre-filmed specimen is shown in Fig.2(b), in which Rs, Cd, Rt, Cf, and Rf present solution resistance, capacitance of double layer, electrochemical reaction resistance, film capacitance, and film resistance respectively.
3.1.2 EIS features of brass tubes in circulating cooling seawater
Fig.3 represents the electrochemical impedance
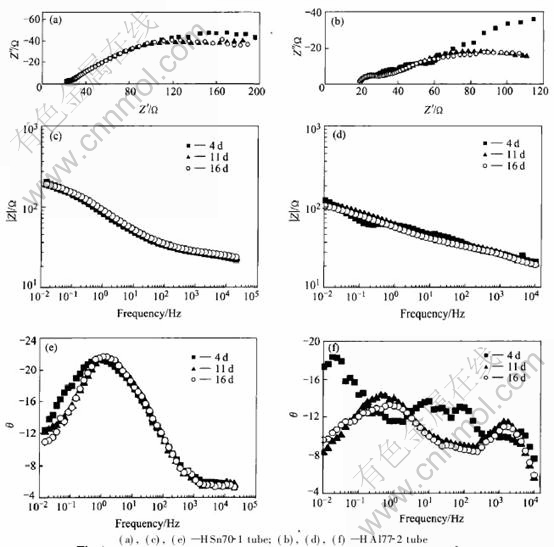
Fig.3 Electrochemical impedance spectroscopy of brass tubes after immersed for different periods of time
spectra of brass tubes at flow rate of 0.6m/s after immersed for different period of time and they all have two time constants. The corresponding electrochemical equivalent circuit is also given in Fig.2(b). It is obvious that there is no apparent variation in the impedance modulus of HSn70-1 and HAl77-2 tubes.
The electrochemical impedance spectra of brass tubes at different flow rates after immersed for 4d are shown in Fig.4. When the flow rate increases, the impedance modulus of HSn70-1 tube changes somewhat but not significantly; while the impedance modulus of HAl77-2 tube increases comparatively much, which means that the corrosion rate decreases at higher flow rate in that the corrosion procedure of HAl77-2 tube is inhibited.
The above analysis suggests that the diffusion speed of oxygen in the seawater increases when the flow rate gets larger, which makes the filming procedure between the inhibitor and surface of HAl77-2 tube boosts and therefore the corrosion rate decreases. While the filming action between the inhibitor and HSn70-1 tube does not change greatly as the flow rate varies, therefore the impedance modulus of HSn70-1 tube does not change distinctly. The fact that the impedance modulus of HAl77-2 tube is a little higher than that of HSn70-1 tube at the same flow rate indicates that the corrosion resistance of HAl77-2 tube is somewhat better, which is because that HAl77-2 is more susceptive to the flow rate or the oxygen content.
Meanwhile, parameters such as Tafel constant ba, bc and corrosion current density Jcorr can be obtained by potentiodynamic scanning fit and Rp can also be calculated according to Stern-Geary equation. The results are listed in Table 2. At the same flow rate, Rp of HAl77-2 tube ranks higher than that of HSn70-1 tube in evidence. When the flow rate is increased, Rp of both tubes gets a little higher, which is in accordance with the results of
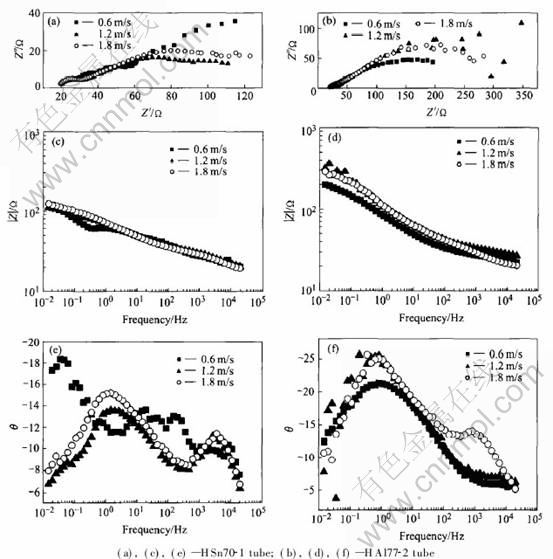
Fig.4 Electrochemical impedance spectroscopies of brass tubes at different flow rates after immersed for 4d
EIS.
As we know that only in limited area of work electrode can the current be distributed, which is determined by the structure of the sensor[9], and this limited area is called effective testing area. From Fig.1 and Fig.3, impedance modulus of HAl77-2 specimens(with exposed area of 1cm2) and that of HAl77-2 tube are compared, and the effective testing area of the sensor is estimated to be around 200cm2. The values of Rp in Table 2 are calculated when taking the effective testing area as 200cm2.
3.2 Features of EN spectrum
The existence of Cl- makes local corrosion such as pitting tend to happen in metal. The random occurring, growing, and dying of the pits on the electrode surface lead to random fluctuation of the current at constant potential, which is called electrochemical noise caused by current fluctuation[10]. The time domain spectra of brass tubes at φcorr under different flow rates are given automatically by PC4/300 GamryTM, as shown in Fig.5. The current in the spectra is the root mean square current after trend removal using linear method[11, 12].
From Fig.5 we can see that the current noise of HSn70-1 tube and that of HAl77-2 tube are similar when the flow rate is kept at 0.6m/s. Howev- er when the flow rate is increased to 1.8m/s,
Table 2 Corrosion rates of tubes at different flow rates
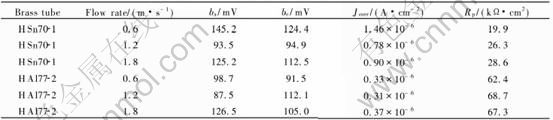
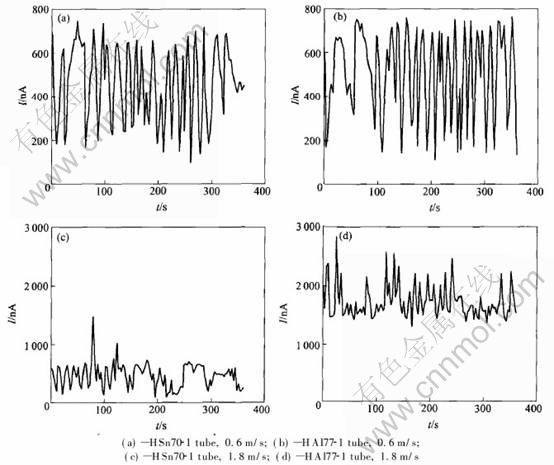
Fig.5 Current noise spectra of brass tubes in condensed seawater
current noise of HAl77-2 tube triples, while that of HSn70-1 tube has a little change. The integral values(Qi) of current noise of brass tubes are given in Table 3. The integral values of current noise do not change significantly at the flow rate of 0.6m/s; however when the flow rate is increased, the integral values of of current noise of HAl77-2 tube triple, while those of HSn70-1 tube have a little change. This shows that at higher flow rate, the total amount of charges of HSn70-1 tube that participate in the electrochemical reaction changes a little while that of HAl77-2 tube triples. This attributes to HAl77-2 tubes increasing susceptivity to local corrosion at higher flow rate. Therefore the EN technology can be used to monitor the change of susceptivity to local corrosion in this way.
On the other hand, EN data of HSn70-1 and HAl77-2 specimens immersed in still condensed seawater after 24h are collected. Current noise spectra of both kinds of specimens are shown in Fig.6, from which linear trend is removed using polynomial method[13, 14], because the presence of the trend can mask the shape of current and po- tential fluctuations[15]. Similar current fluctuation am-
Table 3 Current integration of brass tubes
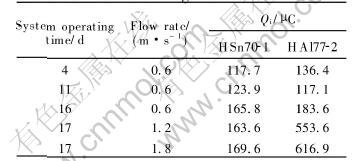
plitude of HSn70-1 and HAl77-2 specimens is observed which presents good agreement with the above results.
EN data are transformed from the time domain into the frequency domain by fast Fourier transformation(FFT). Spectral noise resistance is defined as[16]

where φFFT(f) and IFFT(f) are the FFT of the potential and current fluctuations respectively.
Spectral noise resistances of non-filmed HSn70-1 and HAl77-2 specimens in condensed seawater are compared with corresponding modulus of impedance, which are shown in Fig.7. Good
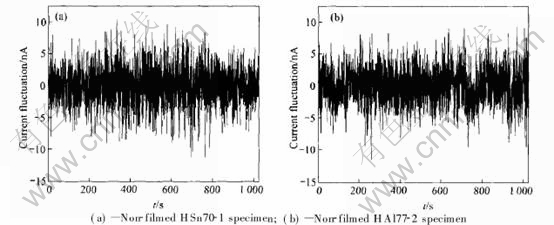
Fig.6 Current noise spectra of specimens in condensed seawater
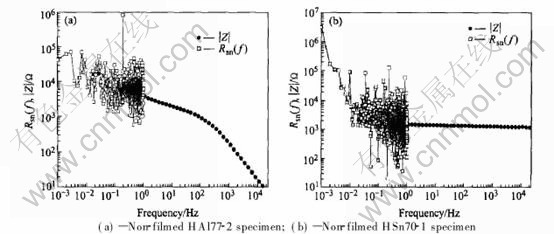
Fig.7 Comparison of spectral noise resistance and impedance modulus
agreements are observed between the spectral noise resistance and the impedance modulus, which is consistent with what Bertocci et al has proved[14].
4 CONCLUSIONS
1) EIS measurement in circulating cooling system shows that the corrosion resistance of brass tubes can be ameliorated by adding inhibitor into the solutions or by filming beforehand on the tubes. The corrosion resistance of HAl77-2 tube is better than that of HSn70-1 tube in seawater.
2) Good agreement between the spectral noise impedance and the modulus of the impedance is observed.
3) The integral values calculated from EN current can be used to monitor the change of susceptivity to local corrosion of brass tube in circulating cooling seawater.
Acknowledgement
The authors gratefully acknowledge the support by Tianjin Seawater Desalting Institute, State Ocean Bureau and thank WU Jie for her contribution to this paper.
REFERENCES
[1]YANG Qing-feng, GU An-zhong, DING Jie, et al. Calcium carbonate fouling behavior and morphology [J]. Journal of Chemical Industry and Engineering, 2002, 53(9): 924-930.(in Chinese)
[2]LI Xiang-qin, LIU Tian-qing, WANG Hong-ling, et al. Mixed fouling growth process-microbial and CaCO3 fouling in water systems [J]. Journal of Chemical Industry and Engineering, 2002, 53(12): 1247-1252.(in Chinese)
[3]PENG Ke-ru, LI Zheng-feng, PENG Ji-min. Study on dezincification corrosion of brass pipes in condensers [J]. Materials Protection, 1995, 28(10): 1-3.(in Chinese)
[4]Chen Y, Jepson W P. EIS measurement for corrosion monitoring under multiphase flow conditions [J]. Electrochimica Acta, 1999, 44: 4453-4464.
[5]Mansfeld F, Lorenz W J. Techniques for characterization of electrodes and electrochemical process [J]. J Wiley, 1991, 581-647.
[6]Gusmano G, Marchioni F, Montesperelli G. A crevice corrosion study by electrochemical noise analysis [J]. Materials and Corrosion, 2000, 51: 537-544.
[7]Aballe A, Bethencourt M, Botana F J, et al. Using wavelets transform in the analysis of electrochemical noise data [J]. Electrochim Acta, 1999, 44: 4805-4816.
[8]YIN Li-hui, SONG Shi-zhe. Study on the sensor for monitoring brass tube corrosion [J]. Journal of Chinese Society for Corrosion and Protection, 2004, 24(1): 52-54.(in Chinese)
[9]SONG Shi-zhe, YIN Li-hui. Corrosion electrochemical study on brass tubes in circulating cooling system [J]. Journal of Chemical Industry and Engineering, accepted.(in Chinese)
[10]CAO Chu-nan. Corrosion Electrochemistry [M]. Beijing: Chemical Industry Press, 1995. 161.(in Chinese)
[11]Mansfeld F, Sun Z, Hsu C H, et al. Concerning trend removal in electrochemical noise measurements [J]. Corrosion Science, 2001, 43: 341-352.
[12]Lee C C , Mansfeld F. Analysis of electrochemical noise data for a passive system in the frequency domain [J] . Corrosion Science, 1998, 40(6): 959-962.
[13]Bertocci U, Huet F, Nogueira R, et al. Drift removal procedures for PSD calculation [A]. NACE [C]. Houston, Texas, 2001, Paper No.01291.
[14]Nagiub A, Mansfeld F. Evaluation of corrosion inhibition of brass in chloride media using EIS and ENA [J]. Corrosion Science, 2001, 43: 2147-2171.
[15]Bertocci U, Frydman J, Gabrielli C, et al. Analysis of electrochemical noise by power spectral density applied to corrosion studies. Maximum entropy method or fast fourier transform? [J]. Electrochem Soc, 1998, 145(8): 2780-2785.
[16]Bertocci U, Gabrielli C, Huet F, et al. Noise resistance applied to corrosion measurements(Ⅰ)—theoretical analysis [J]. Electrochem Soc, 1997, 144(1): 31-36.
(Edited by YANG Bing)
Foundation item: Project(50499335) supported by the National Natural Science Foundation of China
Received date: 2004-07-02; Accepted date: 2005-01-04
Correspondence: SONG Shi-zhe, Professor; Tel: +86-22-27407338; E-mail: szsong@tju.edu.cn