
Static oxidation behaviour of silicide coating on niobium alloy at high temperature
XIAO Lai-rong(肖来荣)1, 2, XU Liang-liang(许谅亮)1, 2, YI Dan-qing(易丹青)1, 2,
LIU Hui-qun(刘会群)1, 2, CAI Zhi-gang(蔡志刚)1, 2
1. School of Materials Science and Engineering, Central South University, Changsha 410083, China;
2. Key Laboratory of Nonferrous Metal Materials Science and Engineering,Ministry of Education, Central South University, Changsha 410083, China
Received 15 July 2007; accepted 10 September 2007
Abstract: A silicide coating was prepared on the surface of the Nb521 alloy by the complex pack cemented method. The oxidation resistance properties of the present coating were examined by the static oxidation tests at 1 700 ℃ in air. The compositions and the microstructures of the coating before and after test were characterised and analysed through scanning electron microscopy (SEM), X-ray diffraction analysis (XRD), energy dispersive X-ray spectrometry (EDS) and electron probe microanalysis (EPMA), respectively. The present silicide coating can provide an effective protection for the Nb alloy for 25 h at 1 700 ℃ in air. The results show that the oxidation kinetics of the present silicide coating is parabolic. The diffusion of Si leads to the phase transformation and evolution during the oxidation.
Key words: niobium alloy; silicide coating; static oxidation; diffusion; phase transformation
1 Introduction
Niobium and its alloys have been considered as one of the important candidates of high temperature structural materials in aero and aeronautical applications, due to the benefits of their high melting point, low density and high strength at high temperature[1, 2]. However, the engineering application of them have been seriously restricted due to their relatively low oxidation resistance[3]. Therefore, as one of a relatively effective ways to improve the oxidation resistance, protective coatings on niobium alloys get much attention. The protective effect of a sputtered TiAlCrAg coating based on Ti-Al-Nb alloy was experimentally studied at 900 ℃[4]. By using arc surface alloying technology and molten salt method, the prepared aluminide and NbSi2 coating on niobium can work at 1 200 ℃ and 1 250 ℃, respectively[5-6]. A TiSi2 coating fabricated by pack cemented method can work at 1 300 ℃ for 30 h[7]. But these protective coatings mentioned above can not meet the requirement of the increasing temperature and enduring time in the newly developed high temperature engine, specifically for the niobium alloy components in the two-component engine for orbit attitude control. This demonstrates that the modified Ti-Cr-Si coating fabricated by slurry sintering method can work at 1 600 ℃ for 17 h[8]. As another important potential silicide coating system, the MoSi2 coating is expected to work at temperature up to 1 800 ℃[9].
In the present study, MoSi2 coating was fabricated on Nb521 alloy by a complex pack cemented method. The static oxidation behavior of the obtained silicide coating at 1 700 ℃ in air was studied, and the micro- structural evolution and phase transformation during oxidation were investigated as well.
2 Experimental
Nb521 alloy (Nb-5W-2Mo-1Zr) was selected as matrix and was cut into specimens with dimension of 3 mm×3 mm×80 mm. After grinding by sand paper, the specimens were treated by ultrasonic cleaning in ethanol, surface treatment of acid solution and cleaning in distilled water, respectively. Then the treated specimens were dried in an oven and ready to be coated.
Slurry was prepared by mixing the Mo powder (D50=0.6 μm, purity>99.9%) with an organic solvent. After dip-coating and sintering at 1 500 ℃, Mo coating was prepared on surface of the Nb521 alloy. The subsequent silicide coating was fabricated by pack cemented treatment of the Mo-coated Nb alloy specimens in Si powder (D50=3.3 μm, purity>99.9%) at 1 200 ℃ in Ar atmosphere.
The fabricated coating specimens were heated from room temperature to 1 700 ℃. The static oxidation tests were carried at 1 700 ℃ in air until there were obvious defects such as cracks, holes and color change on the specimens. The mass change of the specimens during static oxidation test was recorded by an analytical balance.
The morphology and the structure of the specimen on surface and cross-section were observed by a field emission SEM (Sirion200). The XRD (D/max 2500 VB, Cu target, λ=0.154 06 nm, scan rate 8?/min) was used to identify the phases in the coating. The distribution of compositions in surface and cross-section of coating was investigated via the EDS (Genesis60s). An EPMA (JXA-8800R, 20 kV, 2×10-8 A) was chosen for the elemental analysis of the specific areas on cross-section of the coating specimens.
3 Results and discussion
3.1 Static oxidation resistance properties of coating
The static oxidation test indicates that the present silicide coating can work at 1 700 ℃ for 25 h. A glass film can be observed on the surface of the coating during oxidation. After keeping for 25 h at 1 700 ℃ in air, a few burr-like structures are generated at the corner and edge of the specimens and a large amount of black spots can also be seen in the meantime. Subsequently, the coating is gradually devoiced from matrix with the proceeding of the oxidation and causes the final failure of the protective coating. It can be seen from the mass-gained curve of the specimen during the static oxidation tests shown in Fig.1, the oxidation kinetics of the present silicide coating at 1 700 ℃ is parabolic. The mass increases relatively faster at the beginning of the oxidation tests, then the curve enters into a steady oxidation process before the final failure, in which the mass of the specimen decreases sharply due to the coating fallen apart from the matrix.
3.2 Microstructural evolution of coating during static oxidation tests
Fig.2 shows the morphology evolution of the surface on the silicide coating before oxidation.
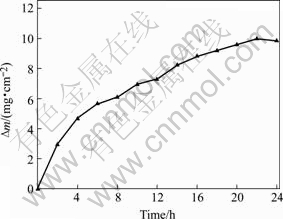
Fig.1 Oxidation kinetics of coating at 1 700 ℃ in air
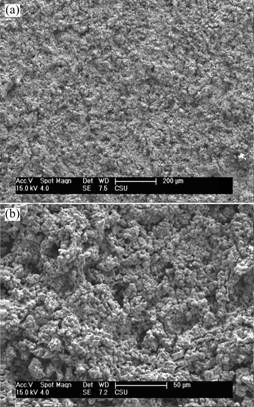
Fig.2 Surface photographs of original coating: (a) Cracks on surface of coating; (b) Holes on surface of coating
Incoherent structures are covered on the rough surface of the coating with a few cracks and micro-holes can be seen in higher magnification image (Fig.2(b)).
As seen in Fig.3, the surface of the coating is covered with melted glass film after oxidation for 8 h at 1 700 ℃. Compared with the coating before oxidation, the surface of the oxidized coating is relatively dense and smooth. Combined with the results from XRD and EDS, SiO2 film is created on the surface of the coating during the high temperature oxidation. The possible reactions of the MoSi2 under high temperature oxidized atmosphere are as follows:
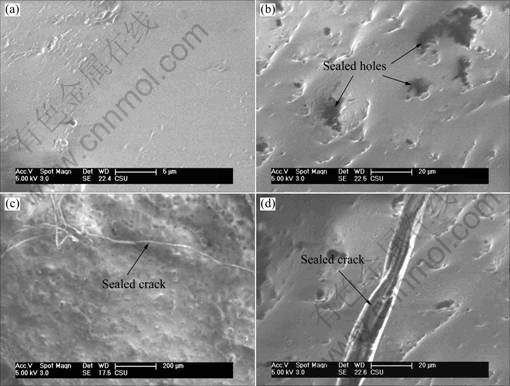
Fig.3 Surface photographs of coatings after oxidation for 8 h at 1 700 ℃ in air: (a) Oxide film on coating surface; (b) Sealed holes on coating surface; (c), (d) Sealed cracks on coating surface
5/7MoSi2+O2=1/7Mo5Si3+SiO2 (1)
MoSi2+7/2O2=2SiO2+MoO3 (2)
Because the melting point of SiO2 (1 480 ℃) is much lower than the testing temperature, the melted SiO2 phases can be formed. During the oxidation, this melted SiO2 has a certain flow ability to seal a number of micro holes and cracks on the surface. Hence the surface of the coating becomes relatively smooth and dense in comparison with that before oxidation. The amorphous bump of the SiO2 can be revealed by XRD in Fig.4, in which a few Al2O3 peaks are caused by the contamination from the alumina packing agent during pack cemented process.
It can be concluded from Fig.3 that the self-healing properties of the present silicide coating can seal the micro defects on the surface, and consequently prevent the quick diffusion of the oxygen into the coating through those existed defects. Moreover, due to the low diffusive rate of the oxygen in SiO2 (in an order of magnitude of 10-3[10]), the dense SiO2 film formed on the surface can give MoSi2 a remarkable oxidation resistance at high temperature. The coating has a typical dual phases structure before oxidation, as shown in
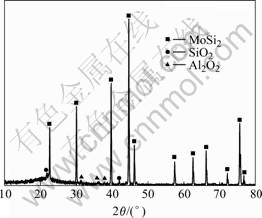
Fig.4 XRD of coating surface after oxidation for 8 h at 1 700 ℃ in air
Fig.5(a). The EPMA results indicate that the relatively loose outer layer is the main body of the coating, which consists of MoSi2, while the dense NbSi2 phase forms the transitional layer between the matrix and the coating.
The SEM image of the cross-section of the coating after oxidation in air at 1 700 ℃ for 2 h is shown in Fig.6(a). From the EPMA (Fig.6(b)) and the ternary phase diagrams of Mo-Nb-Si, five layers in the oxidized coating can be observed from coating to matrix: SiO2→MoSi2→(Mo, Nb)5Si3→NbSi2→Nb5Si3. Compared with the original coating, the transitional layer (NbSi2) becomes thinner and two new phase layers ((Mo, Nb)5Si3 and Nb5Si3) are formed. It can also be found that the micro-holes existed in MoSi2 layer are filled with SiO2 phases.
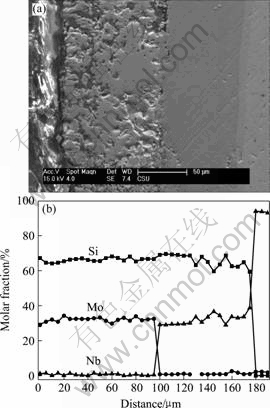
Fig.5 SEM photograph and EPMA of cross-section of coatings: (a) SEM image of coating; (b) EPMA of coating
At the beginning of the oxidation, the partial pressure of oxygen on the surface of the coating is high enough to cause the oxidation of both Si and Mo elements in rough coating surface. With the proceeding of the oxidation, the initially generated MoO3 is quickly volatilized at high temperature (1 700 ℃), but leaves the created SiO2 reserved and much areas of the surface is covered by it in the meantime. This is a fast oxidation process corresponding to the deep slope part of the mass curve in Fig.1. The formed dense SiO2 film prevents the diffusion of the oxygen to the reactive interface in the coating, while the diffusion rate of the silicon element to the surface becomes larger and larger until the balance between the consumable rate and the diffusive rate of the silicon is reached. By now the coating shows a relatively stable oxidation behavior, which is in correspondence to the steady mass gained process in static oxidation test (Fig.1).
The oxidized process and related reactions are schematically illustrated in Fig.7. The silicon element in MoSi2 coating not only diffuses to the surface to react with the oxygen but also moves to the direction of matrix to form the NbSi2 transitional layer at high temperature in air. Figs.6 (c) and (d) are the back scatter SEM images of the cross-section area of the specimens oxidized after 4 h and 6 h, respectively. The thickness of the oxidized layer increases with the prolongation of the oxidized time. The diffusion of Si element driven by the chemical potential gradient causes the phase transformation in the coating. As a result, Nb5Si3 layer and (Mo, Nb)5Si3 layer are created near the matrix and the surface, respectively. With the proceeding of oxidation, the thickness of the Nb5Si3 layer and (Mo, Nb)5Si3 layer increases while silicon rich layers (MoSi2 layer and NbSi2 layer) are consumed gradually.
Fig.6(e) shows the SEM image of the cross-section of the coating specimen after oxidation for 8 h. According to the corresponding EPMA results shown in Fig.6 (f), the main body of the coating consists of the MoSi2, (Mo, Nb)5Si3 and Nb5Si3 layers without the presence of the NbSi2 layer. Similar structures can also be observed in Figs.6 (g) and (h), which show SEM images of the cross-section of the oxidized coating after 12 h and 24 h, respectively. The effect of the diffusion of Si to the direction of matrix, which is one of the major parts of Si consumption in the coating during oxidation, is notable at high temperature. After oxidation for 24 h, the thickness of the left MoSi2 layer is only 34.62 μm, while the thickness of (Mo, Nb)5Si3 and Nb5Si3 layers increases to 167.60 μm, as shown in Fig.6 (h). It is proposed that the restraint of the diffusion of Si to Nb matrix can improve the oxidation resistance to some extent. The oxidized process and related reactions are also schematically illustrated in Fig.7.
4 Conclusions
1) The MoSi2 coating prepared by the complex pack cemented method can provide an effective protection for Nb521 matrix in air at 1 700 ℃ for 25 h. The oxidation kinetics of the present silicide coating at 1 700 ℃ is parabolic. The mass curve of the coating during static oxidation test shows a quick increasing process before a steady increasing period approaches.
2) The generated SiO2 film on the surface prevents the further diffusion of the oxygen into the coating. Due to the existence of the molten SiO2 at high temperature, a number of micro-holes and cracks can be sealed by the flow of SiO2, which makes the present silicide coating have a self-healing behaviour.
3) During the oxidation, the phase transformation and the microstructural evolution are caused by the diffusion of Si in the coating both to the surface and the matrix, which is driven by the force of the chemical potential gradient of Si.
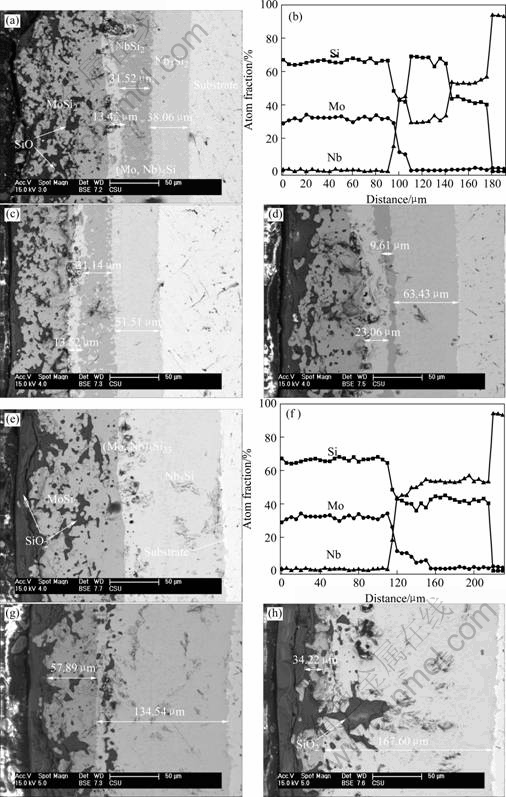
Fig.6 SEM photograph and EPMA of cross-section of coating after oxidation for different time at 1 700 ℃ in air: (a) BSE image of coating after oxidation for 2 h; (b) EPMA of coating after oxidation for 2 h; (c) BSE image of coating after oxidation for 4 h; (d) BSE image of coating after oxidation for 6 h; (e) BSE image of coating after oxidation for 8 h; (f) EPMA of coating after oxidation for 8 h; (g) BSE image of coating after oxidation for 12 h; (h) BSE image of coating after oxidation for 24 h
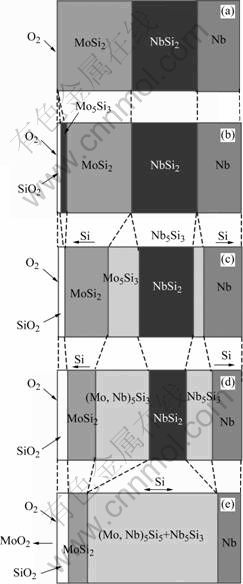
Fig.7 Schematic illustration of oxidation of coating at 1 700 ℃: (a) Model before oxidation; (b) Model of initial oxidation; (c) Model of momental oxidation; (d) Model of process of oxidation; (e) Model of ending oxidation
References
[1] SHA J, HIRAI H, TABARU T, et al. Mechanical properties of as-cast and directionally solidified Nb-Mo-W in-situ composites at high temperature[J]. Metallurgical and Materials Transactions, 2003, 34A(1): 85-94.
[2] DOLLAR A, DYMEK S. Microstructure and high temperature mechanical properties of mechanically alloyed Nb3Al-based materials[J]. Intermetallics, 2003, 11: 341-345.
[3] VILASI M, FRANCOIS M, PODOR R, STEINMETZ J. New silicides for new niobium protective coatings[J]. Journal of Alloys and Compounds, 1998, 264: 244-251.
[4] XI Yan-jun, WANG Fu-hui, HE Lian-long. Protective effect of the sputtered TiAlCrAg coating on the high-temperature oxidation and hot corrosion resistance of Ti-Al-Nb alloy[J]. Journal of Materials Science and Technology, 2004, 20(6): 724-727.
[5] MATSUURA K, KOYANAGI T, OHMI T. Aluminide coating on niobium by arc surface alloying[J]. Materials Transactions, 2003, 44(5): 861-865.
[6] Suzuki R O, Ishikawa M, Ono K. NbSi2 coating on niobium using molten salt[J]. Journal of Alloys and Compounds, 2002, 336: 280.
[7] GLUSHKO P I, SEMENOV N A, STRIGUNOVSKII S V, MIROSHNICHENKO K V. Oxidation resistance of niobium coated with titanium disilicide[J]. Powder Metallurgy and Metal Ceramics, 2000, 39(11/12): 360.
[8] WANG Yu, CHEN Min. Microstructure and crack propagation of Ti-Cr-Si coatings on the niobium alloy C-103[J]. Rare Metal Materials and Engineering, 2000, 29(5): 315.(in Chinese)
[9] Hidouci A, Pelletier J M. Microstructure and mechanical properties of MoSi2 coatings produced by laser processing[J]. Materials Science and Engineering A, 1998(252): 17-26.
[10] JIN Shi. High-temperature oxidation resistant coatings[M]. Beijing: Science Press, 1980: 25-40.
[11] KIM W Y, TANAKA H, KASAMA A, et al. Microstructure and room temperature deformation of Nbss/Nb5Si3 in situ composites alloyed with Mo[J]. Intermetallics, 2001, 9: 521-527.
(Edited by CHEN Can-hua)
Foundation item: Supported by the Science and Technology Program of Hunan(05JT1065)
Corresponding author: XIAO Lai-rong; Tel: +86-731-8830137; E-mail: xiaolr368@sina.com