
High-temperature oxidation behavior of Ti3Si(1-x)AlxC2 in air
LIU Xiao-pan(刘小磐), WAN Long(万 隆), WANG Yang(汪 洋), MA Wen-min(马文闵)
College of Materials Science and Engineering, Hunan University, Changsha 410082, China
Received 10 April 2006; accepted 25 April 2006
Abstract: The oxidation behaviors of bulk Ti3Si(1-x)AlxC2 prepared by hot pressing were investigated. The results show that the isothermal oxidation behavior of Ti3SiC2 obeys a parabolic law between 900 and 1 100 ℃ and follows a two-step parabolic rate law between 1 200 ℃ and 1 300 ℃. The cyclic oxidation behavior of material is assumed to obey a three-step parabolic rate law at
1 100 ℃ and 1 200 ℃. The calculated activation energy of isothermal oxidation is 101.43 kJ/mol. The oxide layers which consist of a mass of α-Al2O3 and little TiO2 and SiO2 are observed on Ti3SiC2 as a dense and adhesive protect scale. The oxidation mechanism varies with the additive aluminum that greatly improves the oxidation resistance of Ti3SiC2.
Key words: Ti3SiC2; Ti3Si(1-x)AlxC2; isothermal oxidation; cyclic oxidation; oxidation kinetics
1 Introduction
Recently, many researchers have put their focuses on a nanolaminate compound Ti3SiC2, which combines the merits of metals and ceramics. Like metals, it is thermally and electrically conductive, easy to machine with ordinary tools, and well resistant to thermal shock. Like ceramics, it has high strength, high melting point (>3 000 ℃) and resistant to oxidation[1-4]. All these merits are due to its special layered microstructure. Ti3SiC2 has hexagonal crystal structure with space group of P63/mmc[1]. The theoretical gravity, measured elastic modulus and VICKERS hardness is 4.53 g/cm3, 320 GPa and 4 GPa, respectively[1, 2]. As an excellent oxidation- resistance material, the oxidation behaviors of Ti3SiC2 or Ti3SiC2-based composite material had been investigated by several researchers. Since the characters of studied materials, such as contents, microstructure and synthesized methods, were different from each other, varied oxidation behaviors were reported[5-9].
In this study, the isothermal oxidation and cyclic oxidation at high temperature of bulk Ti3SiC2 sintered by hot pressing with the starting mixture powders (x(TiC)∶ x(Ti) ∶x(Si) ∶x(Al)=2∶1∶1∶0.2) were investigated. The additive aluminum, which did favor to the fabrication of Ti3SiC2, and also improved the high-temperature oxidation resistance.
2 Experimental
2.1 Material preparation
In our last work, a novel fabrication method of Ti3SiC2 was introduced with the aluminum addition. The mixture powders with a designed composition in molar ratio of x(TiC)∶x(Ti)∶x(Si)∶x(Al)=2∶1∶1∶0.2 were mixed in ethanol for 24 h. Then the mixture powders were placed in a graphite die, 50 mm in diameter, with a layer of BN previously brushed on the inner surface. The samples were sintered in a hot pressing system at 1 350 ℃ in an Ar atmosphere for 2 h at a pressure of 30 MPa. The density and purity of the sintered products were 4.49 g/cm3 and 98.2% in mass fraction, as measured by ARCHIMEDE’s method, XRD analysis and SEM observation. EDS and EPMA results revealed the additive aluminum still exists in the matrix, though the loss of aluminum is much more than that of silicon at high temperature. The compound can be described by the formula, Ti3Si(1-x)AlxC2(0<x<0.16)[11]. In the following study, the formula is abbreviated as TSAC. Further investigation demonstrates that Ti, Al, Si are uniformly distributed in the matrix.
2.2 Oxidation experiments
The rectangular bars with dimensions of 4 mm×4 mm×10 mm were cut by the electrical discharge method for oxidation experiments. The surfaces were ground down to the 800-grit SiC paper. The thermal oxidation experiments were performed from 900 ℃ to 1 300 ℃ in air for 20 h with an interval of 100 ℃. All samples were put in the Al2O3 crucibles and held at the oxidation temperature in a furnace to requisite time. The cyclic oxidation experiment was also performed in the furnace at the temperature of 1 100 ℃ and 1 200 ℃. The samples were held at the temperature for 1 h and cooled to room temperature in 0.5 h, which was called a cycle. The mass gains were measured by a microbalance with ±10-4 g accuracy.
The X-ray diffraction (XRD) was used to determine the phases of oxide using a rotating anode X-ray diffractometer (Model D/MAX-RB, RIGAKU Corporation, Japan). The microstructures of oxide scales were investigated with scanning electron microscopy (SEM, Model JSM-5610LV, JEDL. Ltd, Japan) coupled with energy-dispersive spectroscopy (EDS, Model Phoenix, EDAX, USA) for composition analysis.
3 Results and discussion
3.1 Oxidation kinetics
The dependence of mass gain per unit surface area on oxidation time at 900-1 300 ℃ is shown in Fig.1(a).
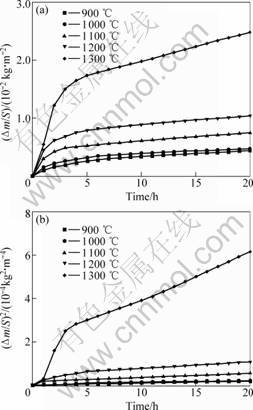
Fig.1 Mass gain per unit area versus time (a) and square of mass gain unit area with time (b) for Ti3SiC2 oxidized at different temperatures
It can be seen that the specific mass gains are relatively small at these tested temperatures. The value 2.48×10-2 kg/m2, which is obtained at 1 300 ℃ for 20 h is much smaller than the values of Ti3SiC2 and Ti3AlC2, 75×10-2 kg/m2 and 3.5×10-2 kg/m2, under similar condition reported by SUN et al[9, 10]. The results indicate that TSAC has good high-temperature oxidation resistance.
Correspondingly, the data shown in Fig.1(b), is the dependence of the square of mass gain per unit area against oxidation time. The curves of 900 ℃ and 1 100 ℃ are in agreement with the linear law, and there is almost no difference for the mass gain between 900 ℃ and 1 000 ℃. The curves of 1 200 ℃ and 1 300 ℃ are rather complicated. The two-step parabolic oxidation process was supposed to explain the oxidation kinetics[9]. So we suggest the oxidation of TSAC generally obeys the parabolic rate law, which can be expressed by the following equation:
(Δm/S)2=kpt+c (1)
where Δm/S is the mass gain per unit surface, kp is the parabolic rate constant, t is the time and c is a constant.
The parabolic rate constants at different temperatures, listed in Table 1, are calculated according to Fig.1(b). At 1 200 ℃, the parabolic rate constants are 3.57×10-9 kg2?m-4?s-1 for 1-5 h and 8.08×10-10 kg2?m-4?s-1 for 6-20 h; at 1 300 ℃, the parabolic rate constants are 2.48×10-8 kg2?m-4?s-1 for 1-4 h and 5.71×10-9 kg2?m-4?s-1 for 5-20 h, which suggests that a protect scale that prevents father oxidation was formed on the surface in the first 5 h at 1 200 ℃ and 4 h at 1 300 ℃.
Table 1 Parabolic rate constants at 900-1300 ℃
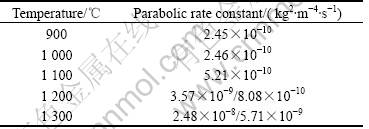
In order to get more information of the oxidation process of TSAC, the activation energy Q was calculated in terms of the Arrhenius-type formula:
kp=k0exp(-Q/RT) (2)
where k0 is the pre-exponential factor, Q is the effective activation energy, R is the universal gas constant and T is the absolute temperature.
The temperature dependence of the parabolic rate constants is shown in Fig.2. According to the slope of the line shown in Fig.2, a value of Q was calculated as (110±10) kJ/mol, however the value of Ti3SiC2 consisting of 2%(volume fraction) TiC is (370±20) kJ/mol[6], and the value of Ti3AlC2 is 175 kJ/mol[11]. But the much lower parabolic rate constants values resulted in the excellent oxidation behavior.
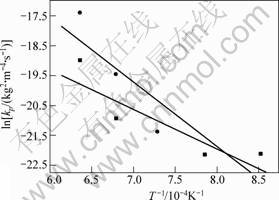
Fig.2 Arrhenius-type plot for kp at 900-1 300 ℃
The cyclic oxidation behavior of TSAC at 1 100-1 200 ℃ for 40 cycles was also investigated. The dependence of mass gain per unit surface area against number of cycles is shown in Fig.3 (a). Correspondingly, the square of the mass gain per unit surface area versus numbers of cycles is shown in Fig.3 (b).
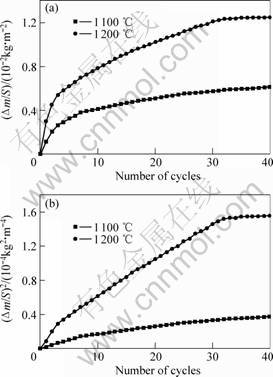
Fig.3 Plots of mass gain per unit surface area against number of cycles (a) and dependence of square of mass gain with number of cycles (b) for Ti3SiC2 oxidized at 1 100 ℃ and 1 200 ℃
A three-step cyclic oxidation process is obtained by carefully analyzing the two curves. In the first step, i.e. at the first 6 cycles for 1 100 ℃ and the first 4 cycles for
1 200 ℃, a sharp oxidation process is observed. In the second step, i.e. from 7 to 33 cycles for 1 100 ℃ and 4 to 31 cycles for 1 200 ℃, the oxidation rate is much slower. In the third step, i.e. after 33 cycles at 1 100 ℃ and 31 cycles at 1 200 ℃, nearly no mass gain is observed. Obviously, the formation and densification of the dense oxide scale occur in the first and second step, while in the third step the dense oxide scale prevents the TSAC from further oxidizing. It takes more time to densify the oxide scale at 1 100 ℃ due to the lower temperature. No macro crack and spallation were observed at the oxidized surface.
3.2 Phase composition and microstructure of scale
To study the excellent oxidation resistance of TSAC samples, XRD and SEM were used to analyze the phase composition and surface morphology of the oxide scales, respectively. The XRD patterns of the samples oxidized at different temperatures for 20 h are shown in Fig. 4. Peaks from reflection on α-Al2O3, TiO2 (rutile) and Ti3SiC2 can be seen, and there are no peaks of SiO2 and other carbides. It is also seen that the main peaks belong to Ti3SiC2 even at the temperature of 1 200 ℃, and its intensities decrease as oxidized temperature increases, which indicates the oxide scales are very thin and prove the good oxidation resistance of samples from the other point. The composition of cyclic oxide scale was also analyzed by X-ray diffraction (Fig.5). The reaction products, α-Al2O3 and TiO2, and Ti3SiC2 are also observed. The peaks of Ti3SiC2, whose intensities decrease with temperatures increase, are also present. The intensities of the α-Al2O3 and TiO2 peaks are
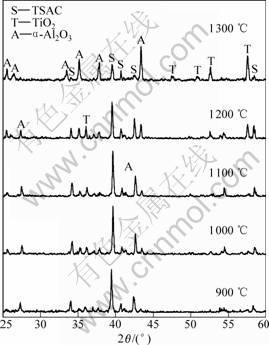
Fig.4 XRD patterns of scale surface for 20 h at different temperatures
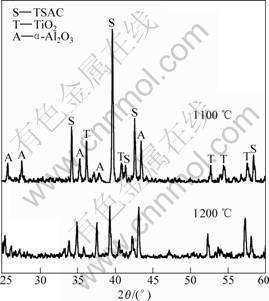
Fig.5 XRD patterns of scale surface after cyclic oxidation for 40 cycles at 1 100 ℃ and 1 200 ℃
strengthened with the increase of temperature.
The SEM micrographs of scales oxidized at 1 200 ℃ and 1 300 ℃ for 20 h are shown in Fig.6. The thicknesses of oxide scales are 4-7 μm at 1 200 ℃ and 8-10 μm at 1 300 ℃, respectively. Fig.7 shows the
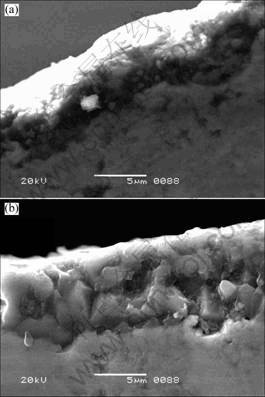
Fig.6 SEM micrographs of samples after oxidation for 20 h at 1 200 ℃ (a) and 1 300 ℃ (b)
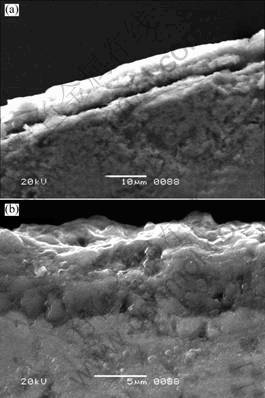
Fig.7 Secondary electron micrographs of samples after cyclic oxidation for 40 cycles at 1 100 ℃ (a) and 1 200 ℃ (b)
oxide-surface micrographs of samples cyclic-oxidized at 1 100 ℃ and 1 200 ℃ for 40 cycles, respectively. The thicknesses of dense oxide scales are about 6-8 μm. The large micro-crack can be observed between matrix and oxide scale. As shown in Table 2, the results from EDS spectra indicated the oxide scales consisted of dense α-Al2O3, and companied with little TiO2 and SiO2 which prevent Ti3SiC2 from further oxidizing at high temperature. However, there are no peaks of SiO2 in XRD patterns. It might be attributed to the fact that the SiO2 content is so low that XRD can not detect it.
Table 2 Approximate mass fraction and volume fraction of products in oxidized scale after cyclic oxidation at 1 200 ℃

3.3 Oxidation mechanism
In order to understand the excellent resistance to oxidation of TSAC further, it is important to appreciate the primary oxidation mechanism. Many researchers have reported the oxidation of Ti3SiC2 was controlled by the outward diffusion of titanium and inward diffusion of oxygen[7]. However, the oxidation product mainly consists of α-Al2O3 in our study, which suggests the oxidation mechanism has completely varied with the addition of aluminum.
At initial oxidation stage, the in-situ oxidation of TSAC occurred. TiO2 and SiO2 were formed, and α-Al2O3 was also formed with the addition of aluminum. The total reaction is summarized in the following equation:

(0<x<0.16)
As the addition of aluminum, the further oxidation of TSAC is not controlled by the outward diffusion of titanium and inward diffusion of oxygen, but by the outward diffusion of aluminum and inward diffusion of oxygen. As the densification of oxide scales, the two diffusion processes were held back. It is why TSAC has better oxidation resistance than Ti3SiC2.
4 Conclusions
The oxidation kinetics of monolithic TSAC prepared by hot pressing generally obeys a parabolic law. The dense and adhesive oxide scales formed on TSAC consisting of a great deal of α-Al2O3 and little TiO2 and SiO2, could greatly improve the high-temperature oxidation resistance of Ti3SiC2. The additive aluminum changes the oxidation mechanism of Ti3SiC2 with the outward diffusion of aluminum and inward diffusion of oxygen. A compressive stress was calculated in oxide scale cyclic-oxidized at 1 200 ℃, which makes the scales tend to craze.
References
[1] BARSOUM M W, EL-RAGHY T. Synthesis and charaterization of a remarkable ceramic: Ti3SiC2[J]. J Am Ceram Soc, 1996, 79 (7): 1945-1956.
[2] EL-RAGHY T, ZAVALIANGES A, BARSOUM M W, et al. Damage mechanisms around hardness indentations in Ti3SiC2[J]. J Am Ceram Soc, 1997, 80(2): 513-516.
[3] BARSOUM M W, EL-RAGHY T, RAWN C, et al. Thermal properties of Ti3SiC2[J]. J Phys Chem Solids, 1999, 60 (1): 429-439.
[4] El-RAGHY T, BARSOUM M W, ZAVALIANGES A, et al. Processing and mechanical properties of Ti3SiC2, Part Ⅱ: mechanical properties[J]. J Am Ceram Soc, 1999, 82(10): 2855-2860.
[5] RACAULT C, LANGLAIS F, NASLAIN R. On the chemical vapor deposition of Ti3SiC2 from TiCl4-SiCl4-CH4-H2 gas mixtures[J]. J Mater Sci, 1994, 29, 3384-3390.
[6] BARSOUM M, EL-RAGHY T. Oxidation of Ti3SiC2 in air[J]. J Electrochem Soc, 1997, 14: 2508-2516.
[7] TONG X, OKANO T, ISEKI T, YANO T. Synthesis and high temperature mechanical properties of Ti3SiC2/SiC composites[J]. J Mater Sci, 1995, 30: 3087-3090.
[8] FENG A, ORLING T, YANO T, MUNIR Z A. Field-activated pressure-assisted combustion synthesis of polucrystalline Ti3SiC2[J]. J Mater Res, 1999, 14: 925-939.
[9] SUN Z, ZHOU Y, LI M. Oxidation behavior of Ti3SiC2-based ceramic at 900-1 300 ℃ in air[J]. Corrosion Science, 2001, 43: 1095-1109.
[10] SUN Z, ZHOU Y, LI M. Cyclic-oxidation behavior of Ti3SiC2-based material at 1 100 ℃[J]. Oxidation of Matels, 2002, 57: 379-394.
[11] WANG X H, ZHOU Y. Oxidation behavior of Ti3AlC2 at 1 000-1 400 ℃ in air[J]. Corrosion Science, 2003, 45: 891-907.
(Edited by YANG You-ping)
Foundation item: Project (KF200505) supported by the Key Laboratory of Heavy Ion Physics (Peking University),Ministry of Education
Corresponding author: LIU Xiao-pan; Tel: +86-731-8822269; Fax: +86-731-8821483; E-mail: liuxiaopanjj@126.com