
Design method of electromagnetic field applied to
Al-alloy electromagnetic casting
YANG Jing(杨 晶), DANG Jing-zhi(党惊知), PENG You-gen(彭有根), CHENG Jun(程 军)
School of Materials Science and Engineering, North University of China, Taiyuan 030051, China
Received 28 July 2006; accepted 15 September 2006
Abstract: The electromagnetic pump imposes the electromagnetic motive force (Lorentz force) on the liquid metal directly and makes it move along the definite direction by using the function of electric current and magnetic field in the conducting fluid. Compared with the traditional die casting, the system of counter-gravity casting can effectively control the speed of filling to make Al-alloy liquid fill steadily by adjusting controlled-current. So the foundry defects can be decreased or avoided effectively by this system. Based on the theory of electromagnetic pump, the design method of electromagnetic field in electromagnetic pump was investigated emphatically. The rule of magnetic induction intensity B influenced by the divided electromagnet airgap’s size was founded. Furthermore, the empirical formula of magnetic induction intensity B in a magnetic airgap for an open magnet in the saturated state was deduced by mathematics regression analysis. Counter-gravity casting applied to the Al-alloy electromagnetic filling was developed with this method. Besides, the electromagnetism filling counter-gravity casting process of the turbo-charge blade wheel was also fixed. The eligibility rate of blade wheel produced by such technique can be increased to 98%. The casts have compact structure and excellent capability.
Key words: Al alloy; casting; electromagnetic pump; magnetic induction
1 Introduction
Electromagnetic casting of materials is a process to combine magneto hydrodynamics and technique of materials molding, and to impose electromagnetic field on the process of materials molding, thereby implements the control of materials forming processes and improves the structure and mechanical properties [1-3]. In the end of the 1970s, Cosworth[4] developed the CP technique which regards the transmission and filling with electromagnetic pump as the core to produce the cylinder body and cylinder head of first-rank formula car. A foundry of Ford Corporation adopted the system of low pressure die casting based on electromagnetic pump and put automatic control into practice entirely in 1990s. More than three million automobile engine cylinder head have been produced each year. The rate of finished product was stabilized above 90% [5].
Nationally, the electromagnetic filling low-pressure die casting equipment used in Al-alloy is originated to the Center of Foundry in North University of China [6-8]. The system of counter-gravity casting controls the speed of rising and filling in liquid and flux to make Al-alloy liquid fill steadily by adjusting control-current and the size of magnetic field, by which several phenomena can decrease or avoid, such as writhe, impact and splash, aroused by the air pressure fluctuation of the system of pneumatic counter-gravity casting in filling course.
Based on the theory of electromagnetic pump, the design method of electromagnetic field applied to the Al-alloy electromagnetic filling is developed in this paper. The rule of magnetic induction intensity B influenced by the divided electromagnet airgap’s size is researched. Furthermore, the empirical formula of magnetic induction intensity B between a magnetic airgap for an open magnet in the saturated state is deduced by mathematics regression analysis [9]. With such design method, the electromagnetic filling counter-gravity casting process of the turbo-charge blade wheel is also fixed. As a result, the form of oxidizing slag is reduced, the purity degree of aluminum alloy liquid is improved and the foundry defects are decreased. The eligibility rate of blade wheel produced by such technique can be increased to 98%. The casts have compact structure and excellent mechanical properties [10-11].
2 Brief introduction about principle of electromagnetic pump
The electromagnetic pump makes use of the function of| electric current and magnetic field in the conducting electricity fluid and puts the electromagnetic motivation force (Lorentz force) into the metal liquid directly and makes it move along definite direction. The operating principle of the electromagnetic pump and the relationship among the primary parameters, which include magnetic induction intensity B and the current density J that flows through the liquid metals and pressing head, Δp, the main technique and performance index as well, are shown in Fig.1 [12].
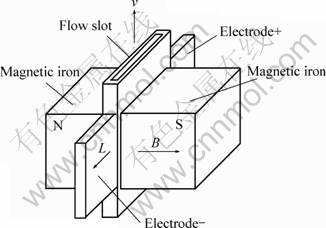
Fig.1 Operating principle of AC electromagnetic pump (J—Current density that is perpendicular to magnetic induction intensity and flowing direction of metal liquid; B—Flowing direction of magnetic induction intensity that is perpendicular to current direction and metal liquid; L—Length of metal liquid that lies in cleft of electromagnet)
3 Influence of electromagnet airgap’s size on magnetic induction intensity in magnetic field spacing
3.1 Structure of divided and uncork electromagnet
Based on electromagnetic pump, the structure of the divided and uncork electromagnet is designed in experiment, and its radical shape is shown in Fig.2. The magnet cores are symmetrical and the section is square, whose material is electric pure iron DT4. Some features of this structure are as follows.
1) Magnet cores are divided and magnet clearance is often bigger than usual magnet;
2) In consideration of installing structure, the exciter winding is distributed asymmetrically;
3) In demand of machining, fixing and spout structure, magnet cores should have edge angle and joint plane relatively;
4) Magnet cores are divided, taking centerline as symmetrical axis and dividing or folding along the plane.
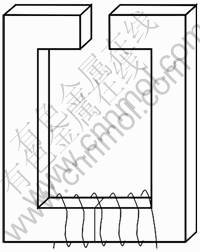
Fig.2 Structure of divided and uncork electromagnet
Magnetic field has a distribution according to solid in magnetic pole clearance. Magnetic flux transits from the end surface of magnetic pole and besides, it drills through side, edge line as well as edges and corners. All of them are called brink magnetic flux which also belongs to air gap’s magnetic flux. In addition, along with airgap increasing, the brink magnetic flux’s proportion in airgap’s magnetic flux becomes bigger and bigger.
3.2 Relationship between magnetic induction intensity and uncork airgap of magnet
The size of magnetic induction intensity B in airgap is measured with SG-3-A type digital T meter in experiment, whose measuring range is (200±0.1) mT. A group of selected samples are used to investigate the influence of airgap’s width on magnetic induction intensity. Magnet cores sectional area S and centerline perimeter L0 in the structure parameter of four samples are unchanged, namely S=41 mm×41 mm, L0=1 020 mm. Only the width of airgap δ is changed, such as 20 mm, 25.4 mm, 28 mm and 32 mm. The change of magnetic induction intensity B in airgap in different magnetization H is measured. Fig.3 indicates that the curve based on magnetization H in connection with magnetic induction intensity B. Fig.3 suggests the width of airgap (δ) affects magnetic induction intensity B greatly in airgap, whereas it is inconspicuous toward the slope coefficient of saturated stage. The relationship between the width of airgap (δ) in saturated stage and magnetic induction intensity B in airgap is also non-linear, as shown in in Fig.4.
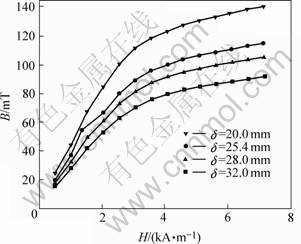
Fig.3 Relationship between magnetic induction intensity B and magnetization H with different width of airgap
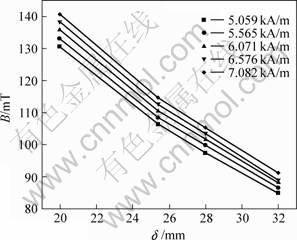
Fig.4 Relationship between magnetic induction intensity B and width of airgap δ
3.3 Relationship between magnetic induction intensity B in airgap and sectional area S of magnet
Another group of samples in experiment are selected. The sectional area S of magnet cores is sorted into 20 mm×20 mm, 27 mm×27 mm, 33 mm×33 mm, 41 mm×41 mm. The centerline perimeter L0 in magnet and the width of airgap are unchangeable, namely L0= 1 020 mm, δ= 20 mm. The changeable situation of magnetic induction intensity B in airgap center along with the magnetization H is measured. Fig.5 presents the curve based on magnetization H in connection with magnetic induction intensity B. Fig.5 also shows that magnet cores sectional area S has apparent influence on the magnetic induction intensity B in airgap.
With the magnet cores sectional area S reducing, the magnetic induction intensity B in airgap drops acutely. Moreover, magnet cores sectional area S has obvious influence on slope coefficient in saturated stage and reversible saturated stage of magnetization, and magnet cores sectional area S shifts to the higher value as the
slope coefficient increases. Magnetic induction intensity B in a certain magnetization and magnet cores sectional area S are selected to plot a relative curve, as shown in Fig.6. It’s clear that the relationship between magnet cores sectional area S and magnetic induction intensity B in airgap is also non-linear, and the magnet cores sectional area S has more significant influence on magnetic induction intensity B in airgap.
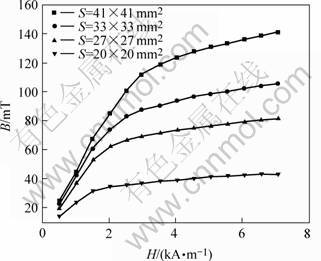
Fig.5 Relationship between magnetic induction intensity B and magnetization H with different magnet cores sectional area
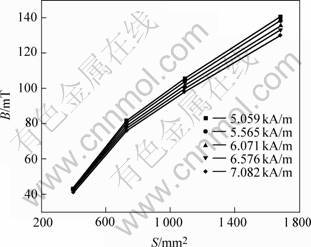
Fig.6 Relationship between magnetic induction intensity B in airgap and sectional area S
3.4 Relationship between magnetic induction intensity in clearance of magnet and centerline perimeter of magnet
Another four samples in experiment are selected. The sectional area S of magnet cores is 33 mm×33 mm, and the width of air gap is 20 mm. The centerline perimeter L0 in magnet is sorted into 820 mm, 920 mm, 1 020 mm and 1 120 mm. Fig.7 presents the curve based on magnetization H in connection with magnetic induction intensity B. According to the length of the centerline perimeter, each curve in magnet follows magnetization law, including initiative magnetization stage, irreversible magnetization stage and saturated magnetization stage, as shown in Fig.7. Compared with the curve of magnet core’s magnetization, the slope coefficient in irreversible magnetization stage is decreased; while the slope coefficient in saturated magnetization stage is increased. These result from dispersive magnetic field in airgap. Magnetic induction intensity B in a certain magnetization and the centerline perimeter L0 in magnet are selected to make out a relation curve, as shown in Fig.8. It is clear that the relationship between the centerline perimeter L0 in magnet and magnetic induction intensity B in airgap is non-linear.
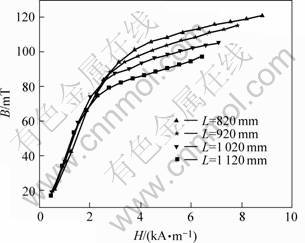
Fig.7 Relationship between magnetic induction intensity B and magnetization H with different centerline perimeter
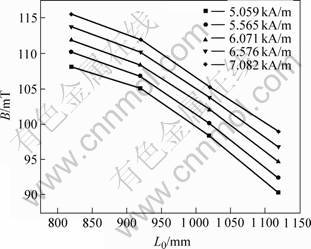
Fig.8 Relationship between magnetic induction intensity B and centerline perimeter L0 in magnet
3.5 Deduction of experience formula for calculating magnetic induction intensity B in airgap
It is considered that the relationship between the magnetic induction intensity B in airgap and the square root of the sectional area S of magnet cores is linear basically via analyzing the data received. In the same way, the relationship between the width of airgap δ and the nature logarithm of the magnetic induction intensity B in airgap (lnB) is also linear. Whereas the nature logarithm of the magnetic induction intensity B in
air gap and the ratio between the centerline perimeter L0 in magnet and the sectional area S of magnet cores form linear relations basically. Assuming relation formula of the magnetic induction intensity B in airgap and the magnetization H can be expressed as follows:
(1)
The influence of centerline perimeter in magnet cores, the length of uncork airgap δ and sectional area on the magnetic induction intensity B in airgap is synthesized to deduce experience calculation formula about magnetic induction intensity B of uncork magnet in airgap’s saturated stage by mathematics regression analysis[13]:
(2)
where H is the intensity of magnetic field, A/m; δ is the length of uncork airgap, mm; L is centerline perimeter of magnet cores, mm; S is the sectional area of magnet cores, mm2; B is the magnetic induction intensity B in airgap, mT; α, β and γ are attenuation coefficients and they are 0.045 29, 0.036 13(mm-1) and 0.821 1, respectively; L0, S0 and H0 are reference values which are 1 020 mm, 41 mm×41 mm and 7 082 A/m, respectively, and the constants, B0 and K, are 890 mT, 0.005 14 mT/(A·m-1), respectively.
3.6 Electromagnetism filling counter-gravity casting of turbo-charge blade wheel
The cast product of supercharger impeller is a typical product. The vane thickness is only 1-2 mm and the center is very thick, about d50-200 mm in a general way. The quality of product is exigent, and besides, gas hole and inclusion defects are unallowed. Working rotation rate is 30 000-7 000 r/min generally. It is hard to take shape for vane by use of ordinary pouring method. It is also difficult to insure tightknit in the center of vane. Gypsum type is adopted in this study. We use the small end of vane as gate. The turbo-charge blade wheels of engine in different types are developed triumphantly. The low pressure casting of electromagnetic pump that developed by us is used for producing the cast products, as shown in Fig.9. The casting processes include mold filling and dwell of electromagnetic pump. Compared with the traditional pneumatic low pressure casting, the system of low pressure casting equipment of electromagnetic pump eliminates the defects which are brought by fluctuation of fluid plane in filling process or aluminum fluid air admission when condensation air is passed or low control precision is in filling process. There are a number of advantages, including calm filling process, continuous flux, and adjustable precision. At the same time, the air admission quantity of aluminum fluid is small in continuous production and cast products are of good quality. The casts of supercharger impeller exterior are integrated and there are no defects on surface. Quality rate of the products can achieve 98% according to Q/BD33—1996 standard. Pinhole degree is in first level, the average of tensile strength σb is 345 MPa, and the relative ductility δ is 8.5%. The center of vane is corroded in low multiple and porosity is not found. Cast products have a compact structure, in which grain size is 0.85-2.00 mm.
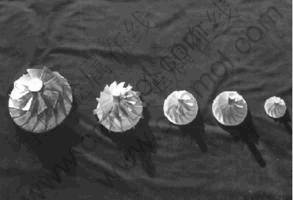
Fig.9 Turbo-charge blade wheels by electromagnetic pump low pressure casting
4 Conclusions
1) The theory of electromagnetic filling is formed based on the function of electric current and magnetic field in the conducting electricity fluid. The electromagnetic pump imposes the electromagnetic motivation force (Lorentz force) on the metal liquid directly and makes it move following a definite direction. So the magnetic induction intensity B is a primary parameter determining the ability of electromagnetic pump. The influence of centerline perimeter in magnet cores, the length of uncork airgap δ and sectional area on the magnetic induction intensity B in airgap is synthesized to deduce experience formula about magnetic induction intensity B of uncork magnet in airgap’s saturated stage by mathematics regression analysis.
2) Remarkable quality rate of the products can achieve 98% according to Q/BD33—1996 standard. Pinhole degree is in first level. The average tensile strength σb is 345 MPa and the relative ductility δ is 8.5%. The center of vane is corroded in low multiple and porosity is not found. Cast products have compact structure, in which the grain size is 0.85-2.00 mm. The quality of product cast by the electromagnetic filling low-pressure die casting is improved more significantly compared with the traditional pneumatic low-pressure casting.
References
[1] CAO Zhi-qiang, ZHANG Yong-an, JIN Jun-ze. The status of materials eletromagnetic-process and up-to-date development [J] . The Chinese Journal of Nonferrous Metals, 1997, 7(S1): 348-350. (in Chinese)
[2] KOVALEV L K. High output power reluctance electric motors with bulk HTS elements[J]. Superconductivity and Its Applications, 2003, 386(15): 419-423.
[3] MAO Da-heng, WANG Wei-juan, ZHONG Jue. Effects of electromagnetic field on aluminum alloys liquid-solid continuous rheological structure evolution [J]. Materials Science and Engineering A, 2004, 385: 22-30.
[4] Smith R A. The cosworth process[J]. Casting World, 1986(3): 31-38.
[5] The investigation overview of the present status of casting for China Armament Industries Group Corporation to USA[R]. Foundry, 1998: 40-44. (in Chinese)
[6] HOU Ji-bo, HUO Li-xing, PENG You-gen. Experimental investigation of technologic parameters of electromagnetic pump used for low-pressure casting[J]. Foundry, 2003(8): 623-625. (in Chinese)
[7] DANG Jing-zhi, YANG Jing, CENG Jun. A study on low pressure casting technology of aluminum alloy applying electromangetic pump [J]. Acta Armamentarii, 2003(2): 286-288.
[8] YANG Jing, HOU Ji-bo, TANG Hai-ping. Calculation of flow rate of the electromagnetic pump for aluminum alloy pouring[J]. Special Casting and Nonferrous Alloys, 2003(1): 50-51. (in Chinese)
[9] LI Dong-yun. The Study on the Key Technology of Electromagnetic Pump [D]. Taiyuan: North University of China, 2001. (in Chinese)
[10] YANG Jing, DANG Jing-zhi, PENG You-gen, Cheng Jun. Study on low pressure casting technology of the turbo-charge blade wheel by electromagnetic pump [J]. Foundry, 2003(8): 632-634. (in Chinese)
[11] BAN Chun-yan, BA Qi-xian, CUI Jian-zhong, ZENG Gui-yi. Effect of magnetic field during solidification on microstructure [J]. Journal of Northeastern University Natural Science, 2002, 23(8). (in Chinese)
[12] The mechanical graduate school of Chinese Academy of Sciences Electromagnetic pump group in graduate school of Shanghai electrical equipment. Electromagnetic Pump of Liquid State Metal [M]. Beijing: Science Press, 1979. 3-15.
[13] HAO Hong-wei, SHI Guang-kan. Example Windows Tutorial of Origin6.0 [M]. Beijing: Electric Power Press, 2000. 8. (in Chinese)
(Edited by YANG Bing)
Foundation item: Project (20031043) supported by the Natural Science Foundation of Shanxi Province, China
Corresponding author: YANG Jing; Tel: +86-351-3921884; E-mail: yangjing@nuc.edu.cn