Trans. Nonferrous Met. Soc. China 24(2014) 1196-1201
Removal of manganese from waste water by complexation-ultrafiltration using copolymer of maleic acid and acrylic acid
Yun-ren QIU1,2, Lian-jun MAO1, Wei-hua WANG1
1. School of Chemistry and Chemical Engineering, Central South University, Changsha 410083, China;
2. Key Laboratory of Resources Chemistry of Nonferrous Metals, Ministry of Education, Changsha 410083, China
Received 8 May 2013; accepted 21 October 2013
Abstract: Copolymer of maleic acid and acrylic acid (PMA-100), combining with polyvinyl butyral (PVB) ultrafiltration membrane was used for the removal of Mn(II) from waste water by complexation-ultrafiltration. The carboxylic group content of PMA-100 and the rate of complexation reaction were measured. Effects of the mass ratio of PMA-100 to Mn(II) (n), pH, background electrolyte, etc on the rejection rate (R) and permeate flux (J) were investigated. The results show that carboxylic group content of PMA-100 is 9.5 mmol/g. The complexation of Mn(II) with PMA-100 is rapid and completed within 5 min at pH 6.0. Both R and J increase with pH increasing in the range of 2.5-7.0, and R increases with the increase of n at pH 6.0 while J is little affected. The background electrolyte leads to the decrease of R, and CaCl2 has much greater effect on R than NaCl at the same ionic strength.
Key words: complexation-ultrafitration; copolymer of maleic acid and acrylic acid; poly (vinyl butyral) hollow fiber membrane; manganese; wastewater treatment
1 Introduction
Manganese is a common contaminant of mine water and other waste waters, and it can gradually be oxidized to insoluble manganic dioxide causing several problems such as water discoloration, metallic taste, odor, turbidity, biofouling and corrosion, staining of laundry. Most common methods to remove Mn(II) are oxidation and precipitation [1,2]. Complexation-ultrafiltration, also described as polymer enhanced ultrafiltration (PEUF) or polymer assisted ultrafiltration (PAUF), is one of the most promising methods in treatment of the wastewater containing heavy metals with significant advantages, like low-energy requirements, high removal efficiency and high selectivity of separation if selective bonding agents are applied [3,4]. In that process, the metals are firstly bound to polymers to form macromolecular complex and rejected by membrane, whereas unbound metals pass through the membrane [5].
Complexation-ultrafiltration process has been successfully used for the treatment of wastewater containing kinds of metal ions such as Co2+ [3,4], Pb2+ [4], Cu2+ [3,5,6], Cd2+ [7], Zn2+, Ni2+ [8,9] and Hg2+ [10]. The selection of water soluble polymer is of significance in complexation-ultrafiltration process, and the natural character directly decides the efficiency of combination with target metal ions and the operation conditions required. In the previous studies, polyethyleneimine (PEI) [5,9-11] and polyacrylic acid (in its sodium PAAS or hydrogen form PAA) [8,9, 12] were most widely used. Copolymer of maleic acid and acrylic acid (PMA-100), a copolymer made of maleic acid and acrylic acid with abundant carboxyl functional groups, as shown in Fig. 1, can be used as complexing agent in complexation- ultrafiltration process [13], but has not been deeply studied as water-soluble polymer in complexation- ultrafiltration process. The high content of the carboxyl group is a significant feature and possibly corresponding to a high capacity of binding metal ions [6,14].
Complexation-ultrafiltration was firstly used for the removal of manganese from simulated waste water by using copolymer of maleic acid and acrylic acid (PMA-100) and polyvinyl butyral (PVB) hollow fiber ultrafiltration membrane. Acid-base property of the polymer was analyzed by potentiometric and conductimetric titrations, and the effects of polymer/ metal ratio and pH etc on both rejection rate and permeate flux were studied. In addition, a comparative study of sodium chloride and calcium chloride on the effect of removal rate of manganese was also carried out.
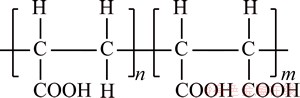
Fig. 1 Structure of PMA-100
2 Experimental
2.1 Chemicals and membrane
Copolymer of maleic acid and acrylic acid (PMA-100), with the relative molecular mass 50 kDa, supplied by Shenyang Xingqi Daily Chemicals Plant (China), was previously pretreated to eliminate the small molecular mass fractions. MnSO4·H2O was used as sources of Mn2+. The pH of solution was adjusted by adding hydrochloric acid (0.1 mol/L) or sodium hydroxide (0.1 mol/L). All the solutions in the experiment were prepared with deionized water.
Polyvinyl butyral (PVB) hollow fiber membrane was produced via thermally induced phase separation method [15] and its main parameters are listed in Table 1.
Table 1 Main parameters of PVB hollow fiber ultrafiltration membrane
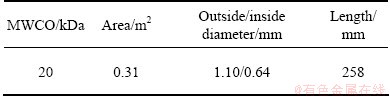
2.2 Ultrafiltration experiment
The UF experiment in laboratory-scale was carried out with the apparatus shown in Fig. 2. An initial feed of 2.5 L was introduced to the feed tank and was circulated through the apparatus. Flow rate of 40 L/h and operative pressure of 40 kPa were controlled. The temperature was kept at 27 °C with the help of thermostatic water batch. In the total recirculation process, both the permeate and retentate stream were returned to the feed tank so that the concentration of feed was constant. The sample of permeate was taken for analysis.
The concentration of the permeate was analyzed by atomic absorption spectroscopy (Shi-Madzu AA-670) and the permeate flux was measured by weighing the permeate volume produced in a certain quantity of time.
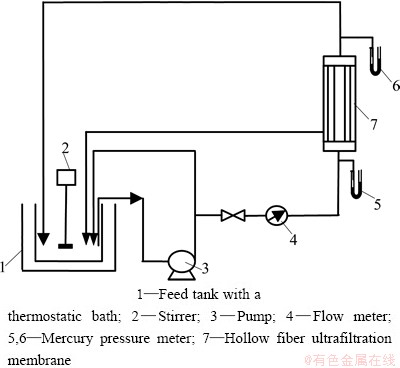
Fig. 2 Scheme of experimental apparatus
2.3 Measurement of concentration of carboxylic group
15.0 mg of PMA-100 was precisely weighed and dispersed in 250 mL of deionized water (final concentration was 60 mg/L), and 0.1 mol/L HCl was added in the beginning of the experiment to lower the pH to approximately 3. Titrations were carried out by stepwise addition of 0.25 mL of 0.1 mol/L NaOH (standardized by potassium acid phthalate) to the flask placed in thermostated cell (27 °C) while the suspension was stirred under a nitrogen atmosphere. The conductivity was measured using a conductivity meter (DDS-11A, Lei-Ci) and pH of solution was recorded with a pH meter (PHS-3C, from Shanghai Precision & Scientific Instrument Co. Ltd, China). Conductivity and pH values were recorded after stabilization. The concentration of the carboxylic group can be calculated by
(1)
where Cp,COOH is the content of carboxylic group (mmol/g); c is the molar concentration of NaOH added into the titration system (mol/L); V is the titrant volume up to equivalence point (mL); mp is the mass of the polymer (g).
3 Results and discussion
3.1 Potentiometric and conductimetric titrations of polymer
The titration curves are shown in Fig. 3(a). Conductivity initially decreases sharply due to the neutralization of free protons coming from hydrochloric acid while solution pH slowly increases because of consumption of hydrochloric acid. When all of hydrochloric acid is neutralized, the weak acidic group-carboxyl group begins to dissociate and the plateau is observed. During the transitional stage, pH increases sharply with the addition of sodium hydrate, even though weak acid group exists which can hardly change the trend. After the neutralization of weak acids, conductivity begins to increase sharply while the increase of pH becomes rather softly as a result of the excess of sodium hydroxide.
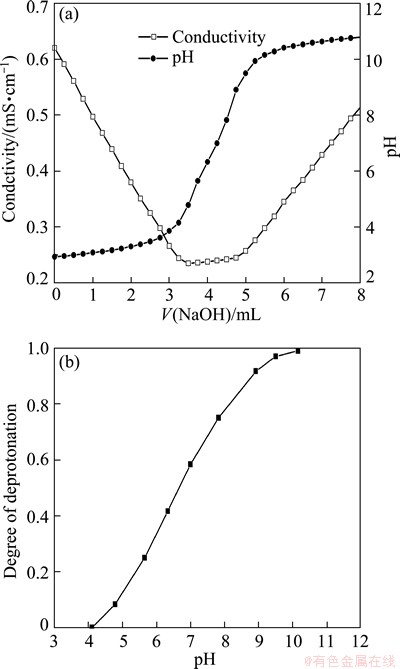
Fig. 3 Titrations of PMA-100 (a) and degree of deprotonation of PMA-100 vs pH (b)
Titration curves provide ways to estimate the concentration of the carboxylic group which is 9.5 mmol/g. The degree of deprotonation of carboxylic group, defined as the number of neutralized acrylic acid functional groups over the total number of functional groups, as a function of solution pH was plotted in Fig. 3(b). It is shown that the degree of deprotonation at a given polymer concentration (60 mg/L) increases with increasing the pH of solution. When the pH is higher than 3.8, the carboxyl groups begin to ionize and negatively charged. At pH 9.2, all carboxyl groups are ionized.
3.2 Total recirculation process
3.2.1 Rate of Mn(II) complexation
The complexation rate was investigated at pH=6.0 and the mass ratio of PMA-100 to Mn(II) of 6, where ρMn(II) is the mass concentration in the permeate, mg/L. From Fig. 4, it is found that the mass fraction of Mn(II) in the permeate rapidly decreases, and keeps constant after 5 min with a residual mass fraction below 0.1 mg/L. However, 30 min was proposed for each experiment, because the steady-state of permeate flux was also considered, which was attained in 30 min proved in the following experiments.
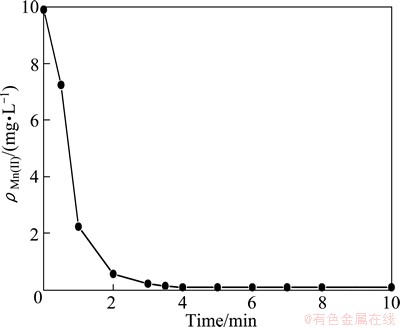
Fig. 4 Change of mass concentration of Mn(II) in permeate with time
The complexation reaction between the Mn2+ and PMA-100 can be explained as follows: PMA-100 will dissociate in aqueous solution, and the solution is acidic, as shown in reaction (1). This can also be seen in Fig. 3(a). As pH increases, the degree of deprotonation of carboxylic group increases, as shown in Fig. 3(b), and more ionized carboxylic groups are available to bind Mn2+, as shown in reaction (2).
—CH2—COOH
[—CH2—COO]–+H+ (1)
2[—CH2—COO]–+Mn2+
[—CH2—COO]2Mn (2)
3.2.2 Effect of pH
The effects of pH on rejection rate (R) of Mn(II) and permeate flux (J) at different mass ratios are shown in Figs. 5 and 6. It is observed that pH significantly affects R. When pH varies from 2.5 to 7.0, R increases from 10% to almost 100% for mass ratio 8 and 2% to 80% for mass ratio 2. In the pH range of 2.5 to 3.0, R is low and increases very slightly. This result could be explained by Fig. 3(b), when pH is lower than 3.8, all sites of polymer-carboxylic groups are protonated (—COOH), and metal-polymer complexes could be hardly formed. At pH 3.8, the carboxylic groups start to ionize (—COO-) and are able to form complexes in the presence of metal ions. As pH continues to increase, the degree of deprotonation of carboxylic group increases and more ionized carboxylic groups are available to bind metal ions.
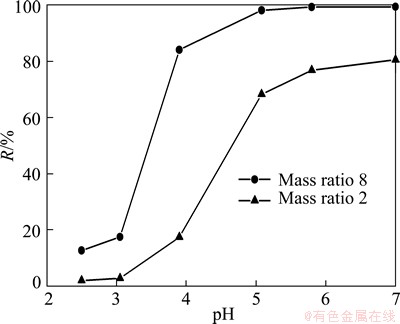
Fig. 5 Effect of pH on rejection rate for different mass ratios of PMA-100 to Mn(II) at 40 kPa
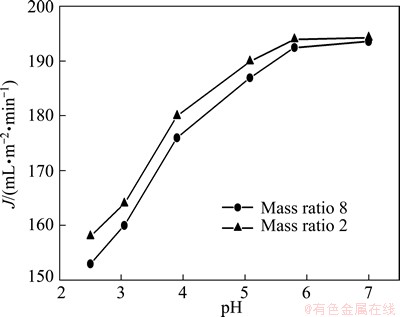
Fig. 6 Effect of pH on permeate flux for different mass ratios of PMA-100 to Mn(II) at 40 kPa
In Fig. 6, J gradually increases with pH increasing, which agrees well with the previous study [16]. It can be explained that as pH increases, the carboxyl groups in PMA-100 molecules are deprotonated and polarized carboxyl groups repulse each other, which stretches polymer chains to the maximum extent and makes them linear and rigid, so membrane fouling decreases [16]. When pH is higher than 6.0, J does not continue to increase. Though no experiment was carried out, we can imagine that J will further decrease as pH is higher than 7.0 considering the formation of hydroxide precipitation. So pH 6.0 is chosen to be an optimal value.
3.2.3 Effect of mass ratio of polymer to Mn(II) (n)
The effect of mass ratio of polymer to Mn(II) (n) was studied under pH 6.0. The results in Fig. 7 show that increasing n improves the removal efficiency of Mn(II), and as n value is above 6, the highest rejection rate is obtained with 99.6%. So the capacity of PMA-100 for Mn2+ is about 0.17 g/g at pH 6.0.
The steady state permeate flux is almost constant as n increases from 2 to 12. This result is different from some works [17,18], in which the flux declined as the polymer concentration increased. It is explained by a fact that polymer concentrations in these work were much higher, 0.4% to 2% (w/w) [17] and 1% to 3% [18], while the polymer concentration was just 20-120 mg/L (equivalent to 0.002% to 0.012% (w/w)) in our research. Such low concentration of polymer would not lead to the fluctuation of solution viscosity and the reduction of permeate flux.
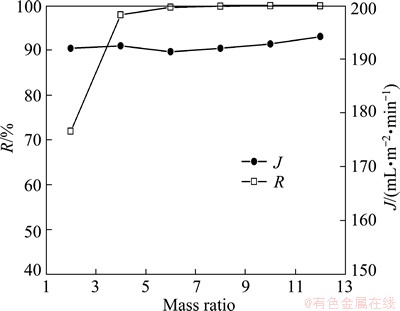
Fig. 7 Effect of for mass ratios of PMA-100 to Mn(II) on R and J at 40 kPa
From the point of economy, the optimal for mass ratios of PMA-100 to Mn(II) is set at 6. Sometimes a little higher for mass ratios of PMA-100 to Mn(II) value is adopted to ensure adequate rejection and will not lead to a decline of flux in practical industrial application.
3.2.4 Effect of operation time on permeate flux
Once the optimal conditions have been determined, experiments were carried out to study the permeate flux decline phenomenon in the total recirculation process with time. The results are shown in Fig. 8. It can be seen that the permeate flux decreases with time and becomes stable after about 20 min. The declination of permeate flux is 2.0%.
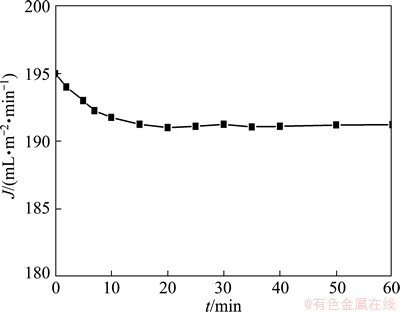
Fig. 8 Declination of J at 40 kPa
3.2.5 Effect of background electrolyte
In the real waste water treatment, there are always some dissolved electrolytes, especially in the treatment of hard water. To evaluate the effect of background electrolyte, experiments were carried out with the addition of NaCl or CaCl2 with ionic strength (I) range of 1.5-6.0 mmol/L. The rejection rate is decreased in the absence of the background electrolyte as shown in Fig. 9, and CaCl2 has a greater effect than NaCl. The rejection rate is 80% with NaCl whereas it is 8% with CaCl2 at ionic strength of 6.0 mmol/L. It has been confirmed that the effect of NaCl was due to the compressing electric double layer rather than the competitive complexation [16]. So it can be inferred that the effect of CaCl2 on Mn(II) rejection is mainly due to the competing complexation between Ca(II) and Mn(II).
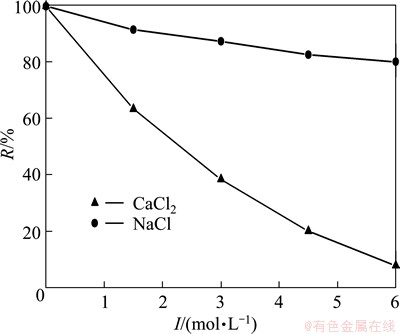
Fig. 9 Effect of background electrolyte on R
4 Conclusions
1) Manganese is removed from aqueous solution by complexation-ultrafiltration process using copolymer of maleic acid and acrylic acid as complexing agent. The complexation process is rapid and completed in 5 min. Under optimal conditions of pH 6.0 and mass ratio of polymer to Mn(II) of 6, the rejection rate of Mn(II) is 99.6% and the declination of the permeate flux is only 2% in the total recirculation process.
2) Potentiometric and conductimetric titrations of the copolymer of maleic acid and acrylic acid reveal that carboxylic group content is 9.5 mmol/g and the degree of deprotonation increases as pH increases.
3) The existence of background electrolyte leads to a decrease of the rejection rate of Mn(II) and CaCl2 leads to a more significant decrease in rejection rate than NaCl, mainly due to the competing complexation between Ca(II) and Mn(II).
References
[1] BAMFORTH S M, MANNING D A C, SINGLETON I, YOUNGER P L, JONSON K L. Manganese removal from mine waters— Investigating the occurrence and importance of manganese carbonates [J]. Appl Geochem, 2006, 21(8): 1274-1287.
[2] ROCCARO P, BARONE C, MANCINI G, VAGLIASINDI F G A. Removal of manganese from water supplies intended for human consumption: A case study [J]. Desalination, 2007, 210(1-3): 205-214.
[3] JUANG R S, SHIAU R C. Metal removal from aqueous solutions using chitosan-enhanced membrane filtration [J]. J Membr Sci, 2000, 165: 159-167.
[4] RETHER A, SCHUSTER M. Selective separation and recovery of heavy metal ions using water-soluble N-benzoylthiourea modified PAMAM polymers [J]. React Funct Polym, 2003, 57(1): 13-21.
[5] MOLINARI R, GALLO S, ARGURIO P. Metal ions removal from wastewater or washing water from contaminated soil by ultrafiltration-complexation [J]. Water Res, 2004, 38: 593-600.
[6] MURPHY V, HUGHES H, MCLOUGHLIN P. Cu(II) binding by dried biomass of red, green and brown macroalgae [J]. Water Res, 2007, 41: 731-740.
[7] QIU Yun-ren, GAO Guo-ying, LIU Min. Removal of cadmium from wastewater by complexation-ultrafiltration process [J]. The Chinese Journal of Nonferrous Metals, 2011, 21(8): 2012-2016. (in Chinese)
[8] BORBELY G, NAGY E. Removal of zinc and nickel ions by complexation-membrane filtration process from industrial wastewater [J]. Desalination, 2009, 240: 218-226.
[9] CANIZARES P, LUCAS, A D, PEREZ A, CAMARILLO R. Effect of polymer nature and hydrodynamic conditions on a process of polymer enhanced ultrafiltration [J]. J Membr Sci, 2005, 253(1-2): 149-163.
[10] BARRON-ZAMBRANO J, LABORIE S, VIERS P, RAKIB M, DURAND G. Mercury removal and recovery from aqueous solutions by coupled complexation-ultrafiltration and electrolysis [J]. J Membr Sci, 2004, 229(1-2): 179-186.
[11] ZAMARIOTTO D, LAKARD B, FIEVET P, FATIN-ROUGE N. Retention of Cu(II)- and Ni(II)- polyaminocarboxylate complexes by ultrafiltration assisted with polyamines [J]. Desalination, 2010, 258(1-3): 87-92.
[12] ZENG Jian-xian, YE Hong-qi, HU Zhong-yu. Application of the hybrid complexation-ultrafiltration process for metal ion removal from aqueous solutions [J]. Journal of Hazardous Materials, 2009, 161: 1491-1498.
[13] KHAIDAR M S, LABANDA J, LLORENS J. Recovery of chromium(III) by polymer enhanced ultrafiltration [J]. Chem Eng Trans, 2007, 11: 179-184.
[14] COJOCARU C, TRZNADEL G Z, JAWORSKA A. Removal of cobalt ions from aqueous solutions by polymer assisted ultrafiltration using experimental design approach. Part 1: Optimization of complexation conditions [J]. J Hazard Mater, 2009, 169: 599-609.
[15] QIU Y R, MATSUYAMA H. Preparation and characterization of poly poly(vinyl butyral) hollow fiber membrane via thermally induced phase separation with diluent polyethylene glycol 200 [J]. Desalination, 2010, 257: 117-123.
[16] CANIZARES P, PEREZ A, CAMARILLO R, MAZARRO R. Simultaneous recovery of cadmium and lead from aqueous effluents by a semi-continuous laboratory-scale polymer enhanced ultrafiltration process [J]. J Memb Sci, 2008, 320(1-2): 520-527.
[17] CAMARILLO R, LIANOS J,
P. Treatment of copper (II)-loaded aqueous nitrate solutions by polymer enhanced ultrafiltration and electrodeposition [J]. Sep Purif Technol, 2010, 70(3): 320-328.
[18] CAMARILLO R, PEREZ A, CANIZARES P, LUCAS A D. Removal of heavy metal ions by polymer enhanced ultrafiltration Batch process modeling and thermodynamics of complexation reactions [J]. Desalination, 2012, 286(1): 193-199.
马来酸-丙烯酸共聚物络合超滤处理含锰废水
邱运仁1,2,毛廉君1,王伟华1
1. 中南大学 化学化工学院,长沙 410083;
2. 中南大学 有色金属资源化学教育部重点实验室,长沙 410083
摘 要:用马来酸-丙烯酸共聚物(PMA-100)作络合剂,采用PVB中空纤维膜,研究络合-超滤处理含锰废水。测定了高聚物的羧基含量以及Mn(II)与PMA-100的络合反应速率,研究聚合物与重金属质量比(P/M)、pH、外加盐浓度等因素对锰离子截留率(R)和膜通量(J)的影响。结果表明:高聚物中羧基含量为9.5 mmol/g,对Mn(II)质量浓度为10 mg/L的模拟废水,在pH=6.0时,Mn(II)与PMA-100的络合在5 min内基本可完成,络合率达99.6%。在一定P/M下,pH在2.5~7.0范围内,截留率和膜通量均随着pH的增加而增大;在一定pH下,截留率随着P/M的增加而增加,但膜通量基本不变。外加盐的存在使截留率下降,在同样离子强度下,CaCl2的影响远大于NaCl的。
关键词:络合-超滤;马来酸-丙烯酸共聚物;PVB中空纤维膜;锰;废水处理
(Edited by Hua YANG)
Foundation item: Project (21176264) supported by the National Natural Science Foundation of China; Project (11JJ2010) supported by Hunan Provincial Natural Science Foundation of China; Project (LC13076) supported by Undergraduate Innovation Foundation of Central South University, China
Corresponding author: Yun-ren QIU; Tel: +86-731- 88879616; E-mail: qiuyunren@gmail.com, csu_tian@csu.edu.cn
DOI: 10.1016/S1003-6326(14)63179-4