
Electrochemical formation of holmium-copper alloys on copper cathode in molten KCl-HoCl3
SU Yu-zhi(苏育志)1, YANG Qi-qin(杨绮琴)2, LIU Guan-kun(刘冠昆)2
1. School of Chemistry and Chemical Engineering, Guangzhou University,
Guangzhou 510006, China;
2. School of Chemistry and Chemical Engineering, Zhongshan University,
Guangzhou 510275, China
Received 20 October 2005; accepted 28 March 2006
Abstract: Cyclic voltammetry, open circuit potential—time curve after potentiostatic electrolysis and potential step chronoamperometry were used to investigate the electrochemical formation processes of holmium-copper alloys on copper cathode in molten HoCl3-KCl. Intermetallic compounds HoCu5, HoCu4, HoCu2 and HoCu are formed in sequence and then the metallic Ho is deposited when Ho3+ is reduced on copper electrode in molten KCl-HoCl3 at 1 066 K. The first charge-transfer reaction is reversible. The structure of holmium-copper alloy film deposited on copper electrode by potentiostatic electrolysis was characterized by X-ray diffraction. The standard free energies of formation for the intermetallic compounds HoCu5, HoCu4, HoCu2 and HoCu are -95.5, -92.6, -73.8 and -44.0 kJ/mol, respectively. The diffusion coefficient and diffusion activation energy of Ho atom in the alloy are estimated to be 10-10-10-11 cm2/s and 75.35 kJ/mol, respectively, from the chronoamperometry data.
Key words: holmium-copper alloy; electrochemical formation; free energy of formation; diffusion coefficient
1 Introduction
Alloys of rare earth Ho with other metals (Fe, Co, Ni, Cu, etc) possess electrical, magnetic and other properties important for modern technology[1-4]. LI and ZHU[5] proposed a new processing technology for high property permanent magnets. Holmium is an active element and can not be deposited from aqueous solution, but it can be prepared by molten salt electrolysis at high temperature. If Ho-alloy is deposited in molten salts on a consumable cathode, the operation temperature may be decreased. Codeposition process of Ho and Co was recently investigated in organic solvent by WANG et al[6].
In this work, we study the electrode process of Ho3+ reduction on copper electrode in molten chlorides. The free energies of formation for the intermetallic compounds of Ho-Cu systems, the diffusion coefficients and the diffusion activation energy of Ho in the alloy are determined.
2 Experimental
Dehydrated HoCl3 was obtained by the reaction of Ho2O3 (99.99%) with NH4Cl (A.R.) at 623 K. KCl was dehydrated in vacuum at 673 K. A molten mixture of the dehydrated KCl and HoCl3 was used as electrolyte. The working electrode was a copper or tungsten electrode, the auxiliary electrode was a glass carbon electrode, and the reference electrode was Ag/AgCl (x=0.1) electrode. All potentials measured in this paper are converted to the values versus the Cl2/Cl- electrode[7]. The electro- chemical measurements were carried out in a quartz electrolytic cell under argon atmosphere by using a potentiostat (HDV-7B), a functional generator (DCD-3) and an x-y recorder (3086x-y). The silicon-controlled power unit (KG-6A) and the temperature regulator (WT-710) were used to control the temperature. The concentration of HoCl3 in molten salts was analyzed by EDTA complexometry. The phase of surface alloy layer on working electrode was analyzed by XRD.
3 Results and discussion
3.1 Electrode processes
The cyclic voltammogram of a tungsten electrode in molten KCl is shown in Fig.1(a). The potassium deposition is at about -3.15 V. Addition of HoCl3 to the molten KCl is resulted in Fig.1(b), in which two reduction peaks start at -3.01 and -3.16 V, respectively. The first reduction peak is due to the reduction of Ho3+ to Ho metal and the second reduction peak corresponds to the deposition of potassium metal.
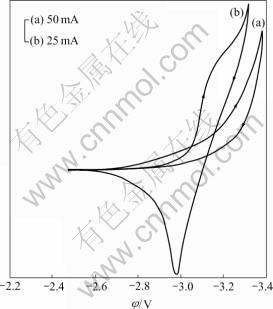
Fig.1 Cyclic voltammogram of tungsten electrode (0.31 cm2) at 1 063 K, 60 mV/s in KCl (a) and KCl-HoCl3 (2.44 %, mole fraction) (b)
The cyclic voltammogram of copper electrode in the molten KCl-HoCl3 at 1 066 K is shown in Fig.2. Six reduction peaks which start at -2.70, -2.75, -2.85, -2.95, -3.02 and -3.14V are observed. In contrast to the first reduction peak in Fig.1(b), it can be found that the last two reduction peaks in Fig.2 corresponds to the deposition of pure metallic holmium and potassium, respectively[8]. Fig.3 corresponds to the voltammograms of the first reduction peak in Fig.2 at different scan rates. The reduction peak potentials φp, c and the oxidation peak potentials φp, a are almost independent on the scan rate. These results indicate that the first reduction reaction is reversible. Furthermore, from the average value of |φp, c- φp, a|, 0.066 V, the electron transfer number is obtained as n=3.2, according to |φp,c-φp,a|=2.3RT/nF[9]. These results indicate that the electrode reaction of the first reduction peak is three-electron transfer reaction. Furthermore, the starting potential evidently is more positive than the potential of Ho3+/Ho, owing to the existence of depolarization[10]. It is inferred that the first reduction peak starting at about -2.70 V is caused by the formation of the intermetallic compound of Ho-Cu, Ho3++3e+nCu= HoCun, and the 2nd, 3rd, 4th and 5th reduction peaks, which start at about -2.75, -2.85, -2.95 V and -3.02 V, respectively, may be related to the formation of Ho-Cu intermetallic compounds too. Thus four intermetallic compounds are formed before Ho3+ ions are reduced to pure Ho metal.
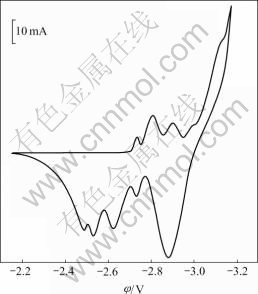
Fig.2 Cyclic voltammogram of Cu electrode (0.47 cm2) in KCl-HoCl3 (2.16 % mole fraction, scan rate 10 mV/s, T=1 066 K)
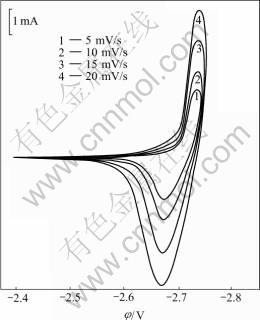
Fig.3 Cyclic voltammograms of first reduction peak in Fig. 2 at different scan rates (T=1 066 K)
The open current potential—time curve shown in Fig.4 is obtained after electrolysis at a constant potential of -3.10 V for 30 s at 1 066 K. Compared with cyclic voltammogram of Fig.2, it can be found that five plateaus at -2.70, -2.75, -2.84, -2.94 and -3.03 V in Fig.4 correspond to the five peaks starting at -2.70, -2.75, -2.85, -2.95 and -3.02 V, respectively, in Fig.2.
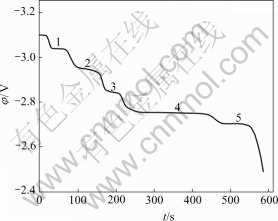
Fig.4 Potential—time curve of KCl-HoCl3 (2.16%, mole fraction) on copper electrode (0.47 cm2) after electrolysis at constant potential of -3.10 V for 30 s (T=1 066 K)
3.2 Alloy phase analysis of surface layer
There are four intermetallic compounds in the Ho-Cu system, which are HoCu5, HoCu4, HoCu2 and HoCu. In order to determine the composition of copper electrode surface alloy, controlled potential electrolysis is carried out on copper cathode at -2.74 V (the first reduction peak) at 1 114 K for 30 min in molten KCl- HoCl3. After removing the salts, the copper electrode is analyzed by XRD and the result is shown in Fig.5, which indicates that the phase of the electrode surface alloy is HoCu5.
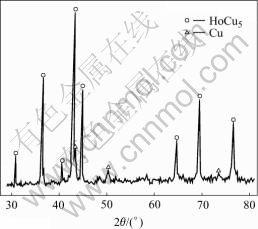
Fig.5 XRD patterns of Cu electrode surface after electrolysis at -2.74 V for 30 min at 1 114 K
The overall process of Ho3+ reduced on the electrode to form the intermetallic compounds includes the following steps: diffusion of Ho3+ to the surface of copper cathode, reduction of Ho3+ to Ho, diffusion of Ho into the copper cathode and formation of intermetallic compounds with copper. The higher the Ho content in the compound, the lower the reduced potential necessary to reduce Ho3+.
At first HoCu5 is formed, and then HoCu4 is formed when Ho3+ is reduced on copper cathode. From the results of cyclic voltammetry, open circuit potential— time curve after potentiostatic electrolysis and XRD, it is concluded that five reduction peaks at -2.70, -2.75, -2.85, -2.95 and -3.02V in Fig.2 are related to HoCu5, HoCu4, HoCu2, HoCu and Ho, respectively.
3.3 Free energies of formation for Ho-Cu inter- metallic compounds
Five plateaus at -2.70, -2.75, -2.85, -2.95 and -3.02 V in Fig.4 correspond to HoCu5, HoCu4, HoCu2, HoCu and Ho, respectively. These plateaus are due to the formation of Ho-Cu intermetallic compounds and the diffusion of Ho deposited on copper electrode surface into the copper electrode. The potential difference between the first plateau and the fifth plateau is related to the electromotive force of the cell as follows:
Ho | KCl-HoCl3 | HoCu5, Cu
The electrode reaction is
Ho=Ho3++3eHo3++3e+5Cu=HoCu5
The cell reaction is
Ho+5Cu=HoCu5
This is the reaction of HoCu5 formation, and for the above cell:
=-nFφ1=-nF[
-(RT/nF)ln
] (1)
where
is the free energy of formation for HoCu5, n is the number of electrons transferred in the reaction: Ho+5Cu=HoCu5, F and R are Faraday constant and universal gas constant respectively, φ1 and
are the potential difference and the standard potential difference for above cell respectively,
, aHo and aCu are the activities of HoCu5, Ho and Cu respectively.
HoCu5, Ho and Cu are all solid and have unit activity, so
(2)
where
is the standard free energy of formation for HoCu5. The potential differences between other plateaus and the first plateau responded to the electromotive force of the cells are listed respectively as follows:
Ho | HoCl3-KCl | HoCu5, Cu (
)
Ho | HoCl3-KCl | HoCu4, Cu (
)
Ho | HoCl3-KCl | HoCu2, Cu (
)
Ho | HoCl3-KCl | HoCu, Cu (
)
The cell reactions listed are respectively as follows:
Ho+5Cu = HoCu5
Ho+4Cu = HoCu4
Ho+2Cu = HoCu2
Ho+Cu = HoCu.
The standard free energies of formation for HoCu5, HoCu4, HoCu2 and HoCu are shown in Table 1.
Table 1 Standard free energies of formaition for Ho-Cu alloys(1 114K)

3.4 Diffusion coefficients and diffusion activation energy of Ho in HoCu5
Fig.6 shows the current-response curve at different potential steps from steady potential to -2.74 V, at which HoCu5 is formed on copper electrode in molten KCl-HoCl3. The diffusion coefficients of Ho into HoCu5 can be calculated from the current—time curve in Fig.7 according to the Eqn.(3)[11]:
D=(1/2τ)(QeM/xnFAρ)2 (3)
where M and ρ are molecular mass and density, respectively, of a intermetallic compound, xn is the number of electrons transferred in the reaction: Ho3++3e +5Cu=HoCu5, F is Faraday constant, A is electrode area, Qe is electric quantity consumed to get a steady state for the formation of intermetallic compounds and τ is time for a steady state. The diffusion coefficients D of Ho atoms into HoCu5 are shown in Table 2. The relationship between lnD and (1/T) is approximately linear. It is inferred from the slope of the linear plot of lnD against (1/T) that the temperature dependence of diffusion coefficient can be expressed as
D=2.37×10-6 exp(-9062/T) (4)
or
D=2.37×10-6 exp(-75.34×103/RT) (5)
where the diffusion activation energy of Ho in HoCu5 is 75.34 kJ/mol.
Table 2 Diffusion coefficients of Ho in HoCu5
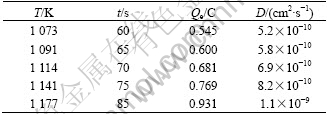
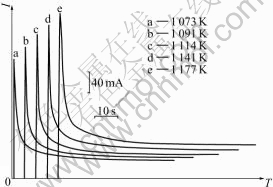
Fig.6 Potential-step current—time curves of Cu electrode (0.35 cm2) in molten KCl-HoCl3 (1.86% mole fraction) at different temperatures
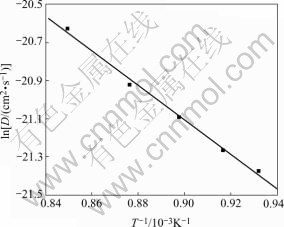
Fig.7 Plot of relation of lnD and 1/T
From previous and present investigation[12-14], we note that the overall processes of Ho3+ reduction on a cathode to form alloys include the following steps:diffusion of Ho3+ ions in molten KCl-HoCl3 to the surface of the cathode, reduction of Ho3+ to Ho, diffusion of Ho atoms into copper cathode and formation of a intermetallic compounds. When the process of formation of the intermetallic compound is the rate determining step, there exists a linear relation between current and potential[15]. But we do not find this feature. The diffusion coefficient of Ho3+ in molten KCl-HoCl3 at 1 071 K is 0.92×10-5 cm2/s[8], however, the diffusion coefficients of Ho3+ into HoCu5 are in the range from 10-10 to 10-11 cm2/s which are several orders of magnitude smaller than that of Ho3+ in molten KCl-HoCl3 at the same temperature range. Hence, the diffusion step of Ho atom into cathode may be the rate-determining step of the electrode process.
4 Conclusions
Intermetallic compounds HoCu5, HoCu4, HoCu2 and HoCu are formed in sequence and then the metallic Ho is deposited when Ho3+ is reduced on copper electrode in molten KCl-HoCl3 at 1 066 K. The first charge-transfer reaction is reversible. The diffusion of Ho atoms into the cathode is the rate-determining step of the cathodic process. The standard free energies, diffusion coefficients and diffusion activation energy of Ho atom in the alloy phase are determined.
References
[1] CWIK J, PALEWSKI T, NENKOV K, BURKHANOV G S. Magnetic, electrical, and thermodynamic properties of the LaxHo1-xNi2 solid solutions [J]. Journal of Alloys and Compounds, 2005, 399(1-2): 7.
[2] JINHAI C, TIMOTHY C, STEIMLE C L. The permanent electric dipole moment of holmium monoxide, HoO[J]. Journal of Molecular Spectroscopy, 2005, 232(1): 105.
[3] MOZHARIVSKYJ Y, KUZMA B Y. Ternary phosphide Ho2Cu6-xP5-y, its crystal structure, and REm+n(Cu2P3)m(Cu4P2)n relationship with other rhombohedral rare-earth copper phosphides [J].Journal of Solid State Chemistry, 2000, 151(1): 150.
[4] VERT R, BOUOUDINA M, FRUCHART D, GIGNOUX D, KALYCHAK Y, OULADDIAF B, SKOLOZDRA R V. Magnetisation and neutron diffraction studies of HoFe12-xTaxXy (0.5≤x≤0.7, X=H, C) [J]. Journal of Alloys and Compounds, 1999, 285(1-2): 56.
[5] LI W, ZHU M G. High property rare-earth permanent magnetic materials and its pivotal preparation technique [J]. The Chinese Journal of Nonferrous Metals, 2004, 14(1): 332. (in Chinese)
[6] WANG Y, LIU G K, TONG Y X. Study on electrodeposition of Ho-Co alloy from DMSO bath [J]. Journal of the Chinese Rare Earth Society, 2001, 19(1): 54. (in Chinese)
[7] FLENGES S N, INGRAHAM T R. Elctromotive force series of metals in fused salts and activities of metal chlorides in 1:1 molar KCl-NaCl solutions [J]. J Electrochem Soc, 1959, 106: 714.
[8] YANG Q Q, LIU G K, SU Y Z. Electroreduction of holmium ion, europium ion in molten chlorides [J]. J Guangdon Non-Ferrous Metals, 1993, 2: 105. (in Chinese)
[9] BARD A J, FAULKNER L R. Electrochemical Methods, Fundamentals and Applications [M]. John Wiley and Sons Inc, 1980: 265.
[10] KABANOV B N, ASTAKHOV I I, KISELEVA I G. Formation of crystalline intermetallic compounds and solid solutions in electrochemical incorporation of metals into cathodes [J]. Electrochim Acta, 1979, 24: 167.
[11] YANG Q Q, LIU G K, CAI W W. Measurement of the diffusion coefficients of metal in its alloy phase [J]. Rare Metals, 1992, 16: 18. (in Chinese)
[12] YANG Q Q, LIU G K, SU Y Z. Electroreduction of holmium ion on iron electrode in molten chlorides [J]. Electrochmistry, 1995, 1: 44. (in Chinese)
[13] SU Y Z, YANG Q Q, LIU G K. Electroreduction of Holmium ion on nickel cathode in molten KCl-HoCl3 [J]. Journal of Rare Earth, 2000, 18: 34.
[14] SU Y Z, YANG Q Q, LIU G K. Electrorchemical formation of holmium-cobalt alloys [J]. Rare Metals, 2003, 22: 19.
[15] KABANOV B N, KISELEVA I G, ASTAKHOV I I, ASTAKHOV I I, TOMASHOVA N N, PETUKHOVA P I. Cathodic introduction of lithuim into gallium in a nonaqueous solution [J]. Elektrokhimiya, 1974, 10(5): 765. (in Russia)
(Edited by YANG Bing)