Article ID: 1003-6326(2005)03-0642-06
Influence of concentration of zinc ions on
electrocrystallization process of zinc
ZHANG Zhao(张 昭)
(Department of Chemistry, Zhejiang University, Hangzhou 310027, China)
Abstract: Cyclic voltammetry, chronoamperometry and scanning electron microscopy were employed to study the influence of Zn2+ ion concentration in electrolyte solutions on zinc electroplating process. The results show that, at high overpotentials, the nucleation of zinc is instantaneous, and nuclear density increases with the overpotentials increasing. While at low overpotentials, the zinc may be preferentially electrodeposited on surface inhomogeneities such as emergence points of edge, screw dislocations, atomic disorder, kink sites, or monoatomic steps, and no distinguished nucleation current can be observed. The major dissolution peak in cyclic voltammogram drifts positively due to the change of the rate-determining step of zinc electroplating processes from diffusion to the electrochemical reaction with the increase of Zn2+ ion concentration.
Key words: zinc electroplating; nucleation; growth; mechanism CLC
number: O646.6 Document code: A
1 INTRODUCTION
The electrodeposited coatings of zinc and its alloys have been widely used as protective films of metals, and electrodeposition of alloys is widely used in the production of new materials with specific chemical and physical properties, such as nanocrystalline magnetic thin films and functional multilayers[1-5]. As far as the electroplating process is concerned, the nucleation kinetics and the growth mechanism of the first metallic nuclei formed on the initial substrate are critical, which determine the physicochemical properties of the electrodeposited materials[6, 7] and are, therefore, crucial points to be understood and controlled.
On the other hand, the different electrocrystallization nucleation and growth processes of zinc will result in different electrodeposited layer morphologies, such as compact, spongy or dendritic surfaces[8].
The aim of this paper is to investigate the influence of Zn2+ ions concentration on the nucleation and growth mechanism of zinc, detect the relationship between the structure of deposit layer and the reaction of rate determining steps during zinc electroplating process, and provide the basis reference for further studying on the nucleation and growth mechanisms of zinc-base alloys.
2 EXPERIMENTAL
The experimental electrolyte was prepared with AR grade reagents and twice distilled water according to the basic compositions listed in Table 1. A three-electrode system consisting of cycloidal polycrystalline brass electrode with an area of 0.5027cm2 exposed which was used as working electrode(WE), saturated calomel electrode(SCE) as reference electrode and a large bright platinum foil as counter electrode, was employed. Before measurements, the exposed surface of WE were polished with silicon carbide papers from 3 through 1 to 0.5μm and velvet, rinsed with the twice distilled water, washed in acetone, rinsed with the twice distilled water again and then dried in air. The experimental temperature was (25±1)℃ controlled by thermostat water tank.
Table 1 Basic electrolyte compositions for electroplating zinc electrodeposits(g/L)

Cyclic voltammetry and chronoamperometry measurements were performed with a commercial electrochemical analyzer/workstation (Model 660A, US). After experiments, scanning electron microscopy(SEM, HITACHI S-570, Japan) was used to observe the morphologies of the electrodeposited layers.
3 RESULTS AND DISCUSSION
3.1 Voltammetric study
The influence of the concentration of Zn2+ ions in the electroplating solution on zinc deposition process was first investigated by cyclic voltammetry. The sweep potential range was set from open circuit potential to -1.5V and always initiated in the negative direction. Fig.1 shows the typical voltammograms obtained in electroplating solution with different Zn2+ ion concentrations. All voltammograms presented here are obtained during the first cycle with a scanning rate of 10mV/s. It can be seen that there are two distinct current peaks in each voltammogram. The cathodic peak is associated with Zn2+ ions reduction process(electrodeposition); while the anodic peak with the Zn dissolution process from the cupro-zinc substrate. Meanwhile, in the anodic branches of the zinc deposition, the major dissolution peaks are located at different potentials, which implies that different zinc phases are involved.
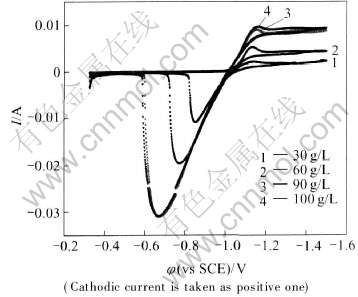
Fig.1 Cyclic voltammograms for brass electrode in basic electrolyte(pH=4.0) containing different Zn2+ ion concentrations
From Fig.1, it can be seen that the cathodic current peak intensity increases with the concentration of Zn2+ ions, indicating that the zinc deposition is controlled(limited) by the diffusion process of Zn2+ ions as far as the investigated concentration range concerned[7]. However, SEM observation of the layers obtained in different Zn2+ ion concentrations contained electrolyte solution(Jk= -1.5A/dm2) shows that, under an identical electroplating current density, the morphology of zinc electrodeposits changes from dentritic to the compact with the increase of Zn2+ ion concentration(Figs.2-4). Because the electroplating process under diffusion control favors the formation of less compact deposits, such as spongy and dentritic coatings, the rate determining step may change from diffusion process of Zn2+ ions through
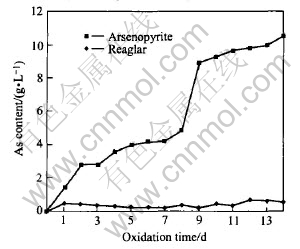
Fig.2 SEM morphology of zinc electrodeposit obtained in electrolyte containing 30g/L Zn2+ ions
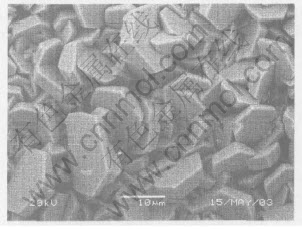
Fig.3 SEM morphology of zinc electrodeposit obtained in electrolyte containing 60g/L Zn2+ ions
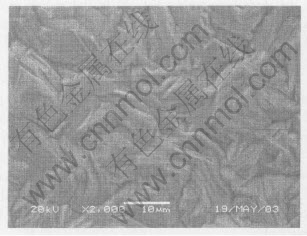
Fig.4 SEM morphology of zinc electrodeposit obtained in electrolyte containing 90g/L Zn2+ ions
the bulk electrolyte solution to the cathodic surface to electrochemical reaction with the increase of Zn2+ ions concentration.
3.2 Chronoamperometric study
In order to clarify the discrepancy between the results of cyclic voltammetry and SEM technique, zinc nucleation process is characterized in more detail by chronoamperometric analysis.
Generally, nuclei are preferentially formed on surface inhomogeneities, such as emergence points of edge, screw dislocations, atomic disorder, kink sites, or monoatomic steps. And there are a number of literatures describing the electrocrystallization process mathematically[9-16], however, up to date, the most widely employed theoretical model for electrochemical nucleation is the one developed by Hills and his colleagues[17]. In this model[12-15], the authors describe the kinetics of electrolytic phase formation at early stages when diffusion of the electroactive species from bulk solution to the interface is the rate determining step, and the growth of nuclei is considered to be 3D when taking into account of overlap of diffusion zones. According to this model, the rising portion of the current transient can be described, respectively for the instantaneous nucleation and progressive nucleation by
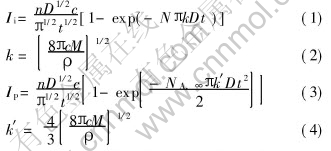
where n is the molar charge of electrodepositing species, D the diffusion coefficient, c the bulk concentration of the zinc species, N is the number of nuclei, N∞ and NA, ∞ are the total number of active sites for instantaneous nucleation and progressive nucleation respectively, M is the molar mass, ρ is the density of the deposited material, and k and k′ are the numerical constants determined by the experimental conditions.
Figs.5-7 show the relationship of the experimental non-dimensional variables between (t/tmax) and (I /Imax)2, where Imax and tmax are the maximum current transient values. Determination of the nucleation process involved is achieved by analyzing the rising section of the current transient and then comparing the curve to the dimensionless theoretical curves obtained from Eqns.(1) and (3) respectively.
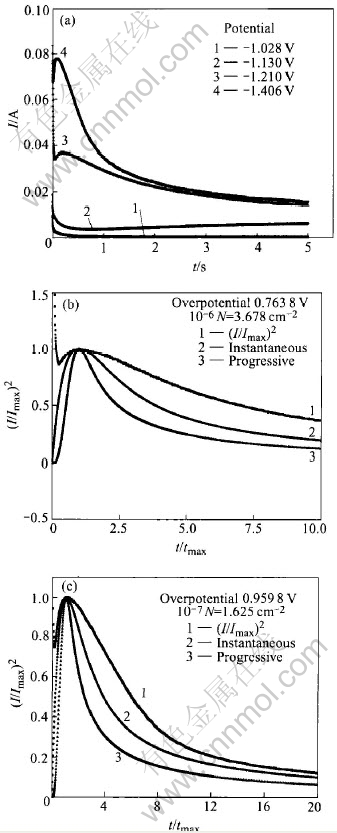
Fig.5 Potentiostatic I—t transients(a) and non-dimensional I/Imax vs t/tmax plot for electrodeposition of zinc from electrolyte containing 30g/L ZnCl2(b, c)
From Figs.5-7, it can be seen that, each of the “I—t” curves consists of an initial spike(within the first 0.02s) due to the charging of the electrochemical double layer, a subsequently rising portion due to the nucleation process and a posterior decreasing portion due to the diffusion process. The rising section appears to reach its maximum in increasingly shorter time with more negative over- potential steps. The maximum in the current tran-
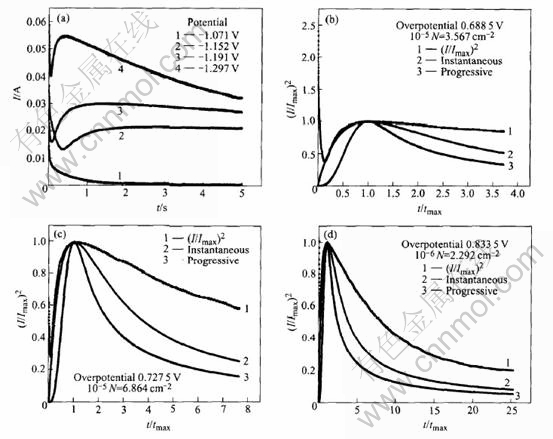
Fig.6 Potentiostatic I—t transients(a) and non-dimensional I/Imax vs t/tmax plot for electrodeposition of zinc from electrolyte containing 60g/L ZnCl2(b, c, d)
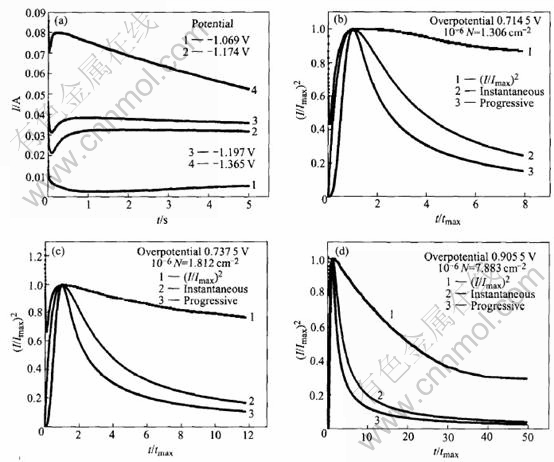
Fig.7 Potentiostatic I—t transients(a) and non-dimensional I/Imax vs t/tmax plot for electrodeposition of zinc from electrolyte containing 90g/L ZnCl2(b, c, d)
sient at high overpotential and short time corresponds to the maximum surface area, i.e. the point at which hemi-spherical nuclei are on the point of collision. Meanwhile, it can also be seen that, in the case of high overpotentials, the experimental I—t curves follow closely the theoretic instantaneous nucleation curve. However, at low overpotential, no distinguished nucleation current is observed because the practical electrode substrate surfaces usually possess many defects such as plateau edges, kinks, vacancies and emergent screw dislocations, at which crystal growth can occur without nucleation.
In order to clarify the influence of the concentration of zinc ions on the zinc electroplating process deeply and clearly, the nuclear densities(N) at different concentrations of zinc ions are calculated according to the theoretical Eqn.(5)[11-14] and illustrated in Figs.5-7.
Imax=0.6382nDc(kN)1/2(5)
With the comparison of Figs.5-7, it can be seen that the nuclear densities(N) increase with overpotential, and the overpotential for nucleation current obviously decreases with the increase of the concentration of zinc ions, which indicates that the zinc electroplating process in electrolyte solution containing less Zn2+ ions is mainly controlled by diffusion processes, which coincides with the above SEM results.
Because the rate-determining step of the zinc electroplating process is changed from diffusion process to activation reaction with the increase of zinc ions concentration, the structure of the electrodeposited zinc is changed from spongy to compact(Figs.2-4), resulting in the positive drift of the major dissolution peak in cyclic voltammogram with the increase of zinc ions concentration(Fig.1).
4 CONCLUSIONS
1) Zinc electroplating processes are controlled by diffusion of Zn2+ ion from bulk solution to cathodic surface. However, SEM and chronoamperometric results clearly show that the rate determining step of zinc electroplating process changes from diffusion to the electrochemical reaction with the increase of Zn2+ ion concentration.
2) At high overpotentials, the nucleation of zinc is instantaneous, and the nuclear densities N increase with increase of the overpotentials. While at low overpotentials, the zinc electrodeposits may be preferentially formed at surface inhomogeneities such as emergence points of edge, screw dislocations, atomic disorder, kink sites, or monoatomic steps, and no distinguished nucleation current can be observed.
REFERENCES
[1]Osaka T, Takai M, Hayashi K, et al. A soft magnetic CoNiFe film with high saturation magnetic flux density and low coercivity [J]. Nature, 1998, 392(23): 796-798.
[2]Bai A, Hu C C. Iron-cobalt and iron-cobalt-nickel nanowires deposited by means of cyclic voltammetry and pulse-reverse electroplating [J]. Electrochemical Communications, 2003, 5: 78-82.
[3]Gomez E, Labarta A, Llorente A, et al. Characterisation of cobalt/copper multilayers obtained by electrodeposition [J]. Surf Coat Technol, 2002, 153: 261-266.
[4]Li F L, Zhang B L, Dong S J, et al. A novel method of electrodepositing highly dispersed nano palladium particles on glassy carbon electrode [J]. Electrochim Acta, 1997, 42(16): 2563-2568.
[5]Zhang Z, Leng W H, Shao H B, et al. Study the behavior of Zn-Fe alloy electroplating [J]. J Electroanal Chem, 2001, 516: 127-130.
[6]Margarita M H, Manuel P P, Nikola B, et al. Identification of different silver nucleation processes on vitreous carbon surfaces from an ammonia electrolytic bath [J]. J Electroanal Chem, 1998, 443: 81-93.
[7]Margarita M H, Manuel P P, Nikola B, et al. Detailed characterization of potentiostatic current transients with 2D-2D and 2D-3D nucleation transitions [J]. Surf Sci, 1998, 399: 80-95.
[8]Zhang Z, Cai Q Y, Cao C N, et al. Primitive study on the relationship between zinc deposit morphology and electrocrystallization noise [J]. Bulletin of Electrochemistry, 2004, 20(7): 333-336.
[9]Holzle M H, Retter U, Kolb D M. The kinetics of structural changes in Cu layers on Au (111) [J]. J Electroanal Chem, 1994, 371: 101-109.
[10]Enrique B, Manuel P P, Nikola B, et al. Formation mechanisms and characterization of black and white cobalt electrodeposition onto stainless steel [J]. J Electrochem Soc, 2000, 147(5): 1787-1796.
[11]Manuel P P, Ignacio G, Nikola B. New insights into evaluation of kinetic parameters for potentiostatic metal deposition with underpotential and overpotential deposition processes [J]. J Phys Chem, 1996, 143(5): 1551-1546.
[12]Gunawardena G, Hills G, Montenegro I, et al. Electrochemical nucleation(Part Ⅲ)—the electrodeposition of mercury on vitreous carbon [J]. J Electroanal Chem, 1982, 138: 255-271.
[13]Hills G J, Schiffrin D J, Thompson J. Electrochemical nucleation from molten salts(Ⅰ)—diffusion controlled electrodeposition of silver from alkali molten nitrates [J]. Electrochim Acta, 1974, 19: 657-670.
[14]Gunawardena G, Hills G, Montenegro I, et al. Electrochemical nucleation(Part Ⅰ)—general considerations [J]. J Electroanal Chem, 1982, 138: 225-239.
[15]Scharifker B, Hills G. Theoretical and experimental studies of multiple nucleation [J]. Electrochim Acta, 1981, 28: 879-889.
[16]Scharifker B, Mostany J. Three-dimensional nucleation with diffusion controlled growth(PartⅠ)—number density of active sites and nucleation rates per site [J]. J Electroanal Chem , 1984, 177: 13-23.
[17]Schindler W, Hugelmann P, Hugelmann M, et al. Localized electrochemical nucleation and growth of low-dimensional metal structures [J]. J Electroanal Chem, 2002, 522: 49-57.
(Edited by YANG Bing)
Foundation item: Projects(20203015, 50499335) supported by the National Natural Science Foundation of China; Project supported by State Key Laboratory for Corrosion and Protection, China
Received date: 2004-09-23; Accepted date: 2005-01-05
Correspondence: ZHANG Zhao, Associate professor, PhD; Tel: +86-571-87952318; Fax: +86-571-87951895;
E-mail: eaglezzy@zjuem.zju.edu.cn