
Effect of deformed microstructure on
mechanical properties of Ti-22Al-25Nb alloy
CHENG Yun-jun(程云君), LI Shi-qiong(李世琼), LIANG Xiao-bo(梁晓波), ZHANG Jian-wei(张建伟)
Central Iron and Steel Research Institute (CISRI), Beijing 100081, China
Received 28 July 2006; accepted 15 September 2006
Abstract: Effect of the deformed microstructure on mechanical properties of an orthorhombic (Ti2AlNb) based alloy of Ti-22Al-25Nb (mole fraction, %) has been investigated. It was found that the deformed microstructures in different portions of a free forged rod with diameter of 30 mm were quite different and thus resulted in the different mechanical properties after the same subsequent heat-treatment. One deformed microstructure with less primary α2/O particles and a larger and equiaxed B2 grains resulted in poor RT ductility, but the other one with a relatively larger amount of the primary α2/O particles and non-equiaxed B2 grains had good combination of the tensile strength and ductility both at RT and 650 ℃. It was also found that two different deformed microstructures were obtained for the hot rolling plates with thickness of 3 mm even processed under an identical nominal rolling and the same post-deforming heat treatment conditions. One only has 3.5% of RT tensile elongation and the other up to 8%.
Key words: Ti2AlNb based alloy; Ti-22Al-25Nb alloy; O phase; deformed microstructure; mechanical properties
1 Introduction
Among titanium aluminides, Ti2AlNb-based alloys have received considerable attention in recent years as potential materials for high temperature applications in aerospace and aircraft engine because of their high yield strength and excellent high temperature performance [1-2]. Numerous studies have been focused on the relationship among processing, microstructure and mechanical properties of Ti2AlNb orthorhombic alloys [3-15]. It has been found that the mechanical properties of Ti2AlNb-based alloys strongly depend on the microstructures, such as the morphology and volume fractions of α2 and O phase as well as β/B2 grain sizes. CISRI has carried out a series of R & D work on Ti2AlNb based alloys and made an extensive progress both in fundamental principle research and in application research [5-7]. A Ti2AlNb based alloy with composition of Ti-22Al-25Nb (mole fraction, %) has been proved to have good mechanical properties at room and elevated temperatures especially for the duplex microstructure obtained after a solution-treatment at (α2+O+B2) phase field plus an aging treatment at (O+B2) phase field. Several testing components applied for aerospace and aircraft have also been successfully manufactured. Normally, the processing of Ti2AlNb based alloys includes two stages: firstly, the primary processing wherein the cast structure is broken down over the β/B2 transus and secondly further processing (rolling or forging) wherein the suitable shape of work-piece and proper microstructure are produced below the beta transus. For the poor workability and strong relationship between the deformation and microstructure below the β/B2 transus, much more attention should be paid to control the processing condition in this field. During the hot deformation processing, it was found that even under the same nominal processing conditions, the deformation structure obtained was different, which led to different final microstructures of components and different tensile properties after subsequent heat treatment. The relationship between the deformed microstructure and the post-deforming heat-treated microstructure as well as the tensile properties of Ti2AlNb based alloy has been rarely reported. The purpose of this study is to investigate the reasonable mechanisms leading to form different deformed microstructures, as well as the effects of which on the subsequent heat treatment microstructure and the tensile properties.
2 Experimental
Cast ingot with the nominal composition of Ti-22Al-25Nb(mole fraction, %) was prepared by using the combination of VAR and arc skull melting technologies, and with a great deal of caution to improve the homogenization of the composition and the purity of the ingot. The chemical analysis showed that the composition of the ingot agreed with the nominal composition of the alloy, and the gas impurity contents were relatively lower (e.g. oxygen: <800×10-1, nitrogen: <200×10-6 and hydrogen: <50×10-6). The ingot of the alloy was first β-forged, followed by air cooling, then free forged or rolled in (α2+B2+O) phase field to obtain rods with about 35 mm in diameter and 3 mm thick plates by multiple passes operation. The rods and the plates were heat treated with solution treatment in (α2+B2+O) phase field and then aged in (B2+O) phase field. The microstructures were investigated using optical microscopy (OM) and scanning electron microscopy (SEM). Tensile tests were performed on MTS–810 machine at room temperature and 650 ℃.
3 Results and discussion
3.1 Microstructure
Two kinds of deformed microstructures were observed on different portions of a free forged rod as shown in Fig.1. One contains less primary α2 particles and a larger amount of B2 phase with the obvious and equiaxed grain boundaries (named as Type 1 microstructure in Fig.1(a)). The other however contains a relatively larger amount of primary α2 particles and elongated B2 grain with irregular grain boundary structure (named as Type 2 microstructure in Fig.1(b)). The post-deforming heat treated microstructures corresponding to the two different portions of the rod are shown in Fig.2(a) and Fig.2(b) respectively. The B2 equiaxed grain boundaries still can be seen clearly for Type 1 deformed microstructure after the subsequent heat treatment as shown in Fig.2(a).
For the hot rolled plates, even processed under the same nominal rolling condition, at least two kinds of deformed microstructures were obtained. One contains about 10% primary α2/O particles elongated and distributed in transformed B2 phase matrix (named as Type 3 in Fig.3(a)). The other contains elongated O and B2 phases, with precipitation of fine and lenticular O phase within the transformed B2 phase (named as Type 4, in Fig.3(b)).
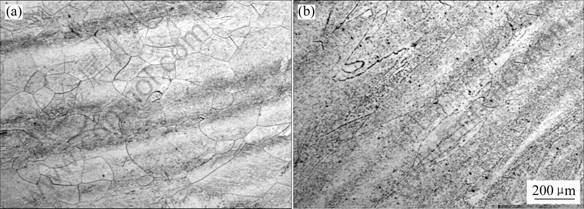
Fig.1 Optical micrographs of deformed microstructures in forged rod: (a) Type 1; (b) Type 2
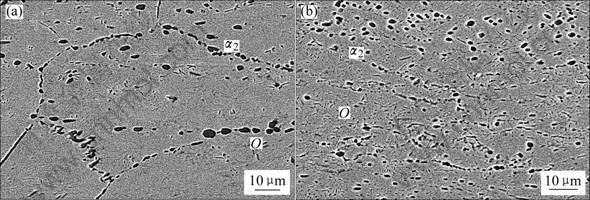
Fig. 2 SEM images of heat-treated microstructure resulted from deformed microstructure of Type 1(a) and Type 2(b ) in Fig.1
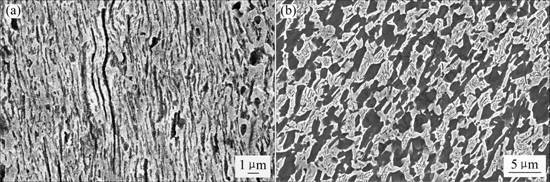
Fig.3 SEM images of deformed microstructure of rolling plates: (a) Type 3; (b) Type 4
After the same subsequent heat treatment, both kinds of the deformed microstructures resulted in similar duplex microstructure but with different details. One consists of fine primary α2/O particles(in dark contrast) and extremely fine and crisscross lath-like O phase(in grey contrast) distributed homogeneously in transformed B2 matrix(in light contrast) as shown in Fig.4(a). The other, however, consists of coarse and lath-like B2 phase precipitated in some of the O phase regions shown in Fig.4(b).
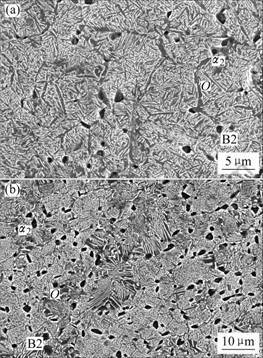
Fig.4 SEM images of heat-treated microstructure resulted from deformed microstructure of (a) Type 3 and (b) Type 4 in Fig.3
3.2 Tensile properties
Table 1 lists the tensile properties of the four types of post-deforming heat treated microstructures which were resulted from the four different deformed microstructures respectively. Compared with Type 2, the post-forging heat-treated microstructure resulted from Type 1 deformed microstructure of the rod has poor RT tensile elongation (δ5=3.5%). Compared with Type 3, the RT elongation of the post-rolling heat-treated microstructures resulted from Type 4 deformed microstructure was also lower.
Table 1 Tensile properties of free forged rods and rolling plates
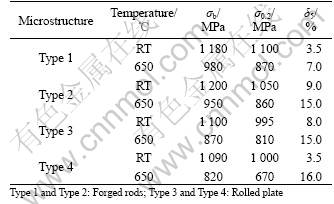
3.3 Discussion
Type 1 deformed microstructure resulted in poor RT ductility of the forged rod is due to the presence of the equiaxed and obvious B2 grain boundaries, which consists of thin and almost continuous α2 phase lathes. Such grain boundary structure was caused by local overheat in some portions of the rod during the free forging processing as a result of relatively high strain rates or large deformation reduction. The temperature of the overheat portions may be close to the B2 transus. In general, in processing of titanium alloy, overheat easily occurs locally or occasionally would lead to the presence of inhomogeneous microstructure in a work-piece.
Type 4 deformed microstructure of the rolled plates shown in Fig.3(b) is similar to the microstructure of rolling sheet with Ti-23Al-27Nb alloy reported by BOEHLERT [9]. Such deformed microstructure after post-deforming heat-treatment could result in the final microstructure with a relatively large amount of primary O phase as well as the coarse and lath-like B2 precipitated within O phase, which would lead to a decrease in ductility of the present alloy. The responsible causes for forming Type 4 deformed microstructure (Fig.3(b)) of the rolled plate can be in terms of an incorrect control of the rolling temperature when the rolling processing was sometimes performed in (O+B2) phase field.
4 Conclusions
1) The two typical deformed microstructures were obtained in different portions of a free forged rod. One deformed microstructure contains less primary α2/O particles and a larger amount of B2 phase with obvious and equiaxed grain boundaries caused by the effect of the localized overheat on some portions of the rod during forging. The other contains a relatively larger amount of primary α2/O particles and non-equiaxed B2 grains.
2) After the same subsequent heat treatment of the rod, the first deformed microstructure results in poor RT ductility, but the second one results in good combination of tensile strength and ductility at both RT and 650 ℃.
3) Different rolled plates with thickness of 3 mm processed with the identical nominal rolling condition have two different deformed microstructures. One contains about 10% of α2/O particles distributed in elongated transformed B2 phase matrix; the other contains elongated O and B2 phases with precipitation of fine and lenticular O phase within B2 phase.
4) After the same post-deforming heat treatment, the two rolled microstructures chang to similar duplex microstructure, but the details present a little different, which strongly leads to a decrease in RT ductility.
References
[1] Germann L, Banerjee D, Guédou J Y, Strudel J L. Effect of composition on the mechanical properties of newly developed Ti2AlNb-based titanium aluminide [J]. Intermetallics, 2005, 13: 920-924.
[2] Banejee D,Gogia A K, Nandi T K, JoshI V A. A new ordered orthorhombic phase in Ti3Al-Nb alloy [J]. Acta Metall, 1988, 36: 871-882.
[3] Row R G. The mechanical properties of titanium aluminide near Ti-25Al-25Nb [A]. KIM Y W, BOYE R R. Microstructure/Property Relationships in Titanium Aluminides and Titanium Alloys[C]. TMS AIME, Warrendale, 1991: 387-398.
[4] Gogia A K, Nandy T K, Banerjee D, et al. Microstructure and mechanical properties of orthorhombic alloy in the Ti-Al-Nb system [J]. Intermetallics, 1998, 6: 741-748.
[5] MAO Yong, LI Shi-qiong, ZHANG Jian-wei, et al. Microstructre and tensile properties of orthorhombic Ti-Al-Nb-Ta alloys[J]. Intermetallics, 2000, 8: 659-662.
[6] LI Shi-qiong, MAO Yong, ZHANG Jian-wei, et al. Effect of microstrucutre on tensile properties and fracture behavior of intermetallic Ti2AlNb alloys [J]. Trans Nonferrous Met Soc China, 2002, 12(4): 582-586.
[7] LI Shi-qiong, CHENG Yun-jun, LIANG Xiao-bo, et al. Recent work on alloy and process development of Ti2AlNb based alloys [J]. Material Science Forum, 2005, 475-479: 795.
[8] Germann L, Banerjee D, Guédou J Y, Strudel J L. Microstructure-property relationships in newly developed multiphase Ti2AlNb-based titanium aluminides [A]. The 10th world Conference on Titanium [C] . Hanburg Germany, 2003, 4: 2137-2144.
[9] Boehlert C J. The effects of forging and rolling on microstructure in O+BCC Ti-Al-Nb alloys [J]. Materials Science and Engineering, 2000, A279: 118-129.
[10] Boehlert C J, Majumdar B S, Seetharaman V, Miracle D B. The microstructural evolution in Ti-Al-Nb O+BCC orthorhombic alloys [J]. Metallurgical and Materials Transactions A, 1999, 30A: 2305-2323.
[11] Boehlert C J. The tensile behavior of Ti-Al-Nb O+bcc orthorhombic alloys [J]. Metall Trans, 2001, 32A: 1977-1988.
[12] Nandy T K, Banerjee D. Creep of orthorhombic phase based on the intermetallic Ti2AlNb [J]. Intermetallics, 2000, 8: 915-928.
[13] ZHANG Jian-wei, LI Shi-qiong, ZOU Dun-xun, et al. Processing and microstructure control of (α2+B2+O) alloy sheet in Ti-Al-Nb system [J]. Intermetallics, 2000, 8: 699-702.
[14] SADI F A, SERVANT C. On the B2→O phase transformation in Ti-Al-Nb alloys [J]. Materials Science and Engineering, 2003, A346: 19-18.
[15] SAROSI P M, PARTRIDGE A, SHELTON E F J, JONES I P. Thermal processing of an orthorhombic (Ti2AlNb) based alloy [A]. Hemker K J, Dimiduk D M, Clemens H, et al. Structural Intermetallics 2001[C]. TMS, 2001, C05.
(Edited by PENG Chao-qun)
Corresponding author: CHENG Yun-jun; Tel: +86-10-62181009; E-mail: Tial@superalloy.cn