
Effects of Pd substitution for Ni on corrosion performances of Mg0.9Ti0.1Ni1-xPdx hydrogen storage alloys
TIAN Qi-feng(田琦峰)1,2, ZHANG Yao(张 耀)1,2, TAN Zhi-cheng(谭志诚)1,2, XU Fen(徐 芬) 1,2,
SUN Li-xian(孙立贤)1,2, ZHANG Tao(张 涛)1,2,Yuan Hua-tang(袁华堂)3
1. Dalian Institute of Chemical Physics, Chinese Academy of Sciences, Dalian 116023, China;
2. Graduate School of Chinese Academy of Sciences, Beijing 100049, China;
3. Institute of New Energy Material Chemistry, Nankai University, Tianjin 300071, China
Received 29 August 2005; accepted 10 January 2006
Abstract: The Mg0.9Ti0.1Ni1-xPdx (x= 0, 0.05, 0.1, 0.15) hydrogen storage electrode alloys were prepared by mechanical alloying. The main phases of the alloys were determined as amorphous by X-ray diffraction(XRD). The corrosion potentials of the alloys were measured by open circuit potential measurements and the values are -0.478, -0.473, -0.473 and -0.471 V (vs Hg/HgO electrode) for x=0, 0.05, 0.1, 0.15, respectively. The corrosion currents of the studied alloys were obtained by non-linear fitting of the anodic polarization curve using Bulter-Volmer equation and Levenberg-Marquardt algorithm, which were obtained after different cycles. The initial corrosion currents of the alloys are decreased with the increasing of Pd content. The increasing of Pd content in the alloys inhibits the corrosion rates of the electrode alloys with the progress of cycle number. The electrochemical impedance spectroscopy(EIS) was conducted after open circuit potential of the alloys stabilizing. The impedance data fit well with the theoretical values obtained by the proposed equivalent circuit model. The corrosion resistances and the thickness of surface passive film of the alloys, which were deduced by the analyses of EIS, are enhanced with the increasing of Pd content in the alloys, which are consistent with the results of corrosion rates obtained from anodic polarization measurements.
Key words: MgNi-based alloys; Pd; mechanical alloying; hydrogen storage alloy; substitution; corrosion resistance
1 Introduction
MgNi-based amorphous hydrogen storage alloys have drawn much attention in the last ten years. Compared with AB5 or AB2 type electrode alloys, the alloy candidates for the negative electrode of Ni-MH batteries possess several advantages, such as higher discharge capacity, lighter mass and lower cost[1, 2].
However the major hindrance of its practical application is the rapid degradation of discharge capacity due to the drastic oxidation of magnesium in KOH solution. Therefore, the settlement of this corrosion problem is very important for the application of MgNi-based electrode alloys[3].
Up to now, many studies of MgNi-based amorphous hydrogen storage alloys focused on improving its cycle life by means of element substitution[4, 5]. Recently it was found that partial substitution of Ti for Mg improved the alloy’s cycle life significantly[6-8]. The latest study found that partial substitution for Ti by Zr, Cr, and V in the Mg35Ti10Ni55 electrode alloy noticeably enhanced its anti-corrosion properties and cyclic capacity retention rate[9]. It was revealed that the combined passive films formed on the surface of the alloys effectively inhibited the corrosion induced by KOH. However, the passive films blocked the charge transfer through the surface of the alloy. The discharge capacity of the substituted alloys is usually lower than that of the initial alloys.
Pd plays a protective role for the electrode alloys from corrosion in alkaline solution. MA et al[10,11] found that the corrosion inhibition properties were greatly improved by modifying the surface and the bulk of MgNi-based amorphous alloy with Pd. Their results showed that the Pd addition inhibited the Mg(OH)2 formation on the surface of the alloys. PARK et al [12] performed in-situ Pd deposition on nanocrystalline Mg2Ni electrodes during the charge cycles and found it improved the electrode properties. The growth of Mg(OH)2 layer was retarded effectively, which enhanced the cyclic stability and rate capability of the electrode alloy in the Pd-added electrolyte. Yamaura et al[13, 14] demonstrated that the Pd addition also improved the cyclic stability of Mg2Ni-based alloys prepared by melt spinning.
In our previous study[15], the substitution of Pd for Ni in Mg0.9Ti0.1Ni alloy significantly enhanced the cyclic stability of the alloys during charge-discharge cycles. In the present work, the corrosion behaviors of Mg0.9Ti0.1Ni1-xPdx (x=0, 0.05, 0.1, 0.15) alloys in stationary and cyclic charge-discharge conditions were intensively studied. Several electrochemical techniques such as open circuit potential test, anodic polarization and electrochemical impedance spectroscopy(EIS) were adopted to characterize the corrosion performance of Mg0.9Ti0.1Ni1-xPdx (x=0, 0.05, 0.1, 0.15) hydrogen storage electrode alloys in KOH solution.
2 Experimental
Mg0.9Ti0.1Ni1-xPdx (x=0, 0.05, 0.1, 0.15) alloys were prepared by mechanically alloying(MA). The purity of all metallic powders was higher than 99.5%. The powder mixtures as designed stoichiometry were ground in a planetary ball mill for 120 h under Ar atmosphere, with a ball to powder mass ratio of 30?1. The structures of the alloys were characterized by X-ray diffraction (XRD, Rigaku D/max-2500, Cu Kα, 50 kV, 200 mA).
The tested working electrode was the pressed mixture of 0.1 g alloy powder with 0.3 g electrolytic Cu powder. The NiOOH/Ni(OH)2 electrode and Hg/HgO electrode were used as counter and reference electrodes, respectively. The electrolyte was 6 mol/L KOH aqueous solution. The charge-discharge measurements were performed upon an automatic LAND battery test instrument. The electrodes were charged for 3 h at a current density of 300 mA/g, rested for 5 min and then discharged to -0.6 V (vs Hg/HgO electrode) at a current density of 100 mA/g.
The open circuit potentials of Mg0.9Ti0.1Ni1-xPdx (x=0, 0.05, 0.1, 0.15) electrode alloys were recorded immediately after inserting the electrode into KOH solution for 10 h and the open circuit potentials of electrodes became stable. The anodic polarization curves were measured from 0 to 100 mV (vs open circuit potential) at the end of 1st, 5th, 10th and 20th cycles. The electrochemical impedance spectroscopy measure- ments were conducted on the Zahner Elektrik IM6e electrochemical workstation from 5 mHz to 10 kHz with an amplitude of 5 mV (vs open circuit potential) after open circuit potential measurements.
3 Results and discussion
3.1 Phase structure of alloys
Fig.1 shows the X-ray diffraction patterns of the Mg0.9Ti0.1Ni1-xPdx (x=0, 0.05, 0.1, 0.15) quaternary alloys after 120 h ball milling. For each alloy, a broad Bragg peak is observed at approximately 2θ=42?. No secondary phase or residual starting materials are observed, which suggests that a single amorphous phase forms in these alloys after 120 h ball milling.
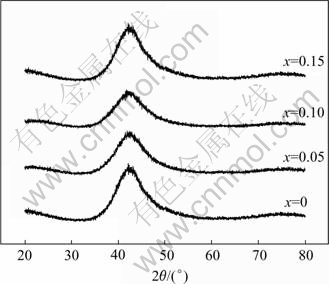
Fig. 1 XRD patterns of Mg0.9Ti0.1Ni1-xPdx (x=0, 0.05, 0.1, 0.15) alloy powders after mechanical alloying for 120 h
3.2 Corrosion potentials of alloys
The corrosion potentials of Mg0.9Ti0.1Ni1-xPdx (x=0, 0.05, 0.1, 0.15) electrode alloys were determined by open circuit potential measurements. Fig.2 demonstrates the variation of open circuit potentials of Mg0.9Ti0.1Ni1-xPdx (x=0, 0.05, 0.1, 0.15) alloys with time. The measure- ments started immediately after immersing the alloys in KOH solution. From this figure, one can see that the stable corrosion potential of Mg0.9Ti0.1Ni1-xPdx alloys is -0.478, -0.473, -0.473 and -0.471 V (vs Hg/HgO electrode) for x=0, 0.05, 0.1, 0.15, respectively. It is obvious that the steady-state potential shifts towards more negative direction with the decrease of Pd content. This phenomenon indicates that the increase of Pd content in the alloys is favorable to improving the anti- corrosion performance of the alloys.
3.3 Corrosion rates of alloys
The effect of Pd content on the corrosion rates of Mg0.9Ti0.1Ni1-xPdx (x=0, 0.05, 0.1, 0.15) alloys was investigated by anodic polarization measured after the 1st, 5th, 10th and 20th charge-discharge cycles. The obtained curves were non-linearly fitted with Bulter-Volmer Equation using Levenberg-Marquardt algorithm. The corrosion current Icorr and the Tafel constant ba, bc are the fitted parameters in the Bulter-Volmer Equation[16]:
(1)
where I and ?E are experimentally obtained current and over-potential, respectively.
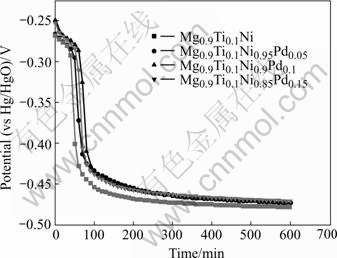
Fig.2 Open circuit potentials of Mg0.9Ti0.1Ni1-xPdx (x=0, 0.05, 0.1, 0.15) electrode alloys after immediately immersing in KOH solution
Fig.3 exhibits the fitted corrosion currents of Mg0.9Ti0.1Ni1-xPdx (x=0, 0.05, 0.1, 0.15) electrode alloys during cycles. The order of initial corrosion current for Mg0.9Ti0.1Ni1-xPdx (x=0, 0.05, 0.1, 0.15) alloys is as follows: Mg0.9Ti0.1Ni>Mg0.9Ti0.1Ni0.95Pd0.05>Mg0.9Ti0.1- Ni0.9Pd0.1≈Mg0.9Ti0.1Ni0.85Pd0.15.
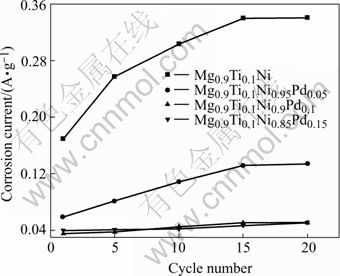
Fig.3 Corrosion currents as function of cycle number for Mg0.9Ti0.1Ni1-xPdx (x=0, 0.05, 0.1, 0.15) electrode alloys at 303 K
One can see that the corrosion rates during charge-discharge cycles vary greatly with the alloys containing different Pd contents. For the alloys with higher Pd content, such as Mg0.9Ti0.1Ni0.9Pd0.1 and Mg0.9Ti0.1Ni0.85Pd0.15, the corrosion current increases very slowly with the cycles. On the contrary, the corrosion current increases progressively for the alloys with lower Pd content or without Pd. It is demonstrated that the corrosion rate decreases with the augmentation of Pd content in the Mg0.9Ti0.1Ni1-xPdx (x=0, 0.05, 0.1, 0.15) alloys. With the cycle proceeding, the discharge capacity degrades to a stable value whereas the corrosion current increases to a steady one. Therefore, the variation of corrosion rates of the alloys coincides with the evolution of the discharge capacities of the alloys reported by the authors[15].
3.4 Electrochemical impedance measurements of alloys
The impedance data obtained after soaking the Mg0.9Ti0.1Ni1-xPdx (x=0, 0.05, 0.1, 0.15) alloys for 10 h in 6 mol/L KOH solution are plotted in Fig.4. The Bode plots of the studied alloys show two phase angle maxima, which indicates that the presence of two time constants denotes the electrode process. The impedance data were analyzed with the proposed equivalent circuit as shown in Fig.5. In the figure, the capacitive components labeled by C are modeled as constant-phase elements(CPE) to describe the depressed nature of the semicircles. Rel denotes the electrolyte resistance between the MH electrode and the reference electrode. Rct and Cct representing the semicircle in the low-frequency region contribute to the charge-transfer reaction resistance and the double-layer capacitance, respectively. The passive film resistance and capacitance on the surface of the alloy particles generate the parameters Rpf and Cpf, respectively. It can be seen that the proposed equivalent circuit fits well with the present system since the measured data and fitted curve are very close to each other in Figs.4(a) to 4(d).
Fig.6 shows the relationship between the fitted passive film resistance Rpf and the reciprocal of Cpf as a function of Pd content in the alloys. The passive film resistance Rpf increases with the Pd content increasing, which agrees well with the results of open circuit potentials and corrosion current measurements. The passive film thickness, which is reversibly proportional to Cpf, also increases with the Pd content increasing[17]. It is concluded that the film thickness and resistance become stable when the Pd content increases to 0.1. It should be noted that during cycles the corrosion rate is also lowered to a stable value when the Pd content reaches 0.1. Therefore, the Pd content is a very important factor that determines the corrosion behavior of the alloys in KOH solution. The further corrosion of the alloy particles is inhibited effectively when the Pd content reaches 0.1.
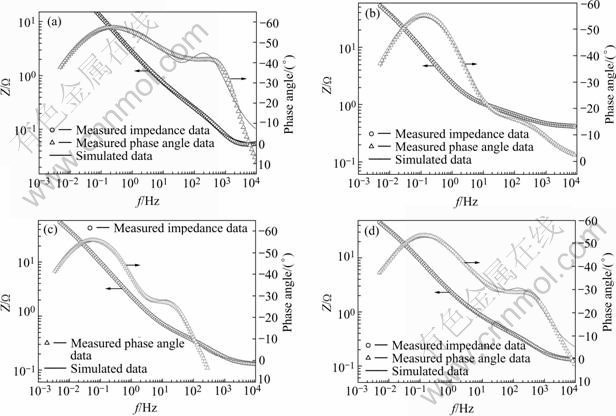
Fig.4 Bode plots of Mg0.9Ti0.1Ni1-xPdx (x=0, 0.05, 0.1, 0.15) electrode alloys after immersion in 6 mol/L KOH solution for 10 h: (a) Mg0.9Ti0.1Ni; (b) Mg0.9Ti0.1Ni0.95Pd0.05; (c) Mg0.9Ti0.1Ni0.9Pd0.1; (d) Mg0.9Ti0.1Ni0.85Pd0.15.
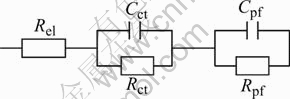
Fig.5 Equivalent circuit used in fitting of impedance data
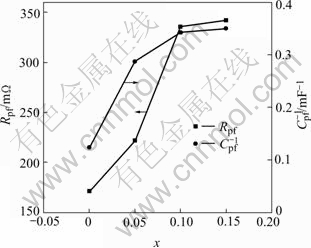
Fig.6 Relationship between fitted Rpf and 1/Cpf as function of Pd content in Mg0.9Ti0.1Ni1-xPdx (x=0, 0.05, 0.1, 0.15) alloys
4 Conclusions
The increase of Pd content leads to more positive corrosion potentials. Pd plays an important role in the anti-corrosion behavior of Mg0.9Ti0.1Ni1-xPdx (x=0, 0.05, 0.1, 0.15) alloys. The corrosion of the alloys with Pd content reaching 0.1 or more is retarded effectively. The proposed equivalent circuit is suitable for the studied system. The increase of Pd content also enhances the corrosion resistances and decreases the corrosion rates of the alloys during charge-discharge cycles.
References
[1] LEI Yong-quan, WU Yu-ming, YANG Quan-ming, WU Jing, WANG Qi-dong. Electrochemical behaviour of some mechanically alloyed Mg-Ni-based amorphous hydrogen storage alloys [J]. Z Phys Chem Bd, 1994, 183: 379-384.
[2] XU Yi-jun, TANG You-gen, HUANG Bo-yun. Progress in research on Mg-Ni based hydrogen storage alloys [J]. Mater Rev, 2003, 17(12): 9-11.
[3] ZHANG Yao, LI Shou-quan, CHEN Li-xin, LEI Yong-quan, WANG Qi-dong. Corrosion mechanism of mechanically alloyed Mg50Ni50 and Mg45Cu5Ni50 alloys [J]. Trans Nonferrous Met Soc China, 2002, 12(2): 238-241.
[4] NOHARA S, HAMASAKI K, ZHANG S G, INOUE H, IWAKURA C. Electrochemical characteristics of an amorphous Mg0.9V0.1Ni alloy prepared by mechanical alloying [J]. J Alloys Compd, 1998, 280(1-2): 104-106.
[5] LIU Wei-hong, WU Hao-qing, LEI Yong-quan, WANG Qi-dong, WU Jing. Effects of substitution of other elements for nickel in mechanically alloyed Mg50Ni50 amorphous alloys used for nickel metal hydride batteries [J]. J Alloys Compd, 1997, 261(1-2): 289- 294.
[6] YE H, LEI Y Q, CHEN L S, ZHANG H. Electrochemical character- ristics of amorphous Mg0.9M0.1Ni (M=Ni, Ti, Zr, Co and Si) ternary alloys prepared by mechanical alloying [J]. J Alloys Compd, 2000, 311(2): 194-199.
[7] HAN S C, LEE P S, LEE J Y, ZUTTEL A, SCHLAPBACH L. Effects of Ti on the cycle life of amorphous MgNi-based alloy prepared by ball milling [J]. J Alloys Compd, 2000, 306(1-2): 219-226.
[8] ZHANG Yao, ZHANG Shu-kai, CHEN Li-xin, LEI Yong-quan, WANG Qi-dong. The study on the electrochemical performance of mechanically alloyed Mg-Ti-Ni-based ternary and quaternary hydrogen storage electrode alloys [J]. Int J Hydrogen Energy, 2001, 26(8): 801-806.
[9] ZHANG Yao, CHEN Li-xin, LEI Yong-quan, WANG Qi-dong. The effect of partial substitution of Ti with Zr, Cr, or V in the Mg35Ti10Ni55 electrode alloy on its electrochemical performance [J]. Electrochim Acta, 2002, 47(11): 1739-1746.
[10] MA T J, HATANO Y, ABE T, WATANAB E K. Effects of Pd addition on electrochemical properties of MgNi [J]. J Alloys Compd, 2004, 372(1-2): 251-258.
[11] MA T J, HATANO Y, ABE T, WATANAB E K. Effects of bulk modification by Pd on electrochemical properties of MgNi [J]. J Alloys Compd, 2005, 391(1-2): 313-317.
[12] PARK H J, GOO N H, LEE K S. In situ Pd deposition on Mg2Ni electrodes for Ni/MH secondary batteries during charge cycles [J]. J Electrochem Soc, 2003, 150(10): A1328-A1332.
[13] YAMAURA S I, KIM H Y, KIMURA H, INOUE A, ARATA Y. Thermal stabilities and discharge capacities of melt-spun Mg-Ni-based amorphous alloys [J]. J Alloys Compd, 2002, 339(1-2): 230-235.
[14] YAMAURA S I, KIM H Y, INOUE A. Discharge capacities of melt-spun Mg-Ni-Pd amorphous alloys [J]. J Alloys Compd, 2003, 358(1-2): 173-176.
[15] TIAN Qi-feng, ZHANG Yao, CHU Hai-liang, SUN Li-xian, XU Fen, TAN Zhi-cheng, YUAN Hua-tang, ZHANG Tao. The electro- chemical performances of Mg0.9Ti0.1Ti1-xPdx (x=0-0.15) hydrogen storage electrode alloys [J]. J Power Sources, in press.
[16] CAO Chu-nan. The Principles of Corrosion Electrochemistry [M]. Beijing: Chemical Industry Press, 2004. 120-122. (in Chinese)
[17] BADAWY W A, ISMAIL K M, FATHI A M. Effect of Ni content on the corrosion behavior of Cu-Ni alloys in neutral chloride solutions [J]. Electrochim Acta, 2005, 50(18): 3603-3608.
Foundation item: Project(20473091) supported by the National Natural Science Foundation of China
Corresponding author: TAN Zhi-cheng; Tel: +86-411-84379219; E-mail: tzc@dicp.ac.cn
(Edited by YUAN Sai-qian)