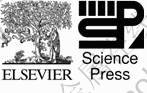
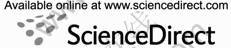
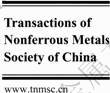
Trans. Nonferrous Met. Soc. China 22(2012) 360-365
Preparation and characterization of composite microspheres of nano zinc ferrite/poly (D, L-lactide-co-alanine)
CAO Lei-jian1, ZHOU Qing-hua2, GU Li3, SHEN Hong-xia3, LI Qing-hua1, LAN Ping3, FANG Yan3
1. School of Materials Science and Engineering, Changzhou University, Changzhou 213164, China;
2. College of Medicine, Jiaxing University, Jiaxing 314001, China;
3. Institute of Polymer Material Engineering, Jiaxing University, Jiaxing 314001, China
Received 30 March 2011; accepted 18 June 2011
Abstract: Magnetic nano zinc ferrite fliuds were synthesized using an improved liquid phase chemical method, which would be used to replace tradditional iron oxides magnetic material. A novel copolymer (PLAA) with D, L-lactide (D, L-LA) and alanine was synthesized using stannous octoate as initiator. Magnetic polymer microspheres were fabricated with nano zinc ferrite fluid coated with alanine modified poly lactide. These as-prepared zinc ferrite fluids, modified poly lactide and magnetic composites, were characterized with X-ray diffraction diffractometer, FT-IR spectrometer, nuclear magnetic resonance spectrometer, scanning electron microscope, transmission electron microscope, vibrating sample magnetometer, and thermogravimetric analyzer. The results demonstrate that the as-prepared zinc ferrite is spinel type of ZnFe2O4 nano crystals with particle size of 20-45 nm and magnetization of 32×10-3 A·m2. Alanine is copolymerized with lactide, and the prepared composite magnetic microsphere is coated with the modified polylactide, with mass fraction of 45.5% of PLA, particle size ranging from 80-300 nm, and magnetization of 10.6×10-3 A·m2, which suggests ZnFe2O4 enjoys a stable magnetization after being coated by polymer.
Key words: zinc ferrite; alanine; polylactide; magnetic composite microsphere
1 Introduction
Magnetic polymer beads are of great interest as targeted drug delivery carrier [1-3], which was endowed with excellent biocompatibility, degradation controllability and physical allocation, and have been of great importance in medical applications, such as targeted drug delivery [4], contrast agents in magnetic resonance imaging [5], bioseparation [6], and destruction of the tumor tissue under the action of high-frequency magnetic fields [7,8]. Polymer materials, used for drug slow released carrier, include natural polymers (chitosan) and synthesized polymer (poly lactide (PLA), polyethylene glycol, polyvidone, and so on) [9,10], poly lactide and copolymer were used as biodegradable material for preparation of drug carrier system. Poly lactide was endowed with excellent biocompatibility and degraded into water and carbon dioxide, and the intermediate product was lactic acid which was not easy to be agglomerated in organs, so PLA has been widely used as targeted drug controlling carrier recently [11]. While long-term research indicated that poly lactide was easy to cause inflammation reaction as a result of its acidity [12].
Ferroferric oxide performing as magnetic core has been in broad application in drug controlling carrier recently, which was endowed with strong magnetic response[13,14]; however, ferroferric oxide was easy to accumulate into large scale and did not reach tumor and finally deposited in endothelial system of liver and kidney, resulting in embolism or intoxication. So, the key factor in targeted drug carrier study was to obtain magnetic material with even size, stale dispersion and strong magnetic response [15,16].
In this work, the synthesized ZnFe2O4 nano crystals were chosen for magnetic core. Alanine modified poly lactide was chosen for encapsulation material. Magnetic PLA composite particles were obtained by reverse emulsion method, for more in-depth study on targeted drug controlling carrier.
2 Experimental
2.1 Materials
Main reagents were urea, olei acid, iron trichloride, cobalt chloride, zinc oxide, methanol, acetone, absolute ethanol, alanine, chloroform, tetrahydrofuran (all were analytically pure, Shanghai National Medicine), stannous octoate (95%, Sigma), and D, L lactide (synthesized in laboratory).
2.2 Preparation of zinc ferrite nano fluids
Improved liquid-phase chemical precipitation method was applied to synthesizing zinc ferrite magnetic fluids [17, 18]. 400 mL de-ionized water and certain amount of oleic acid were added into a clean beaker; the mixture was kept under ultrasonic dispersion for 50 min and sol appeared; excess amount of urea was added into the sol and dissolved completely; the reaction system presented milk white; ferric oxide (10.0 mmol) and zinc chloride (5.0 mml) were added into the pre-formed sol system and dissolved; pale red appeared in the solution. The solution was transformed into a round bottom flask and was kept in microwave synthesizer at temperature ranging from 95 to 100 °C for 3-4 h, which was kept being stirred (1000 r/min) all the time. Then the reaction system was cooled naturally to room temperature and presented dark red sol, into which 20 mL absolute ethanol, 10 mL acetone and 10 mL methanol were added for de-emulsification; after being kept stewing for 1 h, the sol was centrifugated (9000 r/min), and marron red deposit presented at the bottom of centrifugetube and was washed with acetone 5 times; dark red crystals were obtained after the marron red deposit was desiccated by vacuum.
2.3 Copolymerization of alanine and D, L lactide
Dried and pure D, L lactide (3 g) and alanine (90 mg) were put into an agate mortar and ground completely to form even mixture. The mixture was transferred into an ampule, into which then chloroform solution with stannous octoate was added as initiator(mlactide/mstannous octoate was 0.7%). Chloroform was removed by decompressing, the ampule was sealed under vacuum and was kept in vacuum drying oven at 180 °C for 18 h. After being purified with tetrahydrofuran (C4H8O) and deposited with water, the light yellow alanine modified poly lactide (PLAA) membrane was accomplished.
2.4 Preparation of magnetic polymer microspheres by coating zinc ferrite nanocrystals with PLAA
100.0 mg the alanine modified PLA and 20 mg magnetic zinc ferrite nanoparticles were dispersed in 10 mL acetone, and was then added dropwise into 50 mL deionized water under vigorous stirring. The resulting solution was sonicated for 5 min in an ultrasonic bath to get a stable suspension. The acetone was then removed by rotary evaporation. The formed PLAA–MNPs (magnetic nano particles) was separated by centrifugation at 20000 r/min for 10 min and resuspended with 10 mL DI water. After dialysis for 48 h, the PLAA–MNPs solution was freeze-dried.
2.5 Analysis and characterization
Zinc ferrite was characterized for composition and crystal structure using X-ray diffractometry (Cu Kα, λ=1.541 78 ?, Rigaku D/MAX-rotation). Crystallites and magnetic polymer microsphere were observed by scanning electron microscopy (SEM, Hitachi S-4700) and transmission electron microscope (TEM, TecnaiG220). NMR experiments were conducted in a Varian Inova 400 with tetralmethylsilane as internal standard. Samples were solved in deuterochloroform. Infrared spectrum of the as-prepared PLAA was recorded with a Nicolet-IR550 infrared spectrometer. TGA was performed to record the content of PLAA out of magnetic polymer microsphere with a Netzsch STA-409 PC apparatus under nitrogen gas at a flow rate of 25 cm3/min. The magnetic properties of the zinc ferrite nanaoparticles and the encapsulated nanoparticles were measured by means of vibrating sample magnetometry using LDJ model 9600. The samples were placed in a capillary tube, in an inert atmosphere of nitrogen and sealed. The maximum magnetic field applied was 796 A/m.
3 Results and discussion
3.1 Structure, morphology, size and magnetic property determination of ZnFe2O4 nanocrystals
The phase and component of the as-prepared product were characterized by powder X-ray diffraction (XRD), and a typical XRD pattern is shown in Fig. 1. The reflection peaks can be readily indexed to planes (111) (220), (311), (400), (331), (442), (511), and (440) of spinel ZnFe2O4 with a cubic symmetry (JCPDS file, No. 22-1012), respectively. The broadened diffraction peaks can be attributed to the reduced particle size of the product. The crystal size ranged from 20 mm to 35 nm by Scherre equation.
The magnetic properties of the as-prepared ZnFe2O4 nanocrystals were first evaluated by a vibrating sample magnetometer. As the field was cycled between -79.6×104 and 79.6×104 A/m, the magnetization curve (Fig. 2) measured at room temperature (296 K) demonstrates a typical superparamagnetic behaviour of the as-prepared ZnFe2O4 nanocrystals with a little coercivity (63.7 A/m) and retentivity (2×10-6 A·m2), and with a saturation magnetization of 32×10-3 A·m2, suggesting the synthesized ZnFe2O4 nanocrystals were endowed with excellent magnetic property.
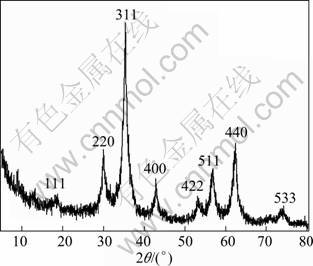
Fig. 1 XRD pattern of as-prepared ZnFe2O4 (All reflection peaks can be exactly indexed, demonstrating that they are spinel ferrites.)
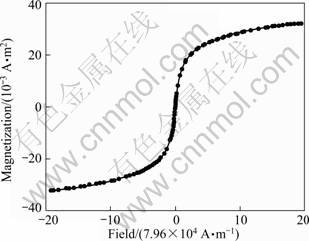
Fig. 2 Magnetization curve of ZnFe2O4 nanocrystals measured at room temperature (296 K)
To evaluate the effect of oleic on the morphology of zinc ferrite nanocrystals, five groups of parallel experiment (with amount of oleic as the only variation) were performed, and the oleic acid was added by volume of 0.1, 0.3, 0.5, 0.7 and 1.0 mL respectively; reactions were kept at temperature of 95 °C for 1.5 h, and being stirred all the time. Series of zinc ferrite crystal fluids were obtained.
TEM observation was used to investigate the morphology and size of the as-prepared ZnFe2O4 nanocrystals, and some typical images are shown in Fig. 3. Two typical morphologies of zinc ferrite crystals, with quite different amounts of oleic acid in the reaction, are shown in Fig. 3. In Fig. 3(a), with 0.1 mL oleic acid as dispersant agent, the ZnFe2O4 crystals presented as spherical or near-spherical shape with particle size of 20-32 nm, the crystals packed relatively close to each other. In Fig. 3(b), with 1.0 mL oleic acid as dispersant agent, the ZnFe2O4 crystals presented relative loose with particle size of 20-45 nm, suggesting the amount of oleic acid used in the reaction plays a key role in the particle size, and oleic acid is an excellent stabilizing agent.
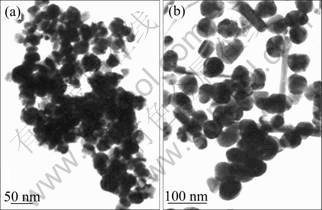
Fig. 3 TEM images of ZnFe2O4 nanocrystals: (a) With 0.1 mL oleic acid; (b) With 1.0 mL oleic acid
3.2 Chemical structure identification of poly-lactide-alanine
Figure 4 shows the IR spectrum of the prepared alanine modified poly lactide, with the mole ratio of lactide to alanine of 97:3. The peak at 1754.45 cm-1 originates from the stretching vibration of C=O of ester bond, peak centered at 1089.54 cm-1 originates from the stretching vibration of —C—O—. Compared with the IR spectrum of pure PLA [19], two new peaks (1681.71 cm-1 and 1540.90 cm-1) are presented in Fig. 4. One peak centered at 1681.71 cm-1 originates from the stretching vibration of C=O in amide, and the peak centered at 1540.90 cm-1 originates from the stretching and bending vibration of N—H in amide. The peaks centered at 1456.95 cm-1 and 1381.67 cm-1 originate from the flexural vibration of methyl (—CH3). The peak centered at 3389.06 cm-1 originates from vibration of NH, and peak at 2994.68 cm-1 originates from vibration of saturated C—H in —CH3. These feature peaks suggest the accomplishment of copolymerization of PLLA.
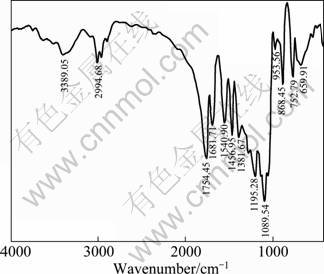
Fig. 4 IR spectrum of poly (D, L-lactide-co-alanine)
With mole ratio of lactide to alanine of 97:3, the copolymerization of lactide and alanine was performed and was tested by NMR, as shown in Fig. 5 (with deuterochloroform as solvent). The 1H NMR peak at about 1.57×10-6 was assigned to methyl protons of D,L lactide, the peak at 5.07×10-6 was assigned to methyne protons of D,L lactide, the peak at 7.25×10-6 was assigned to protons of nitrogen in amide group, and the peaks at about 4.39×10-6 and 4.65×10-6 were assigned to protons of —OCH2 and NHCH2 protons of morpholine-2, 5-dione, respectively. All the 1H NMR peaks are characteristic ones of D, L lactide and alanine, which demonstrate the copolymerization of lactide and alanine successfully. The successful introduction of amino acid group into the PLA chain will facilitate much more application in targeted drug controlling carrier.
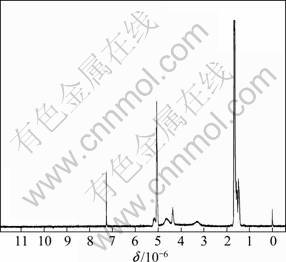
Fig. 5 1H NMR spectrum of poly (D, L-lactide-co-alanine)
3.3 Morphology, size and magnetic property determination of ZnFe2O4/PLLA microspheres
Series TG curves of pure zinc ferrite nanocrystals, oleic acid stabling zinc ferrite nanocrystals, and magnetic PLAA composite microsphere are shown in Fig. 6. As shown in Fig. 6(a), the water loss rate of pure zinc ferrite nanocrystals is 6.3% at temperature of 800 °C. In Fig. 6(b), the loss rate of oleic acid and water is 20.3% at temperature of 800 °C. In Fig. 6(c), from room temperature to 200 °C, the mass loss happened (mainly for water loss); from 200 to 400 °C, magnetic /PLLA particles were in thermal decomposition and an obvious mass loss presented till 400 °C, with a total mass loss
rate of 65.8%. The mass fraction of oleic acid can be calculated by:
w(oleic acid)=w(ZnFe2O4-oleic acid)-w(ZnFe2O4) = 20.3%-6.3%= 14.0% (1)
So, the mass fraction of PLAA in microsphere can be calculated by:
w(PLA)=w(ZnFe2O4–PLAA)-w(ZnFe2O4-oleic acid)=65.8%-20.3%=45.5% (2)
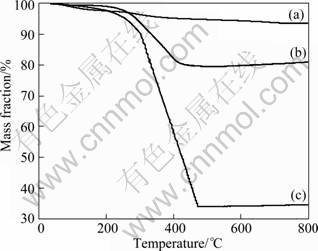
Fig. 6 TGA curves of ZnFe2O4 (a), ZnFe2O4-oleic acid (b), and ZnFe2O4 – PLAA (c)
The mass fraction of PLLA is 45.5%, which is less than the theoretical value (50%), suggesting certain PLLA particle without any magnetic material, which might be removed during magnetic separation.
As shown in Fig. 7, the as-prepared magnetic polymer particles were of characteristic magnetic property, with a coercivity of 398 A/m, a retentivity of 5×10-6 A·m2, and a saturation magnetization of 10.6×10-3 A·m2, which is much less than that of zinc ferrite nanocrystals. The reason may be part of composite microspheres contained no magnetic core. The calculated saturation magnetization percentage of composite microsphere is 33%, which is in good line with the evaluation of TGA, suggesting the zinc ferrite nanocrystals presented stable saturation magnetization during the encapsulation.
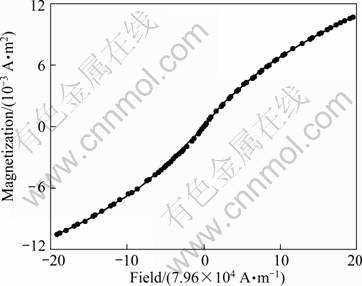
Fig. 7 Magnetization curve of ZnFe2O4/PLLA composite microsphere
Figures 8 and 9 show the SEM image and TEM image of zinc ferrite/PLAA magnetic composite microsphere, respectively. The surface of the as-prepared particles fluctuates a little in Fig. 8, and the particle size ranges from 90 to 350 nm. As shown in Fig. 9, the as-prepared composite particles present to be near- spherical, which are not smooth enough, with particle size of 80-300 nm.
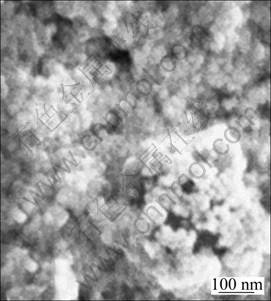
Fig. 8 SEM image of composite microsphere
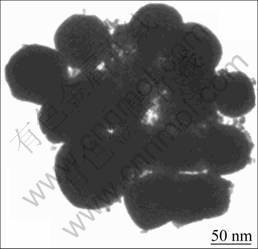
Fig. 9 TEM image of composite microsphere
4 Conclusions
1) Magnetic nano spinel zinc ferrite fliuds stable in oleic acid were synthesized using an improved liquid phase chemical method, with particle size of 20-45 nm, magnetization of 32×10-3A·m2. The effect of amount of oleic acid on dispersion of zinc ferrite nano crystals was studied.
2) Alanine modified poly lactide (PLAA) was achieved with D, L-lactide (D, L-LA) and alanine as monomers and with stannous octoate as initiator.
3) Near-spherical magnetic composite particles were obtained by encapsulating of modified PLA around magnetic zinc ferrite core. The as-prepared particles were endowed with good dispersity, particle size of 80-300 nm, 35% of the composite particle for PLAA. Magnetic property of the composite particles was kept the same as that of pure zinc ferrite nano crystals. The construction of amino acid modified poly lactide magnetic composite particles made it possible to improve biocompatibility of targeted drug controlling carrier.
References
[1] VLADIMIR P T. Targeting of drugs and drug carriers within the cardiovascular system [J]. Advanced Drug Delivery Reviews, 1995, 17: 75-101.
[2] RUUGE E K, RUSETSKI A N. Magnetic fluids as drug carriers: Targeted transport of drugs by a magnetic field [J]. Journal of Magnetism and Magnetic Materials, 1993, 122(1-3): 335-339.
[3] FABRIZIO M, FRAN?OIS L. Active targeting with particulate drug carriers in tumor therapy: Fundamentals and recent progress [J]. Drug Discovery Today, 2004, 9(5): 219-228.
[4] RUDGE S, PETERSON C, VESSELY C, KODA J, STEVENS S, CATTERALL L. Adsorption and desorption of chemotherapeutic drugs from a magnetically targeted carrier (MTC) [J]. Journal of Controlled Release, 2001, 74: 335-340.
[5] ZHAO Yan, ZHAO Ying, WANG Xu-cheng. Progress of application of targeted delivery in tumor therapy [J]. Evaluation and Analysis of Drug-Use in Hospital of China, 2008, 8(1): 8-11. (in Chinese)
[6] ASHUTOSH C, MATTHEW R D, DAN E M. Design of thermally responsive, recombinant polypeptide carriers for targeted drug delivery [J]. Advanced Drug Delivery Reviews, 2002, 54: 1093-1111.
[7] JAE H P, GURUSAMY S, KWANGMEYUNG K, ICK C K. Targeted delivery of low molecular drugs using chitosan and its derivatives [J]. Advanced Drug Delivery Reviews, 2010, 62: 28-41.
[8] SRINIVASAN B, MICHAELJ B R, GEORGE C. Particle size effect on phase and magnetic properties of polymer-coated magnetic nanoparticles [J]. Journal of Magnetism and Magnetic Materials, 2009, 321: 117-122.
[9] RAMANUJAN R V, YEOW Y Y. Synthesis and characterisation of polymer-coated metallic magnetic materials [J]. Materials Science and Engineering C, 2005, 25: 39-41.
[10] ZHANG K, WU W, GUO K, CHEN J F, ZHANG P Y. Magnetic polymer enhanced hybrid capsules prepared from a novel Pickering emulsion polymerization and their application in controlled drug release [J]. Colloids and Surfaces A, 2009, 349: 110-116.
[11] ZHANG Xiao-juan, JIANG Wei, LI Feng-sheng, SUN Zhen-dong, OUYANG Zhi. Controllable preparation of magnetic polymer nanospheres with high saturation magnetization by miniemulsion polymerization [J]. Materials Letters, 2010, 64: 119–121.
[12] NAIRA L S, LAURENCIN C T, CATO T L. Biodegradable polymers as biomaterials [J]. Progress in Polymer Science, 2007, 32: 762-798.
[13] FAHIMA D, ABHALAXMI S, CHANDANA M, SANJEEB K S. Dual drug loaded superparamagnetic iron oxide nanoparticles for targeted cancer therapy [J]. Biomaterials, 2010, 31: 3694-3706.
[14] JANA C, JANA D, DALIBOR H A, VOJTECH A, RENE K, JAROMIR H. Magnetic nanoparticles and targeted drug delivering [J]. Pharmacological Research, 2010, 62: 144-149.
[15] LIU Han-dan, XU Wei, WANG Shi-gang. Mathematical modeling of the magnetic drug targeting delivery [J]. Journal of Shanghai Jiao tong University, 2008, 42(1): 1-4. (in Chinese)
[16] CHENG Deng-miao, ZHAI Xue-liang, XU Yang. Development of magnetic particles for targeting drug [J]. Journal of Hebei Normal University, 2009, 33(5): 649-655. (in Chinese)
[17] XIAO Xu-xian, HUANG Ke-long, YAN Jian-hui, HE Qiong-qiong. Synthesis and characterization of CoFe2O4 nanoparticles [J]. Transactions of Nonferrous Metals Society of China, 2005, 15(5): 1172-1177.
[18] CAO Xue-bo, GU Li. Spindly cobalt ferrite nanocrystals: Preparation, characterization and magnetic properties [J]. Nanotechnology, 2005, 16: 180-185.
[19] SHI Shu-xian, XIA Yu-zheng, GUO Zu-peng, LI Xiao-yu, JIAO Shu-ke. Synthesis and characterization of poly (D, L-lactide) [J]. China Elastomerics, 2002, 12(6): 10-13.
纳米铁酸锌/丙氨酸改性聚乳酸复合磁性微球的制备和表征
曹雷健1, 周勤华2, 谷 俐3, 沈红霞3, 李清华1, 兰 平3, 方 燕3
1. 常州大学 材料科学工程学院,常州 213164;
2. 嘉兴学院 医学院,嘉兴 314001;
3. 嘉兴学院 高分子材料研究所,嘉兴 314001
摘 要:以改进液相化学法合成铁酸锌纳米磁流体来代替传统的铁氧化物磁粉,同时以D, L型丙交酯与丙氨酸为单体进行本体聚合,得到氨基酸改性聚乳酸,再以改性聚乳酸包封纳米磁流体构建磁性高分子微球。采用X射线衍射、傅立叶红外光谱仪、核磁共振仪、扫描电镜、透射电镜、振动样品磁强计、热重分析仪等对所合成的材料进行表征。结果表明:所制备的材料为尖晶石型的ZnFe2O4纳米晶,粒径为20~45 nm,磁饱和强度为32×10-3 A·m2;丙氨酸成功接枝到了聚乳酸链上;铁酸锌纳米磁流体/聚乳酸复合微球的分散性较好,粒径为80~300 nm,聚乳酸的包覆率为45.5%,磁饱和强度为10.6×10-3 A·m2,ZnFe2O4经改性聚乳酸封装后仍然保持较好的磁饱和强度。
关键词:铁酸锌;丙氨酸;聚乳酸;磁性复合微球
(Edited by LI Xiang-qun)
Foundation item: Project (21107032) supported by the National Natural Science Foundation of China; Projects (Y406469, Y4110606) supported by Natural Science Foundation of Zhejiang Province, China; Projects (2008AY2018, 2011AY1048-5, 2011AY1030) supported by the Science Foundation of Jiaxing Science and Technology Bureau, China; Project (2009C21003) supported by Science and Technology Department of Zhejiang Province, China
Corresponding author: GU Li; Tel: +86-573-83646195; E-mail: guli@mail.zjxu.edu.cn
DOI: 10.1016/S1003-6326(11)61184-9