J. Cent. South Univ. (2012) 19: 324-330
DOI: 10.1007/s11771-012-1008-3
Synthesis of Ce-doped yttrium aluminum garnet phosphor by impinging streams co-precipitation
LI You-feng(李友凤), YE Hong-qi(叶红齐), HE Xian-da(何显达), HAN Kai(韩凯), LIU Hui(刘辉)
School of Chemistry and Chemical Engineering, Central South University, Changsha 410083, China
? Central South University Press and Springer-Verlag Berlin Heidelberg 2012
Abstract: Phosphor yttrium aluminum garnet Y3Al5O12 (YAG), activated with trivalent cerium (Ce3+), was synthesized by T-tube impinging streams, T-type vortex impinging streams co-precipitation method (IS-CP) and direct co-precipitation method (D-CP), respectively. The crystallization, morphologies, particle size and particle size distribution of the phosphors obtained under different experimental conditions were studied. The influence of various factors on the luminescence intensity of the phosphor was also investigated, such as feeding methods, volume flow rate, contents of Ce and initial reactant concentration. The results show that the precursors synthesized by T-tube impinging streams co-precipitation reaction transform to Y3Al5O12 (YAG) phosphor at about 1 000 °C. The particles are far smaller and narrower than those prepared by D-CP. In the impinging streams co-precipitation system, the luminescent intensity of YAG:Ce phosphor increases with the increase of liquid flow rate. The intensity firstly increases then decreases with the increasing Ce3+ doping content, and the maximum intensity is shown at 1.67% (molar fraction) Ce. Luminescent intensity gradually decreases with the increase of initial concentration of reactants. At the same operational condition, the luminescent intensity of the phosphors prepared by T-tube impinging streams reactor is higher than that by D-CP, and the luminescent intensity of the phosphors prepared by T-type vortex impinging streams is higher than that by T-tube impinging streams reactor.
Key words: yttrium aluminum garnet: Ce; luminescent properties; impinging streams; co-precipitation; micromixing
1 Introduction
Co-precipitation with a low production cost, ease of mass production and high efficiency, is widely used in industry [1-2]. However, the process often involves rapid chemical reaction. Mixing, particularly the micromixing (namely mixing on the molecular scale) greatly affects the particle size and particle size distribution in the process [3].
Impinging streams (IS) reactor, prepared through micro-machining and precision machining technology, is a small response system. The streams impinge in the microchannel, resulting in an increased mass transfer for reaction and allowing for a decrease in the geometric size of the system [4]. The mixing characteristic size is between several microns and several hundreds microns. So, the length is greatly shortened. Relying on molecule diffusion, IS can realize perfect mixing within extremely short time (millisecond grade). Therefore, IS can provide intensified micromixing which is crucial to create a homogeneous environment for nucleation. Utilizing this advantage for preparing nanometer or submicron inorganic materials with regular morphology is a brand-new field. It has been reported that TiO2, ZnO, and Mg(OH)2 dyes and gold nanoparticles were synthesized by impinging streams reactors [5-10]. MORTEZA and BEHROOZ [11] have investigated the residence time distribution and application of the continuous two impinging streams reactor in liquid-liquid reactions using modeling and experiment methods. CHARINRAT and SAKAMON [12] studied the flow and mixing behavior of impinging streams by numerical simulation.
In this work, the synthesis of Ce3+ doped phosphor yttrium aluminum garnet (YAG:Ce) precursors using corresponding stoichiometric Y3+, Al3+, and Ce3+, with ammonium hydrogen carbonate as precipitant in a direct co-precipitation reactor, T-tube impinging streams reactor and T-type vortex impinging streams reactor were investigated, respectively. Then, the amorphous YAG:Ce precursors converted to YAG:Ce crystals at high temperature. The powders were characterized with X-ray diffraction (XRD), scanning electron microscope (SEM) and luminescence spectrometer. The effects of reactants mixing mode on the particle morphology and size were studied. The effects of feeding method, Ce doping content, flow rate of reactant and initial concentration of reactants on the luminescent intensity of phosphor were also explored.
2 Experimental
2.1 Materials
The starting materials for YAG-based phosphor were rare earth oxide Y2O3 (99.99%, Hunan Non-Ferrous Metals Institute, Changsha), Ce(NO3)3·6H2O (analytical grade, Sinopharm Chemical Reactant Co., Ltd) and Al(NO3)3·9H2O (analytical grade, Tianjin Fine Chemicals). Y2O3 was dissolved in diluted nitric acid (HNO3) and evaporated to remove the surplus HNO3. Ce(NO3)3·6H2O and Al(NO3)3·9H2O were dissolved in deionized water. The ammonium hydrogen carbonate (AHC) as precipitant is of analytical grade. PEG and (NH4)2SO4 are both of analytical grade as the dispersant.
The multi-cation solution was prepared according to stoichiometric proportion of Y3-xCexAl5O12 and the total cation concentration (c0) was 0.055-1.0 mol/L. The nitrate solution (Reactant 1) was mixed in a container and then 1% (mass fraction) disperser (PEG400) and appropriately proportional (NH4)2SO4 were added to the mixture with continuous stirring. The concentration of AHC (Reactant 2) was selected as 1.5 mol/L.
2.2 Powder synthesis
The reaction was operated in semibatch.
1) Direct co-precipitation (D-CP) method
Precursors were produced by dropping 200 mL of the multi-cations solution (Reactant 1) and 200 mL of the precipitant solution (Reactant 2) at the same rate (qv,L) into the stirred tank reactor under strongly mechanical stirring at 80 °C. Resultant suspensions, after aging for 0.5 h at 80 °C, were filtered, washed four times with deionized water, one time with alcohol and dried at 100 °C over 12 h, and then the loose YAG precursors were obtained. Finally, the YAG precursors were calcined in air at 800, 900, 1 000 and 1 100 °C for 2 h, respectively.
2) Impinging streams co-precipitation (IS-CP) method
200 mL of the multi-cations solution (Reactant 1) and 200 mL of the precipitant solution (Reactant 2) were firstly drawn into the impinging streams reactor and then turned into the stirred tank (Fig. 1). Two streams impinged in the microchannel of the impinging streams reactor and produced homogeneous nucleation. Then, the nuclei grew up in the stirred tank reactor. The post- procedure of impinging streams micromixing reaction was the same as that of D-CP.
Figure 2 shows the schematic diagram of the impinging streams reactors. T-tube (T(1)) and a two-inlet vortex mixer (T-type vortex mixer, T(2)) were performed in the experiment. The inlet diameter of nozzle, d1, is 1.0 mm, and the outlet diameter, d2, is 2.0 mm.
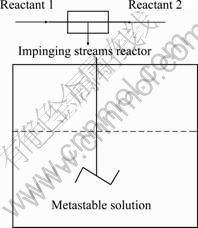
Fig. 1 Flow chart of impinging streams co-precipitation
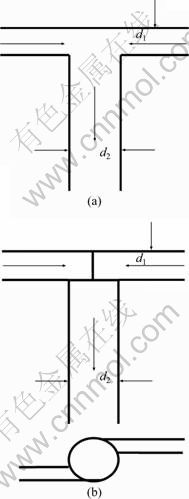
Fig. 2 Schematic diagram of impinging streams reactors: (a) T-tybe; (b) T-type vortex mixer
2.3 Powder characterization
The composition of precursors was assayed by an IRIS Advanced 1000 inductively coupled plasma atomic emission spectrometer (ICP-AES). The phase identification was performed using a conventional D/max X-ray diffractometer with Cu Kα radiation (λ=0.154 18 nm). The morphology of the powders annealed at 1 000 °C was examined with a JEOL JEM-5610LV scanning electron microscope (SEM). The particle size was dealt with software of Nano-measurer 1.2. The emission spectra of all the phosphors were characterized with an F2500 luminescence spectrometer at room temperature.
3 Results and discussion
3.1 Cations contents in precursor
Table 1 gives the effects of feeding methods on the typical composition of cations in the precursors and the variation of pH. The multi-cation solution was prepared according to the stoichiometric proportion of Y2.90Ce0.1Al5O12 and the theoretical ratios of x(Y)/x(Al) and x(Ce)/x(Al) are 58% and 2.0%, respectively. The initial pH is the pH of precipitation solution and the final pH is the pH of solution after precipitation.
Table 1 Effects of feeding methods on composition of cations in precursors and variation of pH
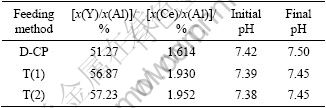
Impinging streams co-precipitation method is in favor of cation complete precipitation and there is more cation loss in the process of direct co-precipitation. It is found that there is little variation in pH value in the process of these co-precipitation methods. So, the effect of pH variation on the co-precipitation process can be omitted and the micromixing rate is the major affecting factor.
3.2 Phase transition during sintering
For YAG phosphor system, the directly synthesized particles in co-precipitation have amorphous phase. Post-treatment process at high temperatures is required for the crystallization of as-prepared particles. In the present work, powders annealed at 800-1 000 °C for 2 h in air were characterized by means of XRD. Figure 3 reveals the XRD patterns of the powder produced by IS-CP method. The powders are found to be amorphous until 800 °C. At 900 °C, different phases which are a mixture of YAG and YAP are observed. At 1 000 °C, the hexagonal YAP (YAlO3) phase disappears and cubic YAG (Y3Al5O12) is the only phase, with crystalline cell constants a=b=c=12.009 ?, which are in good agreement with JCPDS Card (No.33-0040). Refinement of peak shapes and intensities reveals crystallite growth of the powders as the temperature increases.
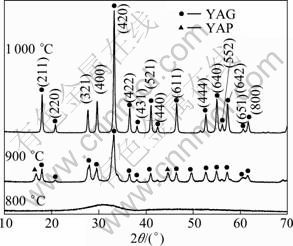
Fig. 3 XRD patterns of Y2.90Ce0.1Al5O12 powders synthesized by T(1) at different temperatures (c0=0.22 mol/L, qv,L=6 mL/min)
3.3 Effects of feeding methods on morphology and size of YAG:Ce phosphors
Figure 4 illuminates the SEM images and particle size distribution (PDS) of YAG:Ce crystals sampled from D-CP and IS-CP under the same technical conditions. It is obvious that phosphor through IS-CP shows quasi-spherical morphology and good dispersity with particle size of 50-100 nm, while particles through D-CP show agglomerate phenomenon and irregular morphology.
Solution supersaturation distribution is the key determining factor for the preparation of nanosized particles in co-precipitation process. The degree of solution supersaturation comes from the reaction, and the reaction process is closely related with the micromixing. That is to say, the generation rate of supersaturation depends on the micromixing rate. From the crystallization kinetics, the nucleation rate of precipitation reaction is strongly nonlinear. Small changes in supersaturation would cause great changes in the nucleation rate, resulting in particle size distribution difficult to control, poor reproducibility between batches, and so on [13-14]. Incorporation mode [15] is used to relate the micromixing time (tm) to the segregation index (Xs) with
. While the induction nucleation period, t, which is milliseconds or less, is often much smaller than tm. So, it is difficulty to control the process in the conventional co-precipitation. According to reaction engineering point of view, when tmm≥t, homogenization of the solution supersaturation and nucleation processes occurs simultaneously. So, it is difficult to control the nucleation process and it is accompanied by significant amplification effects. This behavior was also observed with the iodide-iodate reaction in two micro-mixers [16-17]. The smaller the segregation index Xs is, the better the micromixing effect of the mixer is. The calculated Xs of impinging streams reactor (T(1)) is 0.004 01, compared to 0.604 of the stirred tank reactor. The corresponding mixing time is 4.08 ms in the T-tube impinging streams reactor, while 0.92 s in the stirred tank reactor. Therefore, the micromixing of impinging streams reactors is promoted, and the local supersaturation of the reaction system is enhanced, resulting in smaller particles [18]. And the homogenous mixing is well achieved, which results in the narrower size distribution. It is well demonstrated in the experiments that the measured mean particle size and ranges of particle size distribution decrease as the mixing intensity increases.
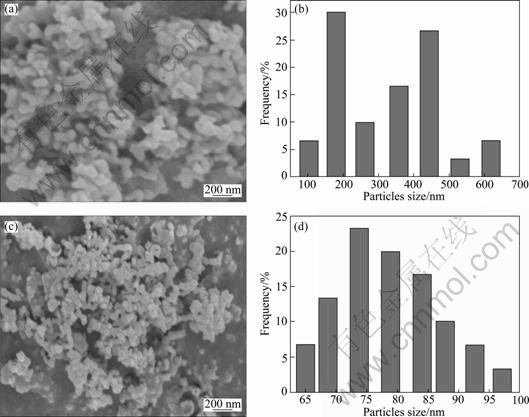
Fig. 4 SEM morphologies and particle size distribution of Y2.90Ce0.1Al5O12 powders synthesized by method of D-CP (a), (b) and T (1) (c), (d) (Sintered at 1 000 °C for 2 h, c0 = 0.22 mol/L, qv, L=6 mL/min)
3.4 Luminescent property of YAG:Ce
It is well known that the quality of phosphor is related not only to its purity but also to its particle size, size distribution, shape and defects [19-22]. Phosphor with fine particle, quasi-spherical, even spherical morphology and high luminescent intensity is desirable.
3.4.1 Effect of feeding method on luminescence properties of YAG:Ce
Emission spectra of phosphors synthesized by D-CP and IS-CP sintered at different temperatures are shown in Fig. 5. The emission of Ce3+ is ascribed to the electron transition from the lowest crystal-splitting component of 5 d level to the ground state Ce3+ (2F5/2, 2F7/2). Phosphor produced by IS-CP method has stronger emission intensity than that by D-CP method. Its luminescent intensity is 7.58% higher than that of the phosphor prepared by the procedure through D-CP method at 1 000 °C, and 3.65% higher at 1 100 °C. This is possibly because the size distribution of phosphors synthesized by IS-CP is more uniform and aggregation is much weaker. In addition, the distribution of Ce3+ ions becomes more homogeneous in YAG lattice in the IS-CP process. This illuminates that the micromixing intensity could decrease the particle size and promote the particles dispersity, which has already been demonstrated in Fig. 4. So, the luminescent intensity is enhanced accordingly with the increasing micromixing intensity of the reactor.
With the raising temperature, the emission intensity increases. This is attributed to the enhancement of crystallization degree and the decrease of surface defects [23]. The maximum emission wavelength hardly changes in spite of phosphor synthesized by different precipitation methods and sintered at different temperatures.
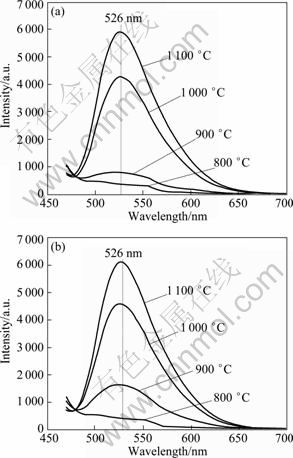
Fig. 5 Emission (λex=450 nm) spectra of Y2.90Ce0.1Al5O12 phosphors synthesized by method of D-CP(a) and T(1) (b) (c0 =0.22 mol/L, qv,L=6 mL/min)
3.4.2 Effect of feeding rate on luminescence properties of YAG:Ce
The effect of feeding rate on the luminous intensities of particles is demonstrated in Fig. 6. The luminescence of particles increases with the increase of feeding rate.
Reynolds numbers Re (Re=ρqv/0.785μd) are about 456, 228 and 547.2 in the mixing channel of impinging streams reactor when the flow rates are 10 mL/min, 5 mL/min and 12 mL/min in the experimental conditions, respectively. KOCHMANN et al [24] found that the flow located in the transition zone at Re 250-300. When Re is higher than 400, the flow exists as turbulence state in the T-shaped microchannel reactor. For small reaction pore size, the flow is mixed mainly on diffusion when the flow is laminar at low rate and efficient mixing is achieved by diffusion combined with turbulence at high flow velocity of turbulent state. So, micromixing time is shortened and the degree of supersaturation uniformity is improved with the increase of the fluid turbulence intensity, which in turn results in small particle size, narrow particle distribution, and favors the doping Ce3+ into YAG lattice. So, the luminescent intensity increases with the increasing flow rate. However, when the flow rate increases to a certain value, the micromixing rate is very fast, the impact of micromixing is weakened and nucleation process is controlled kinetically. Consequently, the luminous intensities of particles change little with increasing the flow rate at this time.
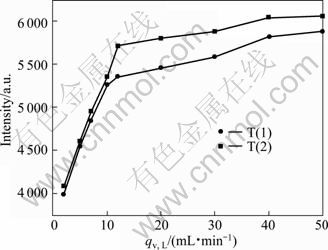
Fig. 6 Emission (λex=450 nm) spectra of Y2.90Ce0.1Al5O12 phosphors synthesized by T(1) and T(2) at different feeding rates (Sintered at 1 000 °C for 2 h, c0=0.22 mol/L)
Vortex mixer could produce self-rotation effect. When the Reynolds number is higher than 2.36, a self-rotation effect is induced in the circular microchamber, which generates a three-dimensional vortex [25]. The turbulence degree of fluids is greatly increased and the mixing performance is improved accordingly. In addition, vortex results in extra contact areas which could guarantee high mass transfer rates in the subsequent micromixer. So, vortex results in superior product property and more Ce doped in the products, and the luminescent intensity of the phosphors prepared by vortex mixer (T(2)) is higher than that by T-tube impinging streams reactor (T(1)) in the same operational conditions.
3.4.3 Effect of Ce contents on luminescence properties of YAG:Ce
Figure 7 shows the emission spectra of Y3-xCexAl5O12 particles at different doping contents of Ce. The as-prepared particles were annealed at 1 000 °C for 2 h. The emission intensity does not increase monotonically with the cerium content as mentioned in previous reports [26]. The intensity firstly increases then decreases with the increasing Ce3+ doping content. The maximum luminescent intensity is shown at 1.67% (molar fraction) Ce (x=0.083%). This is because, at low content, the number of luminescent centers gradually increases in the YAG matrix with the increasing Ce3+ doping content and the corresponding emission intensity increases. While at high content, the intensity decreases because of incomplete activation of Ce under the above mentioned annealing conditions and leads to coexistence of YAG and CeO2.
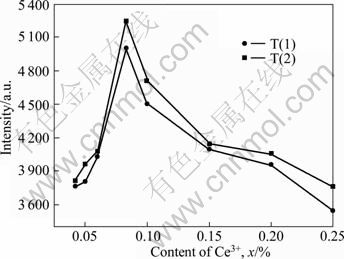
Fig. 7 Emission (λex=450 nm) spectra of Y3-xCexAl5O12 phosphors prepared with different doping contents of Ce3+ (Sintered at 1 000 °C for 2 h, c0=0.22 mol/L, qv,L=6 mL/min)
3.4.4 Effect of reactant concentration on luminescence properties of YAG:Ce
The effect of reactant concentration on the luminous intensities of YAG:Ce phosphor is shown in Fig. 8. The luminous intensity decreases as initial concentration of reactants increases. The initial concentrations of the reactants have no significant effect on emission intensity at low concentration. This might be due to the very high degree of supersaturation generated in this experiment for the concentrations studied. Under these conditions, the main action is the very fast primary nucleation, so the particles are very small and the concentrations show little effect on the particle size and morphology, and the luminous intensity changes little. On the other hand, with the increase of concentration, the change in the emission intensity is noticeable. It may have been caused by the slight agglomeration among particles and the formation of CeO2.
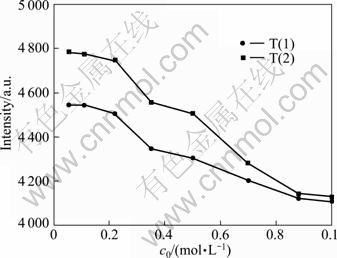
Fig. 8 Emission (λex=450 nm) spectra of Y2.90Ce0.1Al5O12 phosphors synthesized by T(1) and T(2) at different initial concentrations (Sintered at 1 000 °C for 2 h, qv,L=6 mL/min)
4 Conclusions
1) The YAG:Ce phosphors with quasi-spherical morphology and good dispersity are prepared by impinging streams co-precipitation, which are far smaller and narrower than those prepared by direct co-precipitation method. Phosphor produced by impinging streams co-precipitation method has higher emission intensity than that by D-CP. Increasing the sintering temperature could promote the luminous intensity of the phosphor.
2) In the YAG:Ce phosphor system, the optimum preparation conditions for high emission intensity are investigated. The luminescent intensity of YAG:Ce particles increases when the flow rate of reactants increases. The luminescence intensity firstly increases then decreases with the increasing content of Ce. The luminescence intensity decreases as initial concentration of reactants increases. Under the same operational conditions, the luminous intensity of the phosphors prepared by vortex mixer is higher than that by T-tube impinging streams reactor.
References
[1] ZHANG Yong-ming, YU Hong-ming. Synthesis of YAG by the co-precipitation method [J]. Ceramics International, 2008, 7: 1-5.
[2] LEE M H, KANG Y J, MYUNG S T. Synthetic optimization of Li [Ni1/3Co1/3Mn1/3]O2 via co-precipitation [J]. Electrochimica Acta, 2004, 50(4): 939-948.
[3] DANCKWERTS P V. The effect of incomplete mixing on homogeneous reactions [J]. Chemical Engineering Science, 1958, 8(1): 93-101.
[4] GUO Sheng-chang, EVANS D G, LI Dian-qing, DUAN Xue. Experimental and numerical investigation of the precipitation of barium Sulfate in a rotating liquid film reactor [J]. AIChE J, 2009, 55(8): 2024-2034.
[5] WANG H Z, Nakamura H, Uehara M. Preparation of titania particles utilizing the insoluble phase interface in a microchannel reactor [J]. Chemical Communications, 2002, 2(14): 1462-1463.
[6] KIWAMU S, KAZUHITO K, KUNIO A. Hydrothermal synthesis of ZnO nanocrystals using microreactor [J]. Materials Letters, 2004, 58(25): 3229-3231.
[7] SHIRURE V S, PORE A S, PANGARKAR V G. Intensification of precipitation using narrow channel reactors: Magnesium hydroxide precipitation [J]. Ind Eng Chem Res, 2005, 44(15): 5500-5507.
[8] PENNEMANN H, FORSTER S, KINKEL J, HESSEL V, LOWE H, WU L. Improvement of dye properties of the azo pigment yellow 12 using a micromixer-based process [J]. Org Proc Res Dev, 2005, 9(2): 188-192.
[9] WAGNER J, K?HLER J M. Continuous synthesis of gold nanoparticles in a microreactor [J]. Nano Letters, 2005, 5(4): 685-691.
[10] YING Y, CHEN G W, ZHAO Y C, LI S L, YUAN Q. A high throughput methodology for continuous preparation of monodispersed nanocrystals in microfluidic reactors [J]. Chem Eng J, 2008, 135(3): 209-215.
[11] MORTEZA S, BEHROOZ Z. Modeling of the residence time distribution and application of the continuous two impinging streams reactor in liquid-liquid reactions [J]. Chem Eng Technol, 2005, 28(1): 61-66.
[12] CHARINRAT S, SAKAMON D. Numerical simulation of flow and mixing behavior of impinging streams of shear-thinning fluids [J]. Chemical Engineering Science, 2006, 61(15): 4884-4892.
[13] CARLOS M P, ENDERS M, PUTNIS A. The composition of solid solutions crystallizing from aqueous solutions: The influence of supersaturation and growth mechanisms [J]. Chemical Geology, 2000, 168(3): 195-210.
[14] JIA Zhi-qian, LIU Zhong-zhou, HE Fei. Synthesis of nanosized BaSO4 and CaCO3 particles with a membrane reactor: Effects of additives on particles [J]. Journal of Colloid and Interface Science, 2003, 266(2): 322-327.
[15] FOURNIER M C, FALK L, VILLERMAUX J. A new parallel competing reaction system for assessing micromixing efficiency-determination of micromixing time by a simple mixing model [J]. Chem Eng Sci, 1996, 51 (23): 5187-5192.
[16] FOURNIER M C. A new parallel competing reaction system for assessing micromixing efficiency—Experimental approach [J]. Chem Eng Sci, 1996, 51(22): 5053-5064.
[17] GUICHARDON P, FALK L. Characterization of micromixing by the iodide—Iodate reaction system. (Part I) [J]. Experimental Procedure, Chem Eng Sci, 2000, 55(19): 4233-4243.
[18] PHILLIPS R, ROHANI S, BALDYGA J. Micromixing in a single-feed semibatch precipitation process [J]. AIChE J, 1999, 45(1): 82-92.
[19] JONG S B, SUNG B K, JUNG H J, JUNG C P, DONG K K, SONGH B, SOUNG Y. Photoluminescence characteristics of Li-doped Y2O3:Eu3+ thin film phosphors [J]. Thin Solid Films, 2005, 471: 224-229.
[20] LI Xia, LI Qiang, WANG Ji-yang, YANG Shun-liang. Effect of process parameters on the synthesis of YAG nano-crystallites in supercritical solvent [J]. Journal of Alloys and Compounds, 2006, 421(1/2): 298-302.
[21] ZHANG Shu-sheng, ZHUANG Wei-dong, HE Tao. Study on co-precipitation synthesized Y3Al5O12: Ce yellow phosphor for white LED [J]. Journal of Rare Earth, 2010, 8(5): 713-716.
[22] ZHANG Jia-hua, JIA Ming-li, LU Shao-zhe, LUO Yong-shi, REN Xin-guang, WANG Xiao-jun. Study on UV excitation properties of Eu3+ at the S6 site in bulk and nanocrystalline cubic Y2O3 [J]. Journal of Rare Earths, 2004, 22(1): 45-48.
[23] AGEETH A B, ANDRIES M. Luminescence quantum efficiency of nanocrystalline ZnS:Mn2+: Surface passivation and Mn2+ concentration [J]. J Phys Chem B, 2001, 105(42): 10197-10202.
[24] KOCKMANN N, DREHER S, WOIAS P. Unsteady laminar flow regimes and mixing in T-shaped micromixer [C]// Proceedings of the Fifth International Conference on Nanochannels, Microchannels and Minichannels, Puebla: ASME, 2007: 18-20.
[25] LIN C, TSAI C, AND FU L. A rapid three-dimensional vortex micromixer utilizing self-rotation effects under low Reynolds number conditions [J]. Journal of Micromechanics and Microengineering, 2005, 15(5): 935-943.
[26] YUN Chan-kang, LENGGORO I W, PARK S B, OKUYAMA K. YAG:Ce phosphor particles prepared by ultrasonic spray pyrolysis [J]. Materials Research Bulletin, 2000, 35(5): 789-798.
(Edited HE Yun-bin)
Foundation item: Project(200805330032) supported by the Natural Educative Doctoral Foundation of China; Projects(20080440987, 200902475) supported by the China Postdoctoral Science Foundation
Received date: 2011-05-27; Accepted date: 2011-07-06
Corresponding author: YE Hong-qi, PhD, Professor; Tel: +86-731-88876605; E-mail: liyoufeng88@yahoo.com.cn