
Characterization and wear resistance of macro-arc oxidation coating on magnesium alloy AZ91 in simulated body fluids
CHEN Jun (陈 君), ZENG Rong-chang (曾荣昌), HUANG Wei-jiu (黄伟九),
ZHENG Zi-qing (郑自芹), WANG Zhen-lin(王振林), WANG Jun (王 俊)
School of Materials Science and Engineering, Chongqing Institute of Technology, Chongqing 400050, China
Received 12 June 2008; accepted 5 September 2008
Abstract: The mechanical characteristics of the macro-arc oxidation (MAO) coating on Mg alloy AZ91 were examined by means of nano scratch tester. The corrosion and erosion corrosion behavior of AZ91 with and without MAO coating were investigated by using potentiodynamic electrochemical technique and micro-abrasion tribometer in simulated body fluids, respectively. The influence of HCO3- ions on the erosion corrosion was discussed. The results show that the coating and its substrate are in a pronounced bond. The MAO coating increases 1-2 orders of magnitude of the corrosion resistance of AZ91 alloy. HCO3- ions enhance the corrosion rates of the AZ91 alloys more significantly than the alloys with MAO coating. However, there exists an obvious passivation process of AZ91 without coating in the HCO3- solutions. Moreover, an MgCO3 film formed in HCO3- containing solutions leads to an enhancement in micro-wear resistance. MAO coating deteriorates the erosion corrosion resistance of AZ91 alloy due to the formation of oxidation debris resulted from the broken MAO coating.
Key words: magnesium alloy; macro-arc oxidation; coating; erosion corrosion; biomaterial; simulated body fluid
1 Introduction
Mg alloys are very promising implanted materials because of its excellent biocompatibility and mechanical compatibility[1-3]. Unfortunately, Mg alloys rapidly degrade in human body, the lives of Mg alloy implants exist merely several weeks[2]. In addition, Mg alloys have low surface hardness and poor wear resistance[4]. Thus, it is necessary to find a suitable coating to improve their erosion corrosion resistance. Scientists recently try to develop various coatings such as hydroxyapatite (HA) for medical application. For instance, ABDULLAT et al[5], LI and GAO[1, 6] applied NaHCO3 and Na2CO3 or MgCO3 to modify the surface of Mg and obtained HA coating in simulating body fluid (SBFs). Their results show that only NaHCO3 can improve the corrosion resistance. Another research by M?LLER et al[7] indicated that the HCO3- content in SBF influenced the composition and structure of the calcium phosphates. It can be supposed that as long as the HCO3- concentration in the testing solutions is lower than 20 mmol/L, only B-type hydroxyl carbonate apatite (HCA) precipitates. At higher HCO3- concentrations, it can be assumed that A-type HCA forms. Herein, HCO3- ions in SBFs have crucial influence on the corrosion behavior of Mg alloys[7-9]. The above studies are still at preliminary stage, further investigations on bio-coatings are required. It is demonstrated that macro-arc oxidation (MAO) or plasma electrolytic oxidation (PEO) coatings have good corrosion resistance[10-11] or wear resistance of Mg alloys in air[4, 8] and SBF[10]. There are, however, no literatures dealing with the characteristics of MAO coating on Mg alloys and the interaction of corrosion and wear of Mg alloys with MAO coatings in SBFs. It is therefore imperative to investigate the mechanical properties of MAO coating and the influence of solution chemical composition, such as corrosion. This paper aims to probe the erosion corrosion behavior of AZ91 Mg alloy with MAO coatings in SBFs and characterize the mechanical properties of the MAO coatings on Mg alloys.
2 Experimental
The material used in this study was Mg alloy AZ91 (8.5%-9.5% Al, 0.45%-0.90% Zn, 0.17%-0.50% Mn, Mg Balance). The surface roughness and mechanical properties of the anodized coating were examined by virtue of Rochwell nano scratch tester. An EG & G Model 273 Potentiostat was utilized to study the electrochemical corrosion behavior. Three-electrode system was applied. The working electrode exposed a surface area of 2.84 cm2, a saturated calomel electrode (SCE) and a platinum plate were used as reference electrode and auxiliary electrode, respectively. The polarization measurements started after the samples were immersed in the solution for 5 min. The potential was scanned from -400 mV to +400 mV versus open current potential (φcorr) with a scanning rate of 0.5 mV/s. Micro-abrasive wear tests were executed through a TE66 SLIM micro-abrasion tribometer at a speed of 75 r/min for 10 min. ZrO2 ceramic balls and SiC particles with diameters of 25.4 and 4.0 mm were utilized as the friction partner and the abrasive with a concentration of 0.25 g/cm3, respectively. The applied load was 0.05-1.0 N. All wear tests were performed at room temperature ((24±2) ℃). The worn scar profiles and their morphologies were examined by digital profilometer and JSM-5600LV scanning electron microscope (SEM), respectively. In this study, solution Ⅰ, Ⅱ and Ⅲ corresponded to 0.9% NaCl solution, 0.9% NaCl +0.35 g/L NaHCO3 solution and 0.9% NaCl +0.7 g/L NaHCO3 solutions with pH value of 7.52, 8.48 and 8.50, respectively.
3 Results and discussion
3.1 Characteristics of MAO coating
Fig.1 shows the depth or load as function of sliding displacement of MAO coating. The pre-scan curve of scan depth versus sliding distance without applied load is shown as curve 1, by which the surface roughness of coating can be characterized. The coating roughness, is approximately 2.14 μm. Curve 2 corresponds to the scratch curve, which characterizes the bonding adhesion between the coating and substrate. It is apparent that there is a great fluctuation, which indicates the critical abruption value of the coating. And thus the longitudinal coordinate of point B on curve 2 corresponds to the coating thickness (17.6 μm), which is a bit larger than the average thickness value of 14.7 μm, which is measured by optical microscopy. After sliding 500 μm, the load is applied, and then the scratch depth increases with ever-increasing load. Thus, the depth—distance curve increases gradually, and plastic deformation occurs on the MAO film. There exists a significant variation in the depth curve when sliding 2.724 μm to point A. This phenomenon implies that the scratch head may penetrate across the coating to the substrate. The load value corresponds to point A is the critical adhesive force, which is equal to 1.02 N. The hardness and elastic modulus of the MAO coating are 4.2 GPa (about HV 400) and 71.1 GPa, respectively. It is noteworthy that the hardness and elastic modulus of MAO coating are considerably higher than those of the substrate material, which are HV 90 and 45 GPa, respectively.
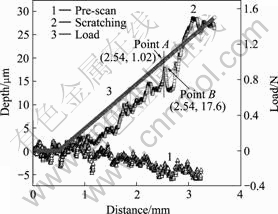
Fig.1 Depth or load as function of sliding displacement of MAO coating through nano-scratch tester: Curve 1 showing pre-scan depth versus distance without applied load; Curve 2 and curve 3 exhibit scratch curve and curve of load vs sliding distance, respectively
3.2 Corrosion behavior of MAO coating
Free corrosion potential (φcorr) vs time curves for the AZ91 substrate and its MAO coating in solutions Ⅰ and Ⅱ are shown in Fig.2(a). It is evident that φcorr of the substrate alloy rapidly increases in the earlier immersion, and then slightly decreases in both solutions. While φcorr of AZ91 with MAO coating declines and then rises continuously in both solutions, probably due to the fact that the MAO coating on the surface is attacked, and thus Mg(OH)2 precipitate forms. It is obvious that φcorr of both AZ91 with and without MAO coating in solution Ⅰ are much higher than that in solution II. The previous studies[12-14] demonstrated that the films on Mg alloys, mainly composed of Mg(OH)2, were quickly produced after immersion in the NaCl solution, whereas a MgCO3?3H2O film could be developed in the solution containing HCO3-[15]. Accordingly, MgCO3 film was more compact than Mg(OH)2 film[13]. In this study, however, HCO3- ions decrease the free corrosion potentials. The cause needs further investigation. Fig.2(b) exhibits the polarization curves of AZ91 with and without MAO coating in the solutions Ⅰ and Ⅱ. It is clear that MAO coating significantly improves the corrosion resistance of Mg alloy AZ91. The corrosion rate decreases 1-2 orders of magnitude, compared with that of the substrate alloy.
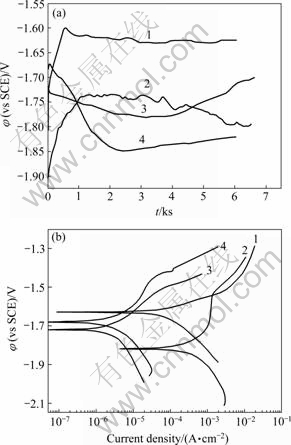
Fig.2 Curves of potential vs time(a) and polarisation curves(b) of AZ91 with and without MAO coating in solutions Ⅰ and Ⅱ: 1—AZ91 in solution Ⅰ; 2—AZ91 in solution Ⅱ; 3—AZ91 with coating in solution Ⅰ; 4—AZ91 with coating in solutionⅡ
It is noticeable that HCO3- ions have a considerable influence on the corrosion behavior of Mg alloys. For AZ91 with and without coating, the corrosion rates increase from 4.00×10-6 and 6.53×10-5 A/cm2 in solution Ⅰ to 3.81×10-6 and 3.04×10-4 A/cm2 in solution Ⅱ. Therefore, HCO3- ions enhance the corrosion rates of the AZ91 alloy more significantly than the MAO one. It is worthy to notice that the AZ91 alloy can be passivated between -1.70 V(vs SCE) and -1.53 V(vs SCE) in solution Ⅱ. This indicates that HCO3- ions inhibit the alloy without MAO coating from corrosion due to the formation of a protective and compact Mg carbonate film.
3.3 Erosion corrosion property of MAO coating
The magnitude of worn scar diameter can characterize the anti-wear property of a material. Worn scar diameters of AZ91 with and without MAO coatings as function of loads in different solutions are shown in Fig.3. It is obvious that there is an onset, corresponding to a load of 0.15 N in each scar diameter vs load curve. The diameters of the wear scar increase rapidly up to the onset and then decrease with increasing loads, ignorance of one exception of AZ91 with MAO coating in solution Ⅰ at the load of 1.0 N. In the initial stage as the load reaches up to 0.15 N, an abrasion groove originally evolves in the scar center, then is size enlarges with the increasing load. Moreover, the wear scar diameters of the alloys with MAO are bigger than that of the materials without MAO coating under the same conditions due to the brittle coating easily cracked at a compressed stress. Herein, the broken oxide debris strip off from the coating as the slurry accelerates abrasive wear damage. In the latter stage, the exposed surface of the coated alloy is subjected to less wear. This results from the peeled oxide pieces left away off the contact area with increasing load. As a result, the wear resistance of AZ91 with MAO coating is not as good as what is expected. On the contrary, the alloy without MAO coating demonstrates a better wear resistance. All wear scar diameters of the samples in the solutions with HCO3- are smaller than that in NaCl solution, and are the smallest in solution Ⅱ. This indicates that MgCO3 film formed in HCO3- containing solutions can be helpful to resisting micro- wear. However, the anti-wear property is not superior in higher hydrogen carbonate ions to that in lower ones.
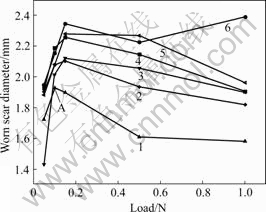
Fig.3 Worn scar diameters of AZ91 with and without coating in various solutions as functions of load: 1—Substrate in solution Ⅱ; 2—Substrate in solution Ⅲ; 3—MAO in solution Ⅱ; 4—Substrate in solution Ⅰ; 5—MAO in solution Ⅲ; 6—MAO in solution Ⅰ
From the worn scar morphologies, it can be found that a variety of corrosion pits exist. The smaller the wear scar diameter, the deeper the grinding groove on the worn surface with increasing loads under the same conditions. And corrosion pits can be seen in the wear scar interior. With increasing loads, the interaction of corrosion and wear is enhanced. The ceramic ball rotation accelerated the process of mass transport, and strengthens the role of corrosion at the same time. As the loads increase up to a critical value, i.e. 0.15 N, the materials surface or subsurface would be subjected to a severe shear stress and result in a strong plastic deformation, and subsequently fine micro-cracks occur on the region contacted with the grinding ball. Hence the surface is roughened, thereby the wearing is facilitated, and the abrasive groove is deepened and extended.
4 Conclusions
1) The critical bonding force of Mg alloy AZ91 with MAO coating reaches 1.11 N. The hardness (4.2 GPa) and elastic modulus (71.1 GPa) of MAO coating are considerably higher than those of the substrate.
2) MAO coating improves the corrosion resistance of Mg alloy AZ91, however, its erosion corrosion resistance decreases due to the formation of wear debris resulting from the brittle MAO film and the interaction between the electrochemistry and mechanics.
3) HCO3- ions enhance the corrosion rate of the AZ91 substrate more significantly than that of the alloy with MAO. They can inhibit the alloy without MAO coating from corrosion due to the formation of a protective and compact Mg carbonate film and a passivation process appears. HCO3- ions can enhance the wear resistance of AZ91 with and without MAO coating. But there is a critical HCO3- content for increase in wear resistance.
References
[1] LI L C, GAO J C, YONG W. Evaluation of cyto-toxicity and corrosion behavior of alkali-heat-treated magnesium in simulated body fluid[J]. Surface & Coating Technology, 2004, 185: 92-98.
[2] WITTE F, KAESE V, HAFERKAMP H, SWITZER E, MEYER-LINDENBERG A., WIRTH C J, WINDHAGEN H. In vivo corrosion of four magnesium alloys and the associated bone response[J]. Biomaterials, 2005, 26(17): 3557-3563.
[3] STAIGER M P, PIETAK A M, HUADMAI J, DIAS G. Magnesium and its alloys as orthopedic biomaterials: A review[J]. Biomaterials, 2006, 27(9): 1728-1734.
[4] HUANG W J, LIU M, LI Z F, ZENG R C. Thickness effects on corrosion and wear resistance properties of micro-arc discharge oxide coatings on AZ91D magnesium alloys[J]. Trans Nonferrous Met Soc China, 2006, 16(s2): s1827-s1830.
[5] ABDULLAT Y A, TSUTSUMI S, NAKAJIMA N, OHTA M, KUWAHARA H, IKEUCHI K. Surface modification of Mg by NaHCO3 and corrosion behavior in Hank’s solution for new biomaterial applications[J]. Materials Transactions, 2001, 42(8): 1777-1780.
[6] GAO J C, LI L C, WANG Y. Surface modification on Mg by alkali-heat-treatment and its corrosion behaviors in SBF[J]. The Chinese Journal of Nonferrous Metals, 2004, 14(9): 1508-1513. (in Chinese).
[7] M?LLER L, M?LLER F A. Preparation of SBF with different HCO3- content and its influence on the composition of biomimetic apatites[J]. Acta Biomaterialia, 2006, 2(2): 181-189.
[8] SONG G., ATRENS A, ST. JOHN D, WU X, NAIM J. The anodic dissolution of Mg in chloride and sulphate solutions[J]. Corros Sci, 1997, 39: 1981-2004.
[9] ZHAO H, LIU Z, CHEN L J, CHEN J, HAN Z. Wear resistance of ceramic coating on AZ91 magnesium alloy by micro-arc oxidation[J]. Trans Nonferrous Met Soc China, 2006, 16(s2): s1814-s1818.
[10] ZHANG X P, ZHAO Z P, WU F M, WANG Y L, WU J. Corrosion and wear resistance of AZ91D magnesium alloy with and without microarc oxidation coating in Hank’s solution[J]. J Mater Sci, 2007, 42: 8523-8528.
[11] ZENG R C, CHEN J, DIETZEL W, HORT N, KAINER K U. Electrochemical behavior of Mg alloys in simulated body fluids[J]. Trans Nonferrous Met Soc China, 2007, 17(s1): s166-s170.
[12] ZENG R C, HAN E H, KE W. Corrosion of artificial aged Mg alloy AZ80 in 3.5wt% NaCl solution[J]. J Mater Sci Technol, 2007, 23(3): 353-358.
[13] XIN Y, LIU C, TANG G., TIAN X, CHU P K. Corrosion behavior of biomedical AZ91 Mg alloy in simulated body fluids[J]. J Mater Res, 2007, 22(7): 2004-2011.
[14] HARA N, KOBAYASHI Y, KAGAYA D, AKAO N. Formation and breakdown of surface films on Mg and its alloys in aqueous solutions[J]. Corros Sci, 2007, 49: 166-175.
[15] GULBRABDSEN E. Anodic behavior of magnesium in HCO3-/CO32- buffer solutions quasi-steady measurements[J]. Electrochimica Acta, 1992, 37(8): 1403-1412.
(Edited by LONG Huai-zhong)
Foundation item: Project(50405005) supported by the National Natural Science Foundation of China; Project(NCET-05-0777) supported by the New Century Excellent Talents in University, China; Project(8655, CSTC 2008BB0063) supported by the Natural Science Foundation of Chongqing Science and Technology commission, China
Corresponding author: ZENG Rong-chang; Tel: +86-23-68665616; E-mail: rczeng2001@yahoo.com.cn