J. Cent. South Univ. Technol. (2008) 15(s1): 443-446
DOI: 10.1007/s11771-008-396-x

Viscoelasticity of mixed polyacrylamide solution
XU Li-na(徐丽娜)
(PetroChina Daqing Oilfield Engineering Co. Ltd, Daqing 163712, China)
Abstract: The viscoelastic behavior of polyacrylamide solution is crucial for its application in various industries. The mixed polyacrylamide solution was prepared by mixing polyacrylamide with different relative molecular masses according to the defined mass fraction. The viscosity and elasticity of mixed polyacrylamide solution were separately tested with RS150 rheometer and capillary breakup extensional rheometer and compared with those of the single polyacrylamide solution which is directly provided by manufacturer without any mixing. The results indicate that the mixed and single polyacrylamide solutions have the same shear viscosity and intrinsic viscosity. However, some mixed polyacrylamide solutions have higher elasticity than single polyacrylamide solution. The flow resistance of mixed polyacrylamide with higher elasticity is also greater than that of single polyacrylamide solution in porous medium. This paper presents an effective method of mixing polyacrylamides with different relative molecular masses, which can enhance the elasticity of polyacrylamide solution and flowing resistance through porous medium.
Key words: polyacrylamide; viscosity; elasticity; flow resistance
1 Introduction
Polyacrylamide is a high molecular mass, good water soluble, anionic homopolymer of acrylamide monomer. It features high viscosity at low shear rate, a viscosity decreasing with shear rate increase (shear- thinning), and shear degradation, which have made it an appealing product to be used in a variety of industries including food, water treatment and agricultural industries. Especially in petroleum industry, the polyacrylamide flooding is the most promising technique for enhancing oil recovery[1].
In addition to its shear-thinning property, polyacrylamide solution is known to be elastic. However, The research on elasticity of polymer solution is much less than that on viscosity. In Refs.[2-3] the viscoelasticity of polymer solution, viscosity and elasticity were regarded as one property—bulk viscoelaticity and the viscoelaticity increased with increasing molecular mass and concentration of polymer, and decreased as the addition of electrolyte. In this paper, a series of mixed polyacrylamide solutions were prepared by mixing polyacrylamide with different relative molecular masses and their rheological properties were investigated.
There exists elastic component of polymer solution when it is subjected to shearing, compression and elongation. Corresponding to these actions, the elasticity has been described with storage modulus[4], normal stress or first normal stress difference, and extension. Each parameter reflects one aspect of elasticity and it is deficient to describe the elasticity of polymer solution simply by one parameter. In this paper, the elasticity was characterized by the storage modulus and extensional breakup time.
2 Experimental
2.1 Materials
Polyacrylamides used in the experiments were commercially available products provided by Daqing Chemical Agent Plant. They were straight chain, partially hydrolyzed, with the average molecular masses of 1 547× 106, 2 778×106, 3 304×106, denoted by P1, P2, P3 respectively, and an average degree of hydrolysis of 23%. The NaCl concentration in brine water was 2.41 g/kg. Other materials were common laboratory agents.
2.2 Methods
2.2.1 Preparation of mixed polyacrylamide solutions
Mixed polyacrylamide solutions denoted by M1, M2, M3 and M4 were prepared by mixing three kinds of polyacrylamide P1, P2, P3 respectively, according to the calculated mass fraction. We first, specified the mass fractions of P2 as 20%, 35%, 65%, 80% respectively, and the mass fractions of P1 and P3 were calculated according to
(1)
where Mm is equal to 2 778×106, being the molecular mass of P2; Mi is the relative molecular mass of Pi, and xi is its mass fraction. Table 1 lists the mass fractions for each mixed polyacrylamide and includs data on apparent shear viscosity(η), intrinsic viscosity([η]), and surface tension(γ) of polymer solutions.
Firstly, P1, P2 and P3 powders were weighed according to the mass fractions in Table 1, then the weighed polymer powders were mixed with brine water to obtain the desired polymer concentration (0.1%, mass fraction), stirred with a mechanical stirrer for 2 h and filtered through 75 μm filter. The polymer solutions were allowed to sit for at least 24 h before measuring the rheological properties.
2.2.2 Measurement of extensional breakup time
The breakup time of polymer solution was measured by the HAAKE capillary breakup extensional rheometer-or CaBER for brevity. STELTER et al[5] and WUNDERLICH et al[6] described the simple principle of the CaBER. A small quantity of sample (less than 0.2 mL) was placed between two parallel plates (6 mm in diameter). The fluid was then exposed to a rapid extensional step strain by moving the upper plate upwards, thereby forming a fluid filament. A laser micrometer measured the midpoint diameter of the gradually thinning fluid filament after the upper plate reached its final position. The measured data described the evolution of the filament diameter as a function of time and the filament breakup time (corresponding to zero filament diameter). For viscoelastic solutions, the filament evolution as a function of time follows a rapid initial viscous-dominated phase, and then there is an intermediate time-scale in which the dynamics of the filament drainage is governed by a balance between surface tension and elasticity, rather than fluid viscosity[7-8]. The surface tension tries to “pinch off” the filament, while the elasticity of the fluid tries to prevent this process. So by comparing the surface tension, extensional breakup time of the polyacrylamide solution, we could investigate the elasticity of polymer solution. At the same surface tension, the long filament breakup time indicates high elasticity of the polyacrylamide solution.
2.2.3 Storage modulus measurement
Storage modulus measurements of polymer solutions were carried out with HAAKE RheoStress RS150 under controlled stress mode. The modulus data were collected using cone-plate sensor. The sensor system consisted of a cone and plate with a diameter of 60 mm and cone angle of 0.5?. The assigned stress was 0.2 Pa within the experimental period of 180 s.
For each fluid listed in Table 1, we measured the viscosity using a viscometer(Brookfield, Model DV-II+) in a constant shear rate of 7.34 s-1. According to the method in Ref.[9], the intrinsic viscosity was obtained (from the point of intersection) after extrapolation with the help of Ubbelohde viscometer (Cannon Instrument Co.). Surface tension measurement was carried out with tensiometer K12 (Kruess Company, Germany). All measurements were made at 45 ℃.
2.2.4 Flow resistance measurement
The flow behavior of fluid consists of shear flow and elongational flow. Though there is no existence of pure shear flow or elongational flow, the flow of polymer solution in the capillary tube is considered dominant shear flow and the flow in porous medium is the combination of shear flow and elongational flow. Fig.1 shows the instrument for measuring the flow resistance. Fig.1(a) shows capillary tube. In Fig.1(b), as a porous media, the screen structure which consists of five overlapping layers of screen with pore diameter of 75 μm is attached to the bottom of the capillary tube and the
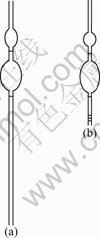
Fig.1 Instruments for measuring flow resistance: (a) Capillry tube; (b) Screen structure
Table 1 Mass fraction and rheological parameters of mixed polyacrylamide

capillary tube is much shorter than that in Fig.1(a). The time for a fixed volume of polymer solution to flow through the capillary tube and screen structure was measured, and it revealed the flow resistance of polymer solutions.
3 Results and discussion
3.1 Viscosity of polyacrylamide solution
The shear viscosity and intrinsic viscosity of each sample are listed in Table 1. The variations in the viscosity and intrinsic viscosity are very small. These values vary by less than 2% from the average values of 28.84, 55.2 respectively. We considered these small variations in shear viscosity and intrinsic viscosity to be negligible, so the mixed polyacrylamide solutions and the solution of P2 without mixing have the same shear viscosity and intrinsic viscosity. According to Ref.[10], the average relative molecular masses of polymers listed in Table 1 are approximately equal.
3.2 Elasticity of polyacrylamide solution
Fig.2 shows a plot of storage modulus of each sample listed in Table 1 as a function of angular frequency, which indicates that the storage modulus of polymer solutions increases with the increase of angular frequency, which reflects the shear rate dependence of elasticity. Fig.2 also shows that the storage moduli of mixed polyacrylamide solutions (M1, M2, M3, M4) are larger than that of P2 solution. It can be concluded that the elasticity of mixed polyacrylamide solutions is higher than that of polyacrylamide solution without mixing only in term of storage modulus, though these polymer solutions have the same shear viscosity and average relative molecular masses.
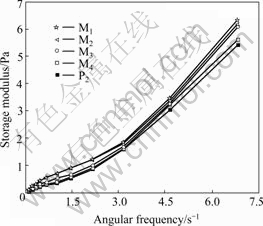
Fig.2 Storage modulus of polyacrylamide solutions vs angular frequency
Another parameter describing the elasticity of polymer solution is polymer filament breakup time. The evolution in the mid-point diameter of filament is plotted vs time in Fig.3. From Fig.3, we can see that filament breakup time of mixed polyacrylamide solutions M3 and M4 is longer than that of P2 solution, and the filament breakup time of mixed polyacrylamide solutions M1 and M2 is shorter than that of P2 solution. However, the surface tensions of mixed polyacrylamide solutions M3 and M4 are larger than those of other polymer solutions. Combining the sequence of breakup time and surface tension, it is obvious that the elasticity of mixed polyacrylamide solutions M3 and M4 is higher than that of polyacrylamide solution without mixing, and the elasticity of M1, M2 is lower than that of the P2 solution.
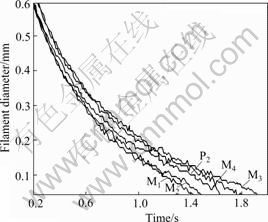
Fig.3 Filament diameter as function of time for polyacrylamide solutions
Storage modulus and filament breakup time describe the elasticity of polymer solution in different aspects. There might be some contradictions between both results such as the conclusions derived from Figs.2 and 3. Despite some disagreements, we can confidently conclude that the elasticity of mixed polyacrylamide solutions M3 and M4 is higher than that of polyacrylamide solution without mixing.
It is well known that the relative molecular mass distribution(RMMD) is the most important factor affecting the elasticity of polymer melt. The mixing of polyacrylamide with different relative molecular masses changes the RMMD of polyacrylamide solution. The results of the above experiment reveal that the RMMD has obvious influence on the elasticity of polyacrylamide solution likewise, while keeping the same viscosity and average relative molecular mass. The feature of mixed polyacrylamide solution with higher elasticity is that the mass fraction of polyacrylamide with in-between molecular mass should be overwhelming majority, and other polyacrylamides should be minority. Thus we can enhance the elasticity of polyacrylamide solution by appropriate mixing of polyacrylamide with different relative molecular masses.
3.3 Flow resistance of polyacrylamide solution
The time of all the polyacrylamide solutions shown in Fig.1 and flowing through the screen structure(ts) and capillary tube(tc) is listed in Table 2. The variations in values of flowing time through capillary tube are very small and these values vary by less than 2% from the average values of 456.528 s. Similar to viscosity, we consider these small variations in flow time to be negligible, so the mixed solutions and P2 have the same resistance flowing through the capillary tube. This result confirms that the flow in capillary tube is viscous-dominated shear flow, and the polymer solutions with the same shear viscosity will hold the same flow resistance.
Table 2 Flow time through screen structure and capillary tube
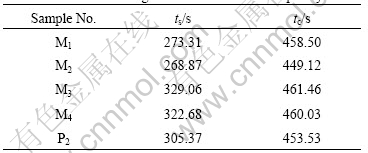
There is evident difference in the flow time of mixed polyacrylamide solutions and P2 solution flowing through screen structure, though these polymer solutions have the same viscosity. Prior work by HASS and DURST[11] confirmed that when polymer solution flows through screen structure, there is a coexistence of shear flow and elongational flow, and the total pressure drop is equal to the pressure caused by shear viscosity plus the pressure drop caused by elasticity of polymer solution. So the polyacrylamide solutions require different time to flow through the screen structure, because they have different elasticities at the same shear viscosity. Mixed polyacrylamides M3 and M4 with high elasticity corresponds to large flow resistance, and M1 and M2 with low elasticity present small flow resistance compared with P2 solution. So it is possible to increase the flow resistance of polyacrylamide solution in porous medium by appropriate mixing of polyacrylamide with different relative molecular masses. This result might have application in enhancing oil recovery by polymer flooding.
4 Conclusions
1) The mixed polyacrylamide solutions prepared have the same shear viscosity and average relative molecular mass. However, some mixed polyacrylamide solutions have higher elasticity than polyacrylamide solution without mixing.
2) The elasticity of polyacrylamide solution can be enhanced by appropriate mixing of polyacrylamide with different relative molecular masses. In the mixed polyacrylamide solution with higher elasticity, the mass fraction of polyacrylamide with in-between relative molecular mass should be overwhelming majority, and the other polyacrylamides should be minority.
3) The higher the elasticity of polyacrylamide solution, the greater the flow resistance flowing through porous medium. This result might have application in enhancing oil recovery by polymer flooding.
References
[1] GRATTONI C A, LUCKHAM P F, JING X D, NORMAN L, ZIMMERMAN R W. Polymers as relative permeability modifiers: Adsorption and the dynamic formation of thick polyacrylamide layers [J]. Journal of Petroleum Science and Engineering, 2004, 45: 233-245.
[2] DONG Man-li, GUO Xiao-li. The viscoelasticity of polymer solution in porous media [J]. Chem Oilfield, 1992(9): 145-150. (in Chinese)
[3] MA Guang-yan. The viscoelasticity of PAM solution in displacing process and distribution of reservoir pressure [J]. Chem Oilfield, 1996(13): 353-356. (in Chinese)
[4] HOU Ji-rui, LIU Zhong-chun, ZHANG Shu-fen. The role of viscoelasticity of alkali/surfactant/polymer solutions in enhanced oil recovery [J]. Journal of Petroleum Science and Engineering, 2005, 47: 219-235.
[5] STELTER M, WUNDERLICH T, RATH S K, BRENN G, YARIN A L, SINGH R P, DURST F. Shear and extensional investigations in solutions of grafted/ungrafted amylopectin and polyacrylamide [J]. Journal of Applied Polymer Science, 1999, 74: 2773-2782.
[6] WUNDERLICH T, STELTER M, TRIPATHY T, NAYAK B R, BRENN G, YARIN A L, SINGH R P. Shear and extensional investigations in solutions of grafted/ungrafted polysaccharides [J]. Journal of Applied Polymer Science, 2000, 77: 3200-3209.
[7] RENARDY M. Some comments on the surface-tension driven breakup of viscoelastic jets [J]. J Non-Newtonian Fluid Mech, 1994, 51: 97-107.
[8] ENTOV V M, HINCH E J. Effect of a spectrum of relaxation times on the capillary thinning of a filament of elastic liquid [J]. J Non-Newtonian Fluid Mech, 1997, 72: 31-53.
[9] KEVIN C TAYLOR, HISHAM A, NASR E D. Water soluble hydrophobically associating polymers for improved oil recovery: A literature review [J]. Journal of Petroleum Science and Engineering, 1998, 19: 265-280.
[10] BOCK J, VALINT P L, PACE S J, SIANO D B, SCHULZ D N, TURNER S R. Hydrophobically associating polymer. STAHL G A, SCHULZ D N, eds. Water-soluble polymers for petroleum recovery [M]. New York: Plenum Press, 1988: 147-160.
[11] HASS R, DURST F. Viscoelastic flow of dilute polymer solutions in regularly packed beds [J]. Rheol Acta, 1982, 21: 566-571.
(Edited by CHEN Wei-ping)
Received date: 2008-06-25; Accepted date: 2008-08-05
Corresponding author: XU Li-na, Chemistry engineer; Tel: +86-459-5903921; E-mail: xulina@petrochina.com.cn