
Droplet transition for plasma-MIG welding on aluminium alloys
BAI Yan(白 岩)1, GAO Hong-ming(高洪明)2, QIU Ling(邱 灵)3
1. Institute for Welding and Surface Technology of R&D Center, Baoshan Iron & Steel Co., Ltd.,
Shanghai 201900, China;
2. State Key Lab of Advanced Welding Production Technology, Harbin Institute of Technology, Harbin 150001, China;
3. Aeronautical Manufacturing Technology Research Institute, Beijing 100024, China
Received 19 November 2009; accepted 13 April 2010
Abstract: The synchronous acquisition system of droplet image inspection and arc electric signals were established and the droplet transition characteristics of aluminum alloys were researched in the plasma-MIG welding process. Typical droplet transition modes include globular transfer mode, short circuiting transfer mode, metastable spray transfer mode and projected transfer mode. The result indicates that MIG droplet transfer frequency and droplet transfer modes are changed by introducing the plasma arc in the plasma-MIG welding process compared with the MIG welding on the aluminum alloys, which broadens the range of welding parameters when the stable welding process proceeds. The metastable spray transfer and projected transfer mode are proved to be the most optimal modes by comparing the stability of electronic signal, droplet transition, weld appearance and weld penetration.
Key words: plasma MIG welding; droplet transition; aluminum alloy; high speed photography
1 Introduction
The plasma-MIG welding process, first invented and studied in Philips Research Laboratories (Holland) in 1972, is regarded as a synthesis of plasma welding and MIG welding, which is superior to regular MIG welding process on the aspects of increasing welding efficiency, reducing welding spatter and gas cavity[1-4]. The characteristic of the process is that the welding wire and MIG arc are surrounded by thermal plasma arc which is propitious to control the transition of droplets and the heat from droplets to base metal[5-9].
Compared with the MIG welding on the aluminum alloys, the preheating effect of welding wire is enhanced by introducing plasma arc in the plasma-MIG welding process and the MIG arc shape is changed by the interaction between the plasma arc and MIG arc, which in turn changes the droplet transfer frequency, droplet transfer types and stability[10-13]. The metal transfer properties of plasma-MIG welding on mild steel were studied by ESSERS et al[14] and LESNEWICH[15], while the research on aluminum alloys were not reported.
In this work, the droplet image inspection system was established and the typical droplet transition modes of plasma-MIG welding process on aluminum alloys were analyzed combined with the arc electric signals. Meanwhile, the influences of plasma-MIG welding parameters on droplet transfer characteristics were researched in order to control the behavior of droplet transfer and obtain the optimum weld effects.
2 Experimental
The signal and image detection system for plasma-MIG welding process shown in Fig.1 included electric signal acquisition module, high speed camera collection module, lighting and filter system. The highest sampling frequency of the high speed camera was 1 000 Hz and the serial port trigger mode was adopted in order to keep synchronization with electric signals sampled under a frequency of 4 kHz.
3 Results and discussion
3.1 Globular transfer
The droplet image and corresponding electric signals are shown in Figs.2 and 3, respectively in the globular transfer of plasma-MIG welding on aluminum alloys. According to the constant melting rate curve for wire with diameter of 1.6 mm, the stable droplet transfer mode should be metastable spray transfer mode or spray transfer mode in the MIG welding process on aluminum alloys when the wire feed rate is 5.7 m/min.
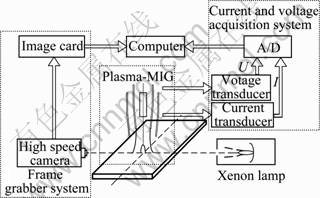
Fig.1 Schematic diagram of signal and image detection for plasma-MIG welding process
From Fig.2, although a small part of current flowed into arc from the position where weld was connected with droplet, most MIG current flowed into the arc through the anode spot under the droplet due to the larger radius, which resulted in that upward spot pressure formed and went against droplet transfer. The gravity of droplet gradually increased and the droplet detached from the wire tip at 1 143 ms. When the droplet fell into the weld pool at 1 167 ms, the MIG voltage increased from 17.6 to 21.3 V and the MIG current decreased from 96.8 to 63.3 A, as shown in Fig.3(a).
The frequency of droplet transfer was low, only 2.8 droplets per second with the welding parameters. The droplet radius calculated according to the wire feed rate and frequency was about 2.5 mm. As seen from Fig.4, a large weld penetration was achieved by the large impact force of detached droplet on the weld pool, although the MIG current was only 95 A. The weld ripples were not smooth due to the larger droplet radius and lower transfer frequency. Short circuiting transfer phenomena often occurred in this mode if the droplet radius was too large.
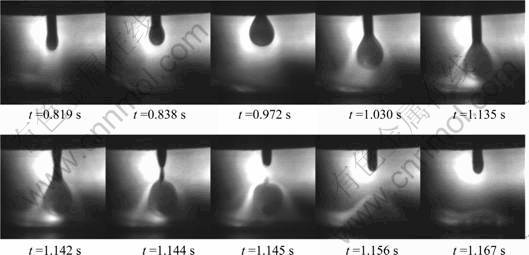
Fig.2 Droplet detachment of plasma-MIG welding with globular transfer mode
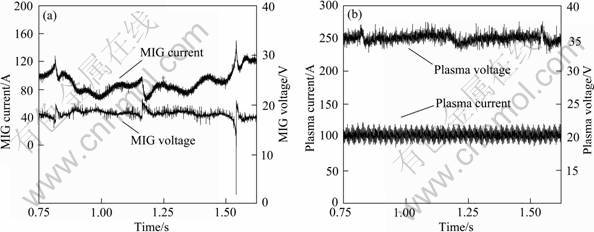
Fig.3 Welding current and voltage in plasma-MIG with globular transfer mode: (a) MIG; (b) Plasma
3.2 Short circuiting transfer
The short circuiting transfer mode could be achieved by increasing wire feed rate and decreasing MIG voltage on the basis of global transfer welding parameters.
As seen from Fig.5, when a droplet was contacted to weld pool, a short circuit and a necking occurred, then the liquid column became thinner gradually under shrinkage force and the current density increased consequently. A droplet detached from the wire tip and the MIG arc was ignited again at 3.547 s. There was no welding spatter during the entire droplet transition. The current and voltage curves are shown in Fig.6. The short circuit duration was about 7 ms, the MIG voltage decreased from 14.1 to 1.6 V and MIG current increased from 95 to 224 A.
The frequency of droplet transfer was only 12.3 droplets per second and the droplet radius calculated according to wire feed rate and frequency was about 1.58 mm. As seen from Fig.7, a small weld penetration was achieved with less arc-heated weld pool heat resulted from short arc time.
3.3 Metastable transfer
The essential differences between metastable spray transfer and short circuit transfer mode were that the necking formed and reached the crisis detaching state before the droplet contacted to the weld pool.
As shown in Fig.8, a necking between wire tip and droplet formed at 6.184 s and a short circuit between droplet and weld pool occurred at 6.186 s. The current and voltage curves are shown in Fig.9. When a droplet contacted to weld pool, the MIG voltage decreased from 15.4 to 2.3 V and MIG current increased from 230.3 to 250.2 A. There was only a slightly change in plasma current and voltage, because the short circuiting duration was only 1 ms, which is short compared with that of short circuiting transfer mode. The frequency of the metastable spray transfer was 55 droplets per second with the welding parameters, and the radius of the droplet can be calculated with the wire-feed speed and frequency. The profile and the crossing section of the weld are shown in Fig.10.
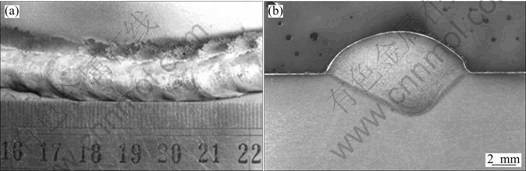
Fig.4 Weld appearance (a) and weld macrograph in cross section (b) with globular transfer mode
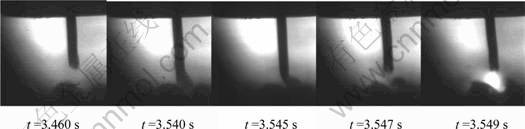
Fig.5 Droplet detachment of plasma-MIG welding with short circuiting transfer mode
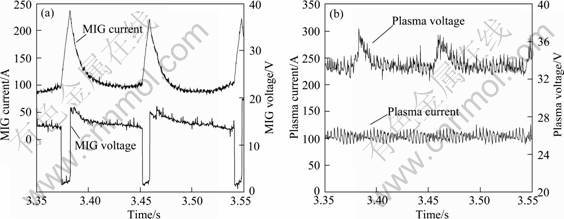
Fig.6 Welding current and voltage in plasma-MIG with short circuiting transfer mode: (a) MIG; (b) Plasma
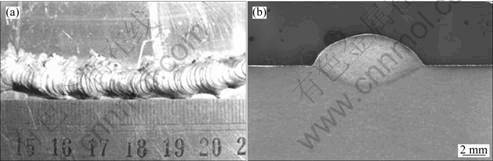
Fig.7 Weld appearance (a) and macrograph in cross section (b) with short circuiting transfer
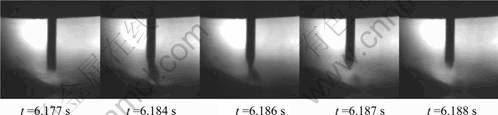
Fig.8 Droplet detachment of plasma-MIG welding with metastable spray transfer mode
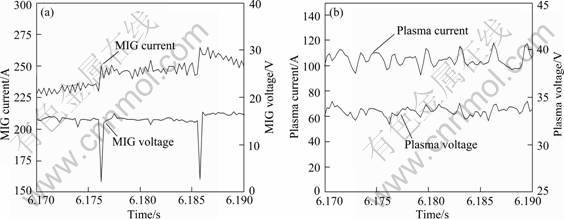
Fig.9 Welding current and voltage in Plasma-MIG with metastable spray transfer mode: (a)MIG; (b) Plasma
3.4 Projected transfer
In the aluminum welding, the shape of the electrode tip was largely affected by the wire-feed speed and the preheating effect of electrode. The larger the preheating effect and the wire-feed speed were, the more easily a “pencil tip” at electrode top formed. It was difficult to form a “pencil tip” in common MIG welding process due to the high thermal conductivity and large viscous of liquid aluminum. Thus, spray transfer mode could not be found in aluminum welding.
The profile of projected transfer in aluminum plasma-MIG welding is shown in Fig.11, and the current and voltage curves are shown in Fig.12. As seen, MIG voltage varied in a range of 2.1 V during the metal transfer process, while MIG current and plasma arc voltage kept a steady state, which indicated that projected transfer was a reliable metal transfer mode. The frequency of projected transfer was 192.6 droplets per second, with a radius of 0.61 mm. Droplet with high frequency and high speed impacted weld pool, and large depth was more easily achieved (Fig.13).
4 Conclusions
1) The globular transfer, short circuiting transfer, metastable spray transfer and projected transfer mode were obtained by adjusting welding parameters.
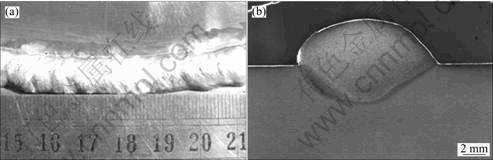
Fig.10 Weld appearance (a) and macrograph in cross section (b) with metastable spray transfer
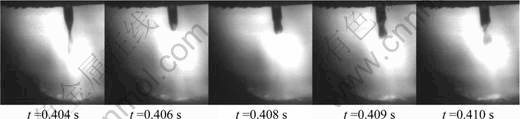
Fig.11 Droplet detachment of plasma-MIG welding with projected transfer mode
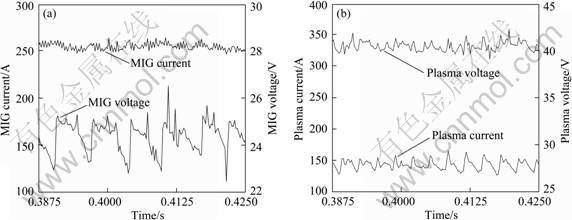
Fig.12 Welding current and voltage in plasma-MIG with projected transfer mode: (a) MIG; (b) Plasma
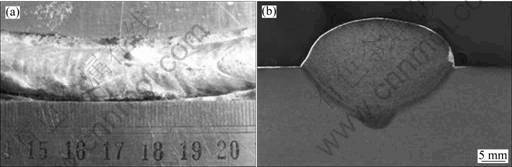
Fig.13 Weld appearance (a) and weld macrograph in cross section (b) with projected transfer mode
2) The metastable spray transfer and projected transfer mode were proved to be the most optimal modes by comparing the stability of electronic signal, droplet transition, weld appearance and weld penetration under the experimental conditions.
3) Compared with traditional MIG welding process, the droplet transfer modes were changed and the frequency became lower with the same MIG current in the plasma-MIG welding process.
References
[1] JELMORINI G, TICHELAAR G W, ESSERS W G, WILLEMS A M, COOPS H. Welding characteristics of the plasma-MIG process [J]. Metal Construction, 1975, 7(11): 568-572.
[2] ESSERS W G. New process combines plasma with GMA welding [J]. Welding Journal, 1976, 55(5): 394-400.
[3] ESSERS W G, JELMORINI G, TICHELAAR G W. The plasma-MIG welding process [J]. Tool and Alloy Steels, 1978, 12(8): 275-277.
[4] TON H. Physical properties of the plasma-MIG welding [J]. Applied Physics, 1975, 8(4): 922-933.
[5] ZHANG Yi-shun, LIU Lei; DONG Xiao-qiang, LI De-yuan. Design and development of plasma-MIG welding equipment [J]. Journal of Shenyang University of Technology, 2007, 29(2): 135-138. (in Chinese)
[6] MATTHES K, KOHLER T. Electrical effects and influencing quantities in the case of the hybrid plasma-MIG welding process [J]. Welding and Cutting, 2002, 54(2): 87-90.
[7] ESSERS W G, WALTER R. Heat transfer and penetration mechanisms with GMA and plasma-GMA welding [J]. Welding Journal, 1981, 60(2): 37-42.
[8] BAI Yan, GAO Hong-ming, WU Lin. State of study on arc characteristic during plasma-MIG welding process [J]. Electric Welding Machine, 2007, 37(9): 17-19. (in Chinese)
[9] PADMANABHAM G, PANDEY S, SCHAPER M. Pulsed gas metal arc welding of Al-Cu-Li alloy [J]. Science and Technology of Welding and Joining, 2005, 10(1): 67-75.
[10] JOSEPH A, FARSON D, HARWIG D. Influence of MIG-P current waveforms on heat input and weld bead shape [J]. Science and Technology of Welding and Joining, 2005, 10(3): 311-318.
[11] OLIVEIRA M A, DUTRA J C. Electrical mode for the plasma-MIG hybrid welding process [J]. Welding and Cutting, 2007, 6(6): 324-328.
[12] BAI Yan, GAO Hong-ming, WU Lin. Plasma-gas metal arc welding procedure on low carbon steel [J]. Transactions of the China Welding Institution, 2006, 27 (9): 59-62. (in Chinese)
[13] BAI Yan. Plasma-MIG arc characteristics and welding process on aluminum alloys [D]. Harbin: School of Materials Science and Engineering, Harbin Institute of Technology, 2007: 68-72. (in Chinese)
[14] ESSERS W G, JELMORINI G, TICHELAAR G W. Arc characteristics and metal transfer with plasma-MIG welding [J]. Metal Construction, 1972, 4(12): 439-447.
[15] LESNEWICH A. Control of melting rate and metal transfer in gas-shielded metal-arc welding [J]. Welding Journal, 1958, 37(9): 418-425.
(Edited by FANG Jing-hua)
Corresponding author: BAI Yan; Tel: +86-21-26649552; E-mail: bai_yan@baosteel.com
DOI: 10.1016/S1003-6326(10)60634-6