J. Cent. South Univ. (2012) 19: 1508-1516
DOI: 10.1007/s11771-012-1169-0
Softening interstage annealing of austenitic stainless steel sheets for stamping processes
HAN Fei(韩飞)1, 2, 3, LIN Gao-yong(林高用)4, HU Meng(胡猛)1,
WANG Shi-peng(王世鹏)1, PENG Da-shu(彭大暑)4, ZHOU Qing(周青)2, 3
1. College of Mechanical and Electrical Engineering, North China University of Technology, Beijing 100144, China;
2. State Key Laboratory of Automotive Safety and Energy (Tsinghua University), Beijing 100084, China,
3. Department of Automotive Engineering, Tsinghua University, Beijing 100084, China;
4. School of Materials Science and Engineering, Central South University, Changsha 410083, China
? Central South University Press and Springer-Verlag Berlin Heidelberg 2012
Abstract: To study the mechanics of work-hardening and annealing-softening, a series of experiments were conducted on samples of 304 austenitic stainless steel sheets. In addition, transmission electron microscopy (TEM), scanning electron microscopy (SEM), and tensile testing were carried out to study changes and mechanisms of the stainless steel structures and properties during work-hardening and annealing-softening. The results indicate that annealing at low temperatures (100-500 ℃) can only remove partial residual stresses in the sample and the softening via annealing is not obvious. Bright annealing and rapid cooling in a protective atmosphere can completely soften the cold-worked material. In addition, the low-temperature sample without a protective atmosphere only has a little oxidation on the surface, but at higher temperature the oxidized layer is very thick. Thus, high-temperature annealing should include bright annealing.
Key words: 304 austenitic stainless steel; work hardening; annealing softening; deformation twinning; strain-induced martensite
1 Introduction
Plates of 304 austenitic stainless steel are commonly used in stamped products. During or after cold-working, stamped products are easily cracked because of significant hardening and high residual stresses [1-4]. This type of cracking is a common problem in production.
During deep-pressing and cold-work hardening, the martensitic phase transformation, which degrades the plastic properties of stainless steel, always occurs. A suitable mid-annealing to promote the transformation of martensite to austenite is an effective way to recover the plastic properties of the work-piece and increase the ratio of good deep-pressed parts to defective parts [5-8]. By studying the AISI 301 austenitic stainless steel, JOHANNSEN et al [9] found that the highest content of reverted austenite was achieved at an annealing temperature of 750 ℃ for a dwelling time of 30 min, and austenite grains with an average grain size of 1.7 μm composed approximately 95% of the specimen microstructure. MISRA et al [10] and RODR?GUEZ et al [11] also verified JOHANNSEN et al’s conclusions. In addition, studies of the hardness and microstructure of cold-rolled SUS301 austenitic stainless steel strips after low-temperature stress-relief annealing by GUI et al [12] and JUNTUNEN et al [6] indicated that the microstructure of the stainless steel strip could be recovered by low-temperature annealing, while the hardness increased slightly with an increase in annealing temperature.
However, researchers now increasingly focus on methods for decreasing the hardness of highly hardened materials [13-17]. TAN and MEI [13] found that the machining property of high Cr-Mn white cast iron was optimal and the hardness was 36-38 HRC after annealing at 850 ℃ for 4 h then at 720 ℃ for 6 h.
To eliminate the residual stresses and soften the material, intermediate annealing must be carried out during forming process [9, 12, 18]. Three main problems should be considered before intermediate annealing being carried out. Firstly, the annealing temperature should exceed 900 ℃ to guarantee thorough annealing. Secondly, annealing temperatures between 500 ℃ and 850 ℃ (sensitized zone) should be avoided, and the cooling rate should be slow enough to avoid the effects of thermal sensitization. Thirdly, delicate instruments and highly purified protective atmosphere are needed to guarantee that no oxidation occurs during annealing.
The 304 austenitic stainless steel plates were used to develop a new type of factory apparatus. The forming process of the shell involved three rounds of deep drawing, trimming, flattening and flanging, etc. During the forming process, the plates always cracked because of serious work-hardening. To develop an annealing process that can be used in practical production, a series of experiments were carried out, and the variable laws and mechanisms of the structure evolution and performance of work-hardened 304 stainless steel after annealing were studied. Then, a suitable intermediate annealing process was developed.
2 Experimental
Optical microscopy, transmission electron microscopy (H-800), microhardness and a tensile test (CSS-44100) were used to study the changes in microstructures and properties and the relating mechanisms of 304 austenitic stainless steels after work-hardening and annealing-softening.
2.1 Materials and sample
In this work, 304 stainless steel plates imported from Japan were used, and the chemical compositions are listed in Table 1. The standard steel tensile specimen is shown in Fig. 1.
Table 1 Chemical compositions of 304 stainless steel (mass fraction, %)
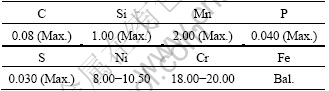
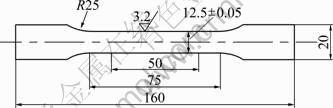
Fig. 1 Standard tensile specimen of 304 stainless steel (Unit: mm)
2.2 Annealing-softening experiment
Semi-finished products and certain pre-deformed specimens provided by manufacturers were annealing-softened via different heat treatments (low temperature and high temperature) by using common heat-treatment furnaces and bright annealing furnaces. The annealing temperature must not be within the sensitization temperature range from 500 ℃ to 850 ℃. The annealing process is shown in Fig. 2. After annealing, the cooling method in common heat-treatment furnace was air-cooling if not stated otherwise. Bright annealing was carried out in a chamber full of hydrogen to prevent surface oxidation of the samples. Bright annealing has two cooling methods: oil cooling and rapid cooling in a water circulation system with hydrogen atmosphere protection (Note: cooling rates in air cooling, oil cooling and rapid cooling in the protective atmosphere are nearly identical. The methods described here were used to compare the surface oxidations of samples). If not otherwise stated, a protective atmosphere was the cooling method used in bright annealing. The processes are shown in Fig. 2. The annealing steps are listed in Table 2. The mechanical properties of the tensile specimen after heat treatment were tested with CSS- 44100-type electronic universal testing machine. The microstructures were analysed with optical microscope, scanning electron microscope (SEM, KYKY-Amray 2800) and transmission electron microscope (TEM, H-800), Vickers hardness measurement was conducted with HAV-10A type Vickers Hardness Tester, and the yield of semi-finished punched products after heat treatment was calculated.
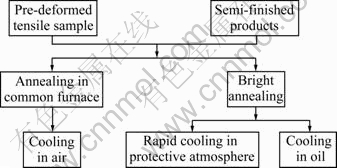
Fig. 2 Annealing steps of pre-deformed parts and semi-finished products
3 Results and discussion
3.1 Effect of annealing on mechanical properties of 304 austenitic stainless steel plates
The effect of different heat treatments on mechanical properties of the 15% pre-deformed 304 stainless steel sample is given in Table 3.
The elongation of the 15% pre-deformed samples without heat treatment is the worst. Low-temperature annealing has less effect on the yield strength of the sample, i.e., the yield strength for an annealing temperature of 330 ℃ is almost unchanged. However, high-temperature annealing has a large effect on the yield strength of the sample. The yield strength rapidly decreases to 257.9 MPa when the annealing temperature is 1 050 ℃ and the heating time is 3 min. The tensile strength σb decreases as the annealing temperature increases, but the magnitude of the change is much less than that of the yield strength. The Vickers hardness (HV) of the samples decreases as the annealing temperature increases. However, the hardness changes little after low-temperature annealing, while the elongation of the sample increases significantly as the annealing temperature increases. In particular, for high-temperature (1 050 ℃) annealing, the elongation increases from the initial 44% up to 73%.
Table 2 Annealing steps of pre-deformed 304 stainless steel and semi-finished products
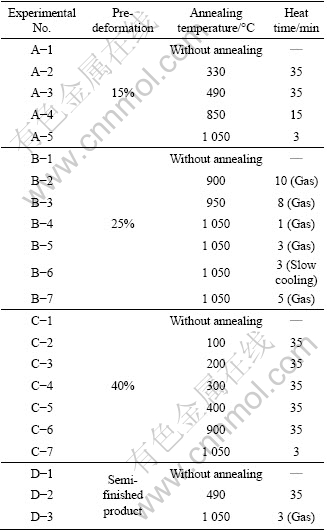
The effect of different heat treatments on the mechanical properties of the 25% pre-deformed 304 stainless steel sample is given in Table 4.
The formability of the 25% pre-deformed sample after high-temperature annealing increases significantly. The elongations of the samples that are rapidly cooled in the inert atmosphere after annealing at 1 050 ℃ for 3 min are the best, while at the same temperature but heated for 5 min, the softening effect is almost unchanged. However, if the sample is slowly cooled in the furnace after annealing at 1 050 ℃ for 3 min, although the hardness is almost unchanged, the elongation is decreased to 67.5% from 80% (1 050 ℃ for 3 min). This decrease in elongation is due to the carbide precipitated from the grain boundaries during cooling and thus the plastic properties of the material suffer.
The effect of different heat treatments on the mechanical properties of the 40% pre-deformed 304 stainless steel sample is given in Table 5.
After low-temperature annealing, the mechanical properties of the 40% pre-deformed sample are almost unchanged compared to the sample without pre-treatment deformation. The elongations of the samples slightly decrease instead when cooled in air for 35 min at 300 ℃ or 400 ℃, and the high degree of work-hardening remains. However, when the annealing temperature is increased to 900 ℃, the formability of the samples is greatly improved, and the sample annealed at 1 050 ℃ for 3 min is fully softened after cooling in air, which yields the best results.
Table 3 Mechanical properties of 15% pre-deformed sample after annealing
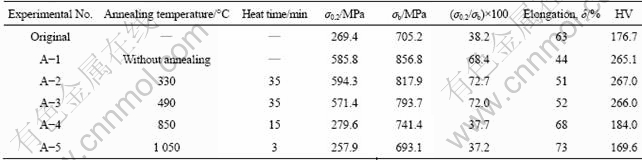
Table 4 Mechanical properties of 25% pre-deformed sample after annealing
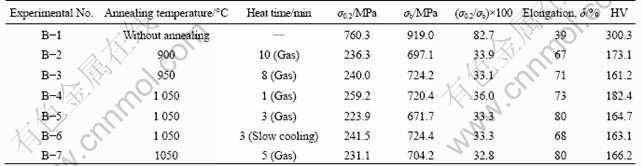
Table 5 Mechanical properties of 40% pre-deformed sample after annealing
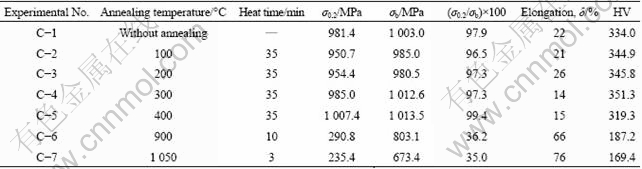
The above results demonstrate that: 1) The material is severely hardened after cold-working; 2) When the materials have pre-deformation, the mechanical properties remain, and the formability is not improved by annealing at low temperature; 3) High-temperature annealing plays an important role in changing the properties, and the material can be fully softened, especially after rapid cooling from 1 050 ℃, which can yield the best results; 4) High-temperature annealing should be used with rapid cooling method to ensure optimal mechanical properties, rather than slow cooling which results in sub-optimal properties.
3.2 Effect of annealing on microstructure of 304 austenite stainless steel
Microstructure morphologies of the 15% pre- deformed tensile parts after heat treatment are shown in Fig. 3 and Fig. 4.
Figure 3(a) and Figure 4(a) show the microstructure morphologies of the sample that is annealed at low temperature and cooled in air after heating at 490 ℃ for 35 min. The images show that the structures undergo little change and the microstructure from the cold- working remains. Figure 4(a) shows that there are a large number of deformation twins and lath-shaped martensite near the grain boundaries in the matrix. Figure 4(b) shows that two groups of deformation twins are intercrossing. Figure 3(b) and Figure 4(c) show the microstructure morphologies of the samples after solid-melt heat treatment at 1 050 ℃ for 3 min with air- cooling. Figure 3(b) shows the fully re-crystallized material with carbide dispersed uniformly in grains, and annealing twins formed at the grain boundaries during grain growth. Figure 4(c) shows that there are only a few
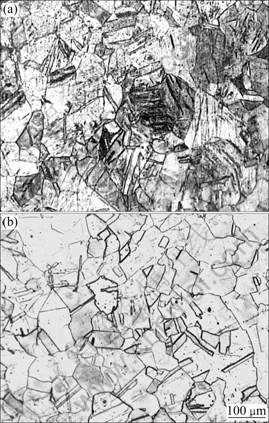
Fig. 3 Metallographic microstructures of 15% pre-deformed tensile parts after heat treatment: (a) 490 ℃, 35 min; (b) 1 050 ℃, 3 min
dislocations and stacking faults in the matrix after annealing.
The microstructures of the 25% pre-deformed tensile pieces heat-treated in different ways are shown in Fig. 5 and Fig. 6. After air-cooled annealing at 900 ℃ for 10 min, because the annealing temperature is low, carbides in the grain boundary are not fully integrated into the matrix (as shown in Fig. 5(a)), and there are a large number of dislocations (as shown in Fig. 6(a)). After rapid cooling in inert gases following bright annealing at 1 050 ℃ for 3 min, the grains are completely recrystallized, and the carbides are almost completely dissolved and diffused evenly in the grains. Moreover, the size of the grains is uniform (as shown in Fig. 5(b)). Figure 6(b) clearly shows the matrix. There are some disappearing stacking faults and a few dislocations. When the dwell time for bright annealing is 5 min, the size of different grains increases significantly, part of which grows abnormally and undergoes secondary recrystallization (as shown in Fig. 5(c)). There are a few vanishing stacking faults in the matrix (as shown in Fig. 6(c)). Figure 5(d) and Figure 6(d) show that after annealing at 1 050 ℃ for 3 min and slow cooling, the dissolved carbide in the matrix precipitates again at the grain boundaries. Because of slow cooling through the sensitization temperature region (500- 850 ℃), sensitization occurs in a short time, that is, carbides (Cr23C6) discontinuously precipitate along the grain boundaries and do not have the required chromium content for corrosion resistance. Thus, the material is highly vulnerable to electrochemical corrosion, and the bonding between grains of 304 stainless steel is seriously compromised. There are also a few dislocations and stacking faults in the microstructure after this annealing process. The selected area electron diffraction pattern and diffraction pattern analysis show that the matrix is cubic lattice and the phase is austenite (as shown in Figs. 6(e) and (f)).
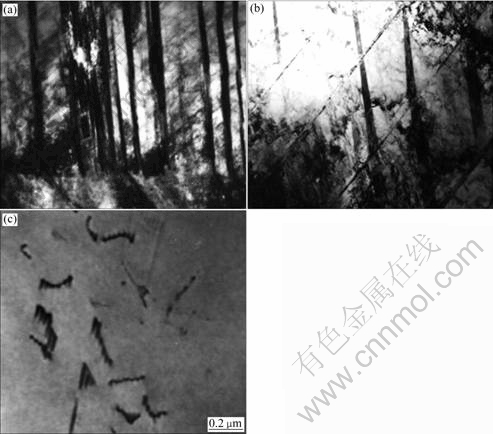
Fig. 4 Microstructure morphologies of 15% pre-deformed tensile parts after heat treatment: (a) 490 ℃, 35 min; (b) 490 ℃, 35 min; (c) 1 050 ℃, 3 min
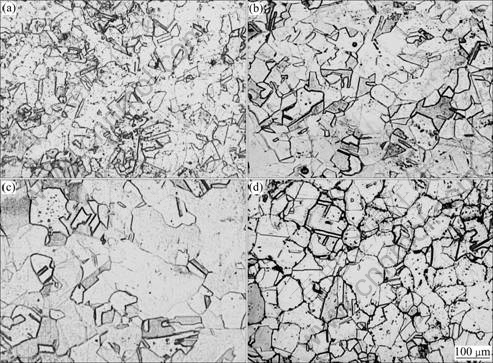
Fig. 5 Metallographic structures of 25% pre-deformed tensile pieces heat-treated in different ways: (a) 900 ℃, 10 min; (b) 1 050 ℃, 3 min in gas; (c) 1 050 ℃, 5 min in gas; (d) 1 050 ℃, 3 min slow cooling
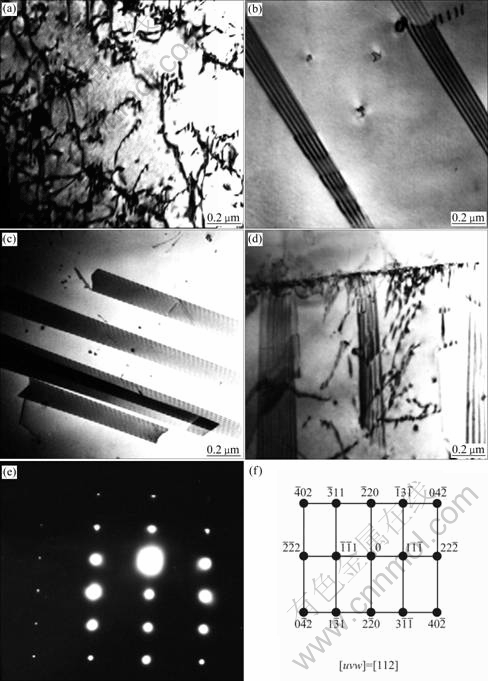
Fig. 6 TEM microstructure morphologies of 15% pre-deformed tensile parts after high heat-temperature treatment: (a) 900 ℃, 10 min; (b) 1 050 ℃, 3 min in gas; (c) 1 050 ℃, 5 min in gas; (d) 1 050 ℃, 3 min slow cooling; (e) Selected area electron diffraction pattern; (f) Diffraction pattern analysis
The microstructure morphologies of the 40% pre-deformed tensile sample, observed by optical microscope, are shown in Fig. 7. When being cooled in air after heating for 35 min at 400 ℃, the microstructure has little change, and there are still many deformation twins in the matrix (as shown in Fig. 7(a)). After air- cooled annealing at 1 050 ℃ for 3 min, complete recrystallization occurs. The carbides are evenly distributed in the matrix, and annealing twins form at the grain boundaries after grain growth (as shown in Fig. 7(b)).
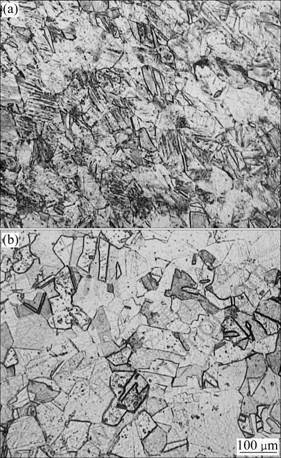
Fig. 7 Metallographic microstructures of 40% pre-deformed tensile sample after different re-annealing processes: (a) 400 ℃, 35 min; (b) 1 050 ℃, 3 min
3.3 Effects of microstructure of 304 austenitic stainless steel on mechanical properties
Annealing-softening of pre-deformed tensile samples as related to the performance of materials is mainly performed to eliminate the internal stresses and reduce the hardness, thereby increasing the forming performance of plates. The microstructure after cold-working exhibits a large number of deformation twins, lath martensite and a high density of dislocations. After annealing at low temperature, the microstructure is almost unchanged, and there are still a large number of twins, dislocations and strain-induced martensite. The mechanical properties also exhibit little change. After bright annealing at 1 050 ℃ for 3 min and rapid cooling in the protective gas, defects in the microstructure almost disappear, and the material is completely softened. The mechanical properties return to their original state, as shown in Table 3. After bright annealing at 1 050 ℃ for 5 min and rapid cooling in the protective gas, the alloy undergoes secondary recrystallization, and a small number of grains grow abnormally. The mechanical properties almost return to their original state, while the difference in grain size increases greatly, which is detrimental to punch forming. If the temperature is not high enough (950 ℃ for 8 min), dislocations in matrix do not entirely disappear, and the mechanical properties are worsened compared to the above cases. However, upon slow cooling from high temperature, carbides (Cr23C6) discontinuously precipitate along the grain boundaries, but the material properties suffer, and the forming performance is reduced.
The experimental data show that lower temperatures (850-1 000 ℃) and longer times (10-15 min) also can soften the material to a greater degree. However, using a short time (3 min) and intermediate temperature (1 050 ℃) annealing process will improve the production efficiency because plate metal punching is a high-speed process. To avoid material sensitization, the cooling rate after annealing must reach 180 ℃/min or greater, and the part temperature must decrease to below 500 ℃ within 3 min. To avoid surface discoloration caused by oxidation and to minimize subsequent cleaning steps, a high temperature intermediate annealing should be carried out in a protective atmosphere in a specially designed furnace for continuous production.
The above results all show that bright annealing at 1 050 ℃ for 3 min and rapid cooling in a protective atmosphere can completely soften the material, and thus the microstructure and formability can return to their original states, which is a better annealing process.
3.4 Effects of annealing on yield of 304 austenitic stainless steel punched products
Before suitable mid-annealing is incorporated, products produced from 304 austenitic stainless steel plates are full of forming defects. A lagging crack defect is shown in Fig. 8(a).
Annealing-softening experiments on semi-finished products, which have already undergone the first deep drawing (the largest deformation during processing), are conducted in two ways. The experimental results for the pre-deformed tensile parts with simulated punching deformation are given in Table 6.
For semi-finished products without intermediate annealing-softening, the semi-final yield (20%) is very low. However, after low-temperature annealing, local stress concentration is eliminated, which can increase the yield to 50%, but the piece is not completely softened. After bright annealing at 1 050 ℃ for 3 min followed by rapid cooling in a protective atmosphere, the yield can reach 97% (the damaged product is due to mold strain, not material hardening), which shows that the material is completely softened. The yield meets the requirements of the customer. The punched products that are rapidly cooled under a protective atmosphere after high- temperature (1 050 ℃) and short time (3 min) bright annealing are shown in Fig. 8(b).
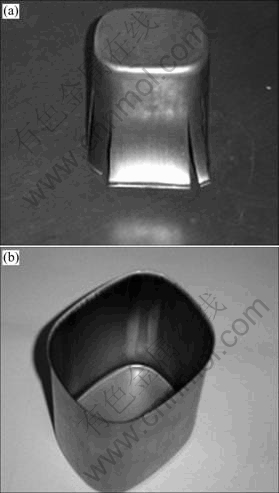
Fig. 8 Punched products: (a) Punched product with lagging crack defect; (b) Heat-treated punched products
Table 6 Effects on yield of semi-finished products on first deep drawing process
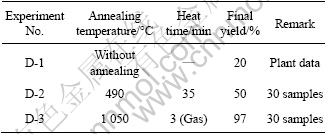
4 Conclusions
1) After annealing at low temperature (100-500 ℃), the microstructure of the cold-deformed sample has almost no change, and there are still a large number of twins, dislocations and strain-induced martensite. The mechanical properties have little change. Annealing at low temperature can only remove partial residual stress of the sample, and the annealing-softening is not obvious.
2) Bright annealing at 1 050 ℃ for 3 min and rapid cooling in a protective atmosphere (cooling rate> 180 ℃/min) can completely soften the materials of the cold-deformed sample, and the microstructure and formability can return to their original states. The yield of punched semi-finished products after annealing can reach 97%.
3) The sample surface has a little oxidation after annealing at low temperature without a protective atmosphere (200-490 ℃). The sample surface has very thick oxidation layer that is distributed unevenly after annealing at high temperature. So, high-temperature annealing must use bright annealing.
References
[1] BALAN K P, REDDY A V, SARMA D S. Effect of single and double austenitization treatments on the microstructure and mechanical properties of 16Cr-2Ni steel [J]. Journal of Materials Engineering and Performance, 1999, 8(3): 385-393.
[2] RAVI K B, MAHATO B, SINGH R. Influence of cold-worked structure on electrochemical properties of austenitic stainless steels [J]. Metallurgical and Materials Transactions A, 2007, 38(9): 2085- 2094.
[3] ROBINSON S L, ODEGARD B C, MOODY N R, GOOD S H, CHIESA M, MEYER B A. Strain-aging in highly worked 316L stainless steel [J]. Practical Failure Analysis, 2001, 1(2): 83-92.
[4] de MOOR E, LACROIX S, CLARKE A J, DENNING J, SPEER J G. Effect of retained austenite stabilized via quench and partitioning on the strain hardening of martensitic steels [J]. Metallurgical and Materials Transactions A, 2008, 39(11): 2586-2595.
[5] M?ESZ?AROSA I, PROH?ASZKAB J. Magnetic investigation of the effect of α?-martensite on the properties of austenitic stainless steel [J]. Journal of Materials Processing Technology, 2005, 161: 162- 168.
[6] JUNTUNEN P, KARJALAINEN P, RAABE D, BOLLE G, KOPIO T. Optimizing continuous annealing of interstitial-free steels for improving deep drawability [J]. Metallurgical and Materials Transactions A, 2001, 32(8): 1989-1995.
[7] KHLESTOV V M, McQUEEN H J, CINGARA G A, KONOPLEVA E V. Characteristics of steels in annealing combined with hot-rolling preheating [J]. Journal of Materials Engineering and Performance, 2005, 14(2): 179-187.
[8] SUH D W, OH C S, KIM S J. Dilatometric analysis of austenite formation during intercritical annealing [J]. Metals and Materials International, 2008, 14(3): 275-282.
[9] JOHANNSEN D L, KYROLAINEN A, FERREIRA P J. Influence of annealing treatment on the formation of nano/submicron grain size AISI 301 austenitic stainless steels [J]. Metallurgical and Materials Transactions A, 2006, 37(8): 2325-2338.
[10] MISRA R D K, NAYAK S, MALI S A, SHAN S J, SOMANI M C, KARJALAINEN L P. Microstructure and deformation behavior of phase-reversion-induced nanograined/ultrafine-grained austenitic stainless steel [J]. Metallurgical and Materials Transactions A, 2009, 40(10): 2498-2509.
[11] RODRI?GUEZ-BARACALDO R, BENITO J A, CABRERA J M. Tensile and compressive test in nanocrystalline and ultrafine carbon steel [J]. Journal of Materials Science, 2010, 45: 4796-4804.
[12] GUI Jing-jing, MING Rui-zhen, LONG Yuan-ning, TU Yi-you. Effect of low-temperature annealing on hardness and microstructure of cold rolled austenitic stainless steel strip [J]. Heat Treatment of Metals, 2010, 35(8): 15-18. (in Chinese)
[13] TAN Yin-yuan, MEI Li-ping. Soften treatment of high Cr-Mn white cast iron [J]. Heat Treatment of Metals, 2002, 27(1): 21-22. (in Chinese)
[14] JENA P K, MISHRA B, RAMESH BABU M, BABU A, SINGH A K, SIVAKUMAR K, BALAKRISHNA B T. Effect of heat treatment on mechanical and ballistic properties of a high strength armour steel [J]. International Journal of Impact Engineering, 2010, 37: 242-249.
[15] CLEMONS K, LORRAINE C, SALGADO G, TALOR A, OGREN J, UMIN P, ES-SAID O S. Effects of heat treatments on steels for bearing applications [J]. Journal of Materials Engineering and Performance, 2007, 16(5): 592-596.
[16] BEREZOVSKAYA V V, KOSTINA M V, BLINOVl E V, BOBRDVA V E, BANNYKH I O. Effect of heat treatment on the structure of high-nitrogen austenitic corrosion-resistant 04Kh22AG17N8M2F and 07Kh20AG9N8MF steels [J]. Russian Metallurgy (Metally), 2009, 2009(2): 146-153.
[17] LEE S J, CLARKE K D, VAN TYNE C J. An on-heating dilation conversional model for austenite formation in hypoeutectoid steels [J]. Metallurgical and Materials Transactions A, 2010, 41(9): 2224- 2235.
[18] TARASENKO L V, TITOV V I, ELYUTINA L A. Control of variation of properties of maraging chromium-nickel steels in long-term heating [J]. Metal Science and Heat Treatment, 2010, 52(5/6): 251-254.
(Edited by HE Yun-bin)
Foundation item: Project(2009D005002000003) supported by the Foundation for Fostering Outstanding Talents of Beijing, China
Received date: 2011-07-04; Accepted date: 2012-02-08
Corresponding author: HAN Fei, Associate Professor, PhD; Tel: +86-10-88803702; E-mail: hanfei@ncut.edu.cn