J. Cent. South Univ. (2017) 24: 1544-1550
DOI: 10.1007/s11771-017-3559-9

Kinetics of roasting potash feldspar in presence of sodium carbonate
LIU Jia-nan(刘佳囡)1, ZHAI Yu-chun(翟玉春)1, 2, WU Yan(吴艳)1, ZHANG Jun(张俊)1, SHEN Xiao-yi(申晓毅)1
1. School of Metallurgy, Northeastern University, Shenyang 110819, China;
2. School of Resources and Materials, Northeastern University at Qinhuangdao, Qinhuangdao 066004, China
Central South University Press and Springer-Verlag Berlin Heidelberg 2017
Abstract: A novel process was proposed for the utilization of potash feldspar by roasting in the presence of sodium carbonate. The effects of roasting temperature, granularity, molar ratio of sodium carbonate to potash feldspar and roasting time on the silica extraction rate were investigated. Under the optimal roasting conditions, the silica extraction rate was 98%. The optimal conditions, determined using an orthogonal experiment, were found to be roasting temperature of 875 °C, potash feldspar granularity of 74–89 μm, molar ratio of sodium carbonate to potash feldspar of 1.2:1, and roasting time of 80 min. The kinetics of potash feldspar roasting in the presence of sodium carbonate was described by the shrinking core model and the reaction rate was found to be controlled by the chemical reaction at the particle surface. According to the Arrhenius expression, the activation energy was 164.99 kJ/mol, and the process could be expressed as [1-(1-α)1/3]=2.66×105 exp[-164990/(RT)] t.
Key words: potash feldspar; sodium carbonate; roasting kinetics; silica
1 Introduction
Potash feldspar is an insoluble aluminum silicate mineral composed of an alkali or alkaline earth metal such as potassium, sodium, calcium, or barium that is commonly found in China. Due to its chemical composition, potash feldspar is extremely stable [1-9].
To date, a variety of methods that aim at the effective utilization of potash feldspar has been reported [10-13]; including ion exchange methods and methods that damage the silicon aluminum oxygen lattice. Reported ion exchange methods include molten salt ion exchange, hydrothermal treatment, and high-temperature volatilization. Methods designed to damage the silicon aluminum oxygen lattice include low-temperature decomposition, high-pressure hydration, and sintering. In general, each of these methods has one or more drawbacks. The process of molten salt ion exchange results in the formation of residues without any industrial value [14, 15], whereas hydrothermal treatment [16-20] requires liquid in abundance and a high energy input to facilitate the preparation of potassium products by evaporation. High-temperature volatilization requires a high energy input, delivers a low economic benefit, and requires the difficult recycling of potassium entrained in the process gas. Low-temperature decomposition results in the emission of large amounts of hydrogen fluoride and ammonia, which causes equipment corrosion and environmental pollution. High-pressure hydration has some shortcomings, including a complex process and unique infrastructure requirements. Sintering [21-24] at medium temperatures in the presence of a co-solvent such as sodium carbonate or calcium carbonate is an effective method for the decomposition of potash feldspar, which meets environmental targets for pollution reduction and energy conservation.
In this work, the kinetics of potash feldspar roasting was studied in the presence of sodium carbonate. The reaction mechanism was analyzed; the kinetic model and the rate-controlling step of the reaction were determined in order to find a way to improve the reaction rate and further boost the extraction rate of the reaction.
2 Experimental
2.1 Materials
The raw material used in this work was obtained from Liaoning Province, China. The chemical composition of the potash feldspar was analyzed using inductively coupled plasma-optical emission spectrometry (ICP-OES); the results are shown in Table 1. The mineralogical composition of the sample was investigated using X-ray diffraction (XRD). The XRD analysis was performed using Cu Kα radiation (λ=1.5406 nm) at 40 kV and 30 mA. The results, shown in Fig. 1 indicate that microcline and albite are the major mineral phases, and dissociative silica a minor phase. Furthermore, silicon is present as microcline, albite and dissociative silica.
Table 1 Chemical composition of potash feldspar (mass fraction, %)

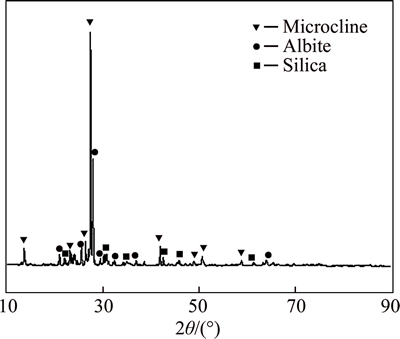
Fig. 1 XRD pattern of potash feldspar
Sodium carbonate was industrial grade. Other chemical reagents were analytical grade. Deionized water was used throughout the experiments.
2.2 Method
Potash feldspar was crushed, ground and dry-sieved to different granularities. Sodium carbonate, the granularity of which was less than 75 μm, was mixed with potash feldspar at the desired molar ratio. The mixture was put into a resistance wire-heating furnace that was controlled by a programmable temperature controller with a deviation of ±1 °C, and heated. When the selected time intervals were reached, the clinker was taken out and cooled to room temperature in order to stop the reaction. After being crushed, ground, and dry-sieved to a granularity less than 75 μm, the clinker was leached into 0.2 mol/L sodium hydroxide solution at 95 °C for 80 min using a mechanical stirrer at 400 r/min. The silica content in solution was determined using sodium fluoride titration and the extraction rate was calculated using Eq. (1).
(1)
where αSiO2 is the silica extraction rate,
is the extracted mass of silica in the solution, and mSiO2 is the total mass of silica in the potash feldspar.
3 Results and discussion
3.1 Thermodynamic analysis
Figure 2 shows the thermogravimetry–differential thermal analysis (TG-DTA) curves of a mixture in which the molar ratio of sodium carbonate to potash feldspar was 1.1:1. The TG curve shows an obvious decrease in the sample mass at roasting temperatures between 788.64 and 875.26 °C. The DTA curve exhibits a weak peak at 78.89 °C, which may be attributed to the loss of absorbed water. When the roasting temperature reaches the range 788–875 °C, the reaction system undergoes a phase change from solid–solid to liquid– solid and CO2 is eliminated, which leads to a strong peak in the DTA curve at 820.15 °C.
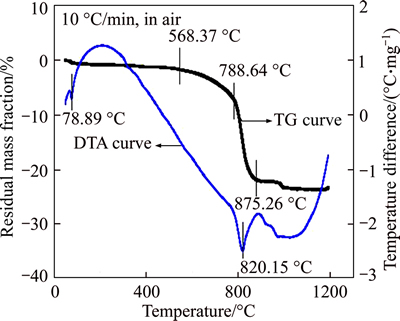
Fig. 2 TG–DTA curves of potash feldspar roasted in presence of sodium carbonate
To better understand the phase transformation of the mixture, the clinkers obtained at different roasting temperatures were analyzed using X-ray diffraction. The results presented in Fig. 3 indicate that the expected products sodium silicate, kaliophilite and nepheline are generated when the roasting temperature reaches 800 °C.
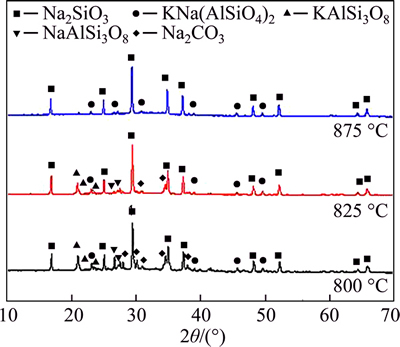
Fig. 3 XRD patterns of clinker obtained at different roasting temperatures
In contrast, if the roasting temperature does not reach the minimum eutectic point, the reaction is not complete, and part of the sodium carbonate remains in the clinker. As the roasting temperature increases, the liquid phase starts to appear in the system, and the reaction mixture transforms from the solid–solid phase to the liquid–solid phase. Consequently, the surface area available for interaction between the reagents increases and the reaction conditions are thus improved. When the roasting temperature reaches 875 °C, the diffraction absorption peaks corresponding to microcline and albite disappear. When the reaction reaches completion, sodium silicate, kaliophilite and nepheline are only the products present in the system.
3.2 Roasting procedure
The main reactions that occur during the roasting of potash feldspar in the presence of sodium carbonate are simply described as follows:
KAlSi3O8+2Na2CO3=KAlSiO4+2Na2SiO3+2CO2↑ (2)
NaAlSi3O8+2Na2CO3=NaAlSiO4+2Na2SiO3+2CO2↑ (3)
SiO2+Na2CO3=Na2SiO3+CO2↑ (4)
The silica extraction rate is mainly affected by roasting temperature, molar ratio of sodium carbonate to potash feldspar, roasting time and granularity. These four key parameters interact and can simultaneously alter the silica extraction rate. The appropriate parameters for use in a single factor experiment are therefore optimized using an orthogonal experiment to determine the optimum procedure for potash feldspar roasting with sodium carbonate. The results and analysis of the orthogonal experiments are listed in Table 2.
The range method is used in the statistical analysis of the orthogonal experiment results. The results are concluded according to R (extreme difference): 1) The relationship between the primary and secondary of each factor affecting the silica extraction rate is roasting temperature>granularity>molar ratio of Na2CO3 to ore> roasting time, namely the roasting temperature is the predominant factor that affects the silica extraction rate. Granularity is more important than the molar ratio of sodium carbonate to potash feldspar, while roasting time has a marginal effect on the extraction rate; 2) Multiple validation experiments are conducted according to the optimized roasting conditions. Under these conditions, viz., roasting temperature 875 °C, granularity 74-89 μm, molar ratio of sodium carbonate to potash feldspar 1.2:1, and roasting time 80 min, the silica extraction rate is stable at 98%.
The chemical composition of the roasted residue obtained under the optimized roasting conditions is examined using ICP-OES, and the results are listed in Table 3. Compared with Table 1, the silica content decreases while the content of aluminum oxide, sodium oxide, and potassium oxide increase. It can therefore be concluded that most of the silica is extracted under the optimized conditions. The X-ray diffraction pattern of the roasted residue shown in Fig. 4 indicates that the main phases of the roasted residue are kaliophilite and nepheline. The diffraction peaks are sharp, indicating that the crystalline structures of microcline, low albite, and quartz in potash feldspar are no longer present. Figure 5 shows the scanning electron microscopy (SEM) images of potash feldspar and the roasted residue. The potash feldspar particles are spherical and distributed homogeneously across a narrow size range. When compared with potash feldspar, it is apparent that the roasted residue particles are not uniform or porous and that their surface is rough as a result of the corrosion by sodium carbonate. Furthermore, the roasted residue particles are far smaller than those of potash feldspar.
Table 2 Results and analysis of orthogonal experiments
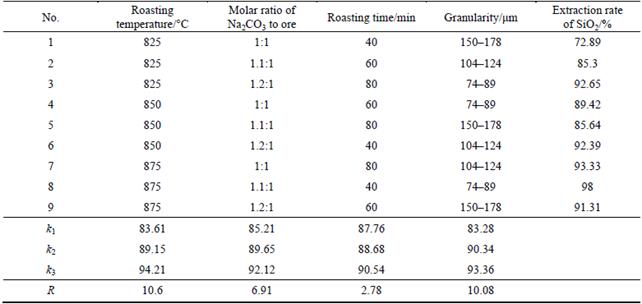
Table 3 Main chemical composition of roasted residue (mass fraction, %)

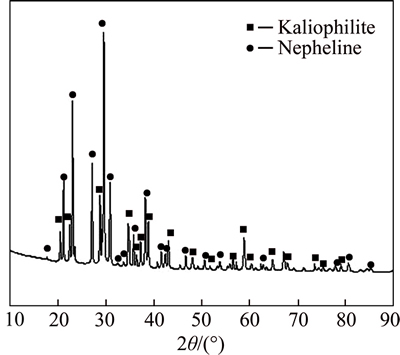
Fig. 4 XRD pattern of roasted residue
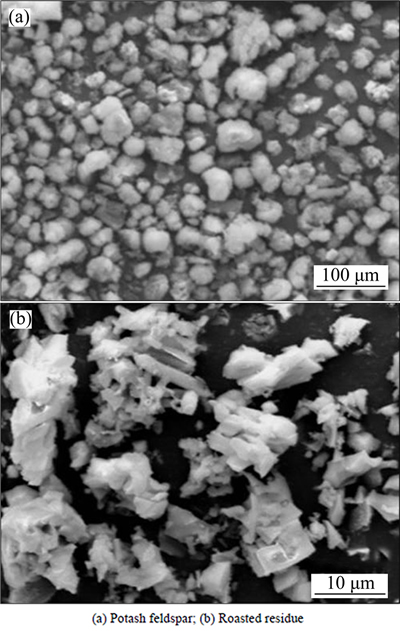
Fig. 5 SEM images
3.3 Kinetics analysis
The roasting of potash feldspar in the presence of sodium carbonate is a typical liquid–solid reaction, as the liquid phase appears in the reaction system when the roasting temperature reaches the minimum eutectic point. Potash feldspar particles are spherical and uniform in shape while also dense without holes, as shown in Fig. 5(a). After reacting with sodium carbonate, the particles of the remaining inert substance, kaliophilite and nepheline, are loose, as shown in Fig. 5(b). Therefore, the particle model can be analyzed with the shrinking core model.
The roasting process can be described in five steps: 1) the molten sodium carbonate externally diffuses through the diffusion layer to reach the potash feldspar particle surface; 2) the molten sodium carbonate diffuses further through the product layer as a form of internal diffusion; 3) the chemical reaction occurs at the particle surface between molten sodium carbonate and solid potash feldspar; 4) the insoluble products, kaliophilite and nepheline, form an increasing product layer around the potash feldspar particle while the soluble product, sodium silicate, diffuses through the product layer; 5) the sodium silicate then diffuses through the diffusion layer into the system. The overall reaction rate is determined by the slowest step in the roasting process.
When the external diffusion through the diffusion layer is the rate-controlling step, the kinetic equation can be expressed as follows:
α=kdt (5)
When internal diffusion through the product layer is the rate-controlling step, the following expression can be used to describe the roasting kinetics:
1+2(1-α)-3(1-α)2/3=kdt (6)
When the chemical reaction between molten sodium carbonate and potash feldspar at the particle surface is the rate-controlling step, the following expression can be used to describe the kinetics of the roasting process:
1-(1-α)1/3=krt (7)
In the equations above, α is the silica extraction rate, kr and kd are the apparent rate constants of reaction and diffusion, respectively, and t is the reaction time.
3.3.1 Effect of roasting temperature
The effects of roasting temperature and roasting time on the silica extraction rate were investigated using potash feldspar particles with a granularity of 74-89 μm and a molar ratio of sodium carbonate to potash feldspar of 1.2:1 by roasting at temperatures of 800, 825, 850 and 875 °C. The results presented in Fig. 6 show that roasting temperature has a noticeable effect on the silica extraction rate, which increases as the roasting temperature increases. When the roasting temperature increases from 800 to 875 °C, the silica extraction rate improves from 52.48% to 97.97% following a roasting time of 80 min. It seems that the roasting process may be controlled by the chemical reaction at the surface, since it is well known that the rate of chemical reactions at surfaces is strongly dependent on roasting temperature, whereas the effect of temperature on external or internal diffusion-controlled processes is slight.
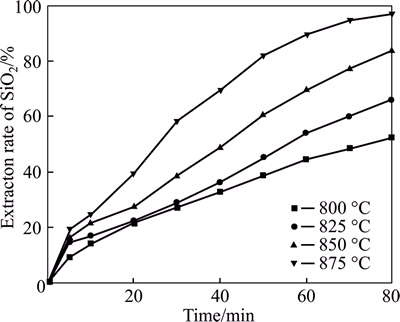
Fig. 6 Effects of roasting temperature and roasting time on silica extraction rate
From the experimental data in Fig. 6, it can be seen that the roasting process is not controlled by external diffusion through the diffusion layer, as the data are not consistent with a straight line passing through the origin. Using the silica extraction rates at different roasting temperatures shown in Fig. 6 together with Eq. (6), the values of [1+2(1-α)-3(1-α)2/3] are calculated and plotted against the roasting time. Table 4 shows the apparent reaction rate constants and their correlation coefficients for diffusion through the product layer. The results indicate that internal diffusion is not the rate-controlling step of the reaction.
Table 4 Apparent reaction rate constants and correlation coefficients calculated at different roasting temperatures by assuming reaction was controlled by internal diffusion
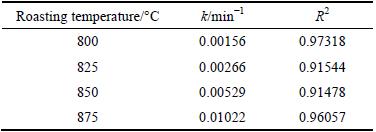
The data are then substituted into Eq. (7). The relationship between [1-(1-α)1/3] and the roasting time, presented in Fig. 7, clearly shows that the rate of the roasting process is determined by the chemical reaction at the surface.
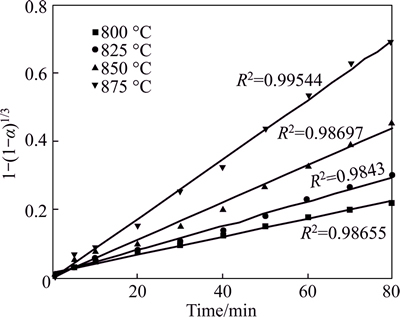
Fig. 7 Relationship between [1-(1-a)1/3] and t at different reaction temperatures
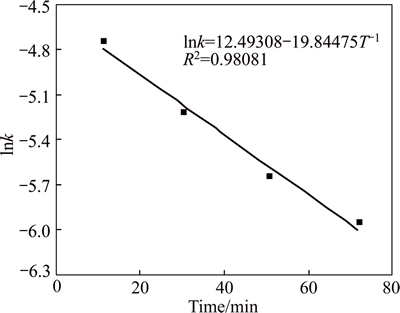
Fig. 8 Relationship between ln k and T-1
The apparent reaction rate constants are determined from the straight lines shown in Fig. 7 and the relationship between lnk and T-1 is determined, as shown in Fig. 8. According to the Arrhenius equation, the apparent activation energy of the roasting process is 164.99 kJ/mol. The value of the apparent activation energy of the roasting process can also be used to predict the rate-controlling step. When the process is controlled by external or internal diffusion, the apparent activation energy is expected to be between 4 and 12 kJ/mol; in contrast, when the process is controlled by the chemical reaction at the surface, the apparent activation energy is expected to be greater than 41.8 kJ/mol. The calculated value of the apparent activation energy confirms that the roasting process is controlled by the chemical reaction at the surface, and the kinetics model for the process can be expressed as follows:
[1-(1-α)1/3]=2.66×105exp[-164990/(RT)]t (8)
where α is the silica extraction rate, R is the molar gas constant (8.314 J/(K·mol)), T is the thermodynamic temperature in K, and t is the roasting time.
3.3.2 Effect of granularity
The effects of granularity and roasting time on the silica extraction rate are studied under the following conditions: roasting temperature of 875 °C and molar ratio of sodium carbonate to potash feldspar of 1.2:1. As shown in Fig. 9, granularity has a significant influence on the silica extraction rate, which increases as the granularity decreases. This is because the reactivity and specific surface area of the potash feldspar increase as granularity decreases. Using the experimental data in Fig. 9, plots of [1-(1-α)1/3] vs roasting time are calculated and presented in Fig. 10. There is clearly a linear relationship between [1-(1-α)1/3] and roasting time, which further indicates that the roasting process is controlled by the chemical reaction at the surface.
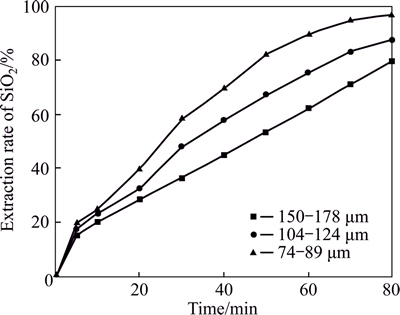
Fig. 9 Effects of granularity and roasting time on silica extraction rate
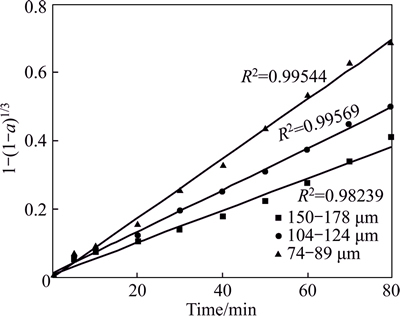
Fig. 10 Relationship between [1-(1-a)1/3] and t at different potash feldspar granularities
3.3.3 Effect of molar ratio of sodium carbonate to potash feldspar
Figure 11 shows the effects of the molar ratio of sodium carbonate to potash feldspar and the roasting time on the silica extraction rate. The molar ratio of sodium carbonate to potash feldspar varies in the range 1:1-1.2:1 at roasting temperature of 875 °C and a granularity of 74-89 μm. It can be seen that the silica extraction rate increases as the molar ratio of sodium carbonate to potash feldspar increases. Excess sodium carbonate, which acts as a fluxing and fluidizing agent, is necessary to improve the reaction conditions and ensure the liquidity of the mixture. It is worth noting that the silica extraction rate does not increase dramatically as the molar ratio of sodium carbonate to potash feldspar increases, which may be attributed to the fact that external diffusion is not the rate-controlling step of the roasting reaction. The corresponding relationship between the value of [1-(1-α)1/3] and the roasting time is shown in Fig. 12; there is a good linear correlation between the two variables. The results further indicate that the roasting process is controlled by the chemical reaction at the surface.
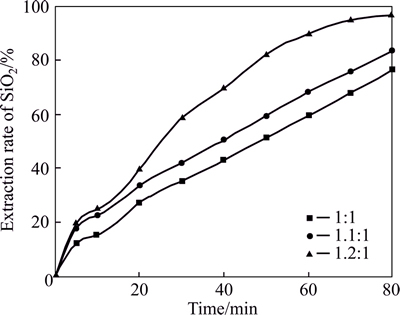
Fig. 11 Effects of molar ratio of sodium carbonate to potash feldspar and roasting time on silica extraction rate
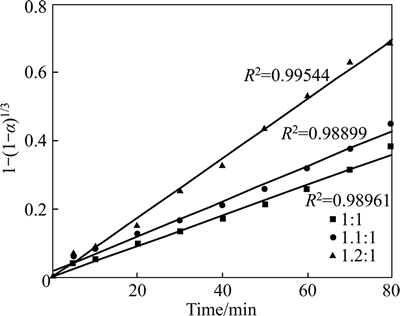
Fig. 12 Relationship between [1-(1-a)1/3] and t at different sodium carbonate to potash feldspar molar ratios
4 Conclusions
1) The effects of roasting temperature, granularity, molar ratio of sodium carbonate to potash feldspar, and roasting time on the rate of silica extraction during potash feldspar roasting are investigated. The results indicate that the silica extraction rate increases with increasing roasting temperature, the molar ratio of sodium carbonate to potash feldspar, and the roasting time, while decreasing granularity also increases the extraction rate.
2) The silica extraction rate is 98% under the optimal conditions, viz., roasting temperature of 875 °C, granularity of 74-89 μm, molar ratio of sodium carbonate to potash feldspar of 1.2:1, and roasting time of 80 min.
3) The kinetics of roasting potash feldspar in the presence of sodium carbonate is described by the shrinking core model and the reaction rate is controlled by the chemical reaction at the particle surface. According to the Arrhenius expression, the activation energy is 164.99 kJ/mol and the process could be expressed as [1-(1-α)1/3]=2.66×105exp[-164990/(RT)] t.
References
[1] SWAPAN K D, KAUSIK D. Differences in densification behaviour of K- and Na-feldspar-containing porcelain bodies [J]. Thermochimica Acta, 2003, 406(1, 2): 199-206.
[2] FERRI L D, LOTTICI P P, VEZZALINI G. Characterization of alteration phases on Potash-Lime-Silica glass [J]. Corrosion Science, 2014, 80(3): 434-441.
[3] TALBOT C J, FARHADI R, AFTABI P. Potash in salt extruded at Sar Pohldiapir, Southern Iran [J]. Ore Geology Reviews, 2009, 35(3, 4): 352-366.
[4] MEENA V S, MAURYA B R, VERMA J P. Does a rhizospheric microorganism enhance K+ availability in agricultural soils [J]. Microbiological Research, 2014, 169(5, 6): 337-347.
[5] GAIED M E, GALLALA W. Beneficiation of feldspar ore for application in the ceramic industry: Influence of composition on the physical characteristics [J]. Arabian Journal of Chemistry, 2015, 8(2):186-190.
[6] CRUNDWELL F K. The mechanism of dissolution of the feldspars: Part I. Dissolution at conditions far from equilibrium [J]. Hydrometallurgy, 2015, 151(1): 151-162.
[7] KAMSEU E, BAKOP T, DJANGANG C, MALO U C, HANUSKOVA M, LEONELLI C. Porcelain stoneware with pegmatite and nepheline syenite solid solutions: Pore size distribution and descriptive microstructure [J]. Journal of the European Ceramic Society, 2013, 33(13, 14): 2775-2784.
[8] MANNING D A C. Mineral sources of potassium for plant nutrition [J]. Sustainable Agriculture, 2011, 2(1): 187-203.
[9] CRUNDWELL F K. The mechanism of dissolution of the feldspars: Part II dissolution at conditions close to equilibrium [J]. Hydrometallurgy, 2015, 151(1): 163-171.
[10] XU Hua, JANNIE S J D. The effect of alkali metals on the formation of geopolymeric gels from alkali-feldspars [J]. Colloids and Surfaces A: Physicochemical and Engineering Aspects, 2003, 216(1-3): 27-44.
[11] LIU Yan-jie, PENG Hui-qing , HU Ming-zhen. Removing iron by magnetic separation from a potash feldspar ore [J]. Journal of Wuhan University of Technology-Materials Science Edition, 2013, 28(2): 362-366.
[12] CICERI D, MANNING D A C, ALLANORE A. Historical and technical developments of potassium resources [J]. Science of the Total Environment, 2015, 502(1): 590-601.
[13] HYNEK S A, BROWN F H, FERNANDEZ D P. A rapid method for hand picking potassium-rich feldspar from silicic tephra [J]. Quaternary Geochronology, 2011, 6(2): 285-288.
[14] JENA S K, DHAWAN N, RAO D S, MISRA P K, MISHRA B K, DAS B. Studies on extraction of potassium values from nepheline syenite [J]. International Journal of Mineral Processing, 2014, 133(10): 13-22.
[15] YUAN Bo, LI Chun, LIANG Bin, LV LI, YUE Hai-rong, SHENG Hao-yi, YE He-ping, XIE He-ping, Extraction of potassium from K-feldspar via the CaCl2 calcination route [J]. Chinese Journal of Chemical Engineering, 2015, 23(9): 1557-1564.
[16] ZHANG Yi, QU Chi, WU Jian-qing, LU Ming, RAO Ping-gen, LIU Xiao-xi. Synthesis of leucite from potash feldspar [J]. Journal of Wuhan University of Technology-Materials Science Edition, 2008, 23(4): 452-455.
[17] XU Jing-chun, MA Hong-wen, YANG Jing. Preparation of β-wollastonite glass-ceramics from potassium feldspar tailings [J]. Journal of the Chinese Ceramic Society, 2003, 31(2): 121-140.
[18] KAUSIK D, SUKHEN D, SWAPAN K D. Effect of substitution of fly ash for quartz in triaxial kaolin-quartz-feldspar system [J]. Journal of the European Ceramic Society, 2004, 24(10, 11): 3169-3175.
[19] NIE Tie-miao, MA Hong-wen, LIU He, ZHANG Pan, QIU Mei-ya, WANG Lei. Reactive mechanism of potassium feldspar dissolution under hydrothermal condition [J]. Journal of the Chinese Ceramic Society, 2006, 34(7): 846-850.
[20] SU Shuang-qing, MA Hong-wen, CHUAN Xiu-yun. Hydrothermal decomposition of K-feldspar in KOH-NaOH-H2O medium [J]. Hydrometallurgy, 2015, 156(7): 47-52.
[21] HU Guo-rong, WANG Jia-liang, PENG Zhong-dong, DU Ke. A novel method to produce potassium chromate from carbon ferrochrome [J]. Journal of Central South University, 2015, 22(4): 966-972.
[22] GALLALA W, GAIED M E. Sintering behavior of feldspar and influence of electric charge effects [J]. International Journal of Minerals, Metallurgy, and Materials, 2011, 18(2): 132-137.
[23] FENG Wu-wei, MA Hong-wen. Thermodynamic analysis and experiments of thermal decomposition for potassium feldspar at intermediate temperatures [J]. Journal of the Chinese ceramic Society, 2004, 32(7): 789-799.
[24] EZEQUIEL C S, ENRIQUE T M, CESAR D, FUMIO S. Effects of grinding of the feldspar in the sintering using a planetary ball mill [J]. Journal of Materials Processing Technology, 2004, 152(3): 284-290.
(Edited by FANG Jing-hua)
Cite this article as: LIU Jia-nan, ZHAI Yu-chun, WU Yan, ZHANG Jun, SHEN Xiao-yi. Kinetics of roasting potash feldspar in presence of sodium carbonate [J]. Journal of Central South University, 2017, 24(7): 1544-1550. DOI: 10.1007/s11771-017-3559-9.
Foundation item: Project(51204054) supported by the National Natural Science Foundation of China; Project(2007CB613603) supported by the National Basic Research Program of China; Project(N140204011) supported by the Ministry of Education Basic Scientific Research Business Expenses, China
Received date: 2016-01-28; Accepted date: 2016-05-19
Corresponding author: ZHAI Yu-chun, Professor, PhD; Tel: +86-13304048096; E-mail: ljn19870305@163.com