J. Cent. South Univ. (2016) 23: 2314-2320
DOI: 10.1007/s11771-016-3289-4

Numerical simulation study on hard-thick roof inducing rock burst in coal mine
HE Jiang(何江)1, 2, 3, DOU Lin-ming(窦林名)1, 2, 3, MU Zong-long(牟宗龙)1, 2, 3,
CAO An-ye(曹安业)1, 2, 3, GONG Si-yuan(巩思园)2,3
1. School of Mines, China University of Mining and Technology, Xuzhou 221116, China;
2. Key Laboratory of Deep Coal Resource Mining of Ministry of Education of China(China University of Mining and Technology), Xuzhou 221116, China;
3. State Key Laboratory of Coal Resources and Mine Safety(China University of Mining and Technology), Xuzhou 221116, China
Central South University Press and Springer-Verlag Berlin Heidelberg 2016
Abstract: In order to reveal the dynamic process of hard-thick roof inducing rock burst, one of the most common and strongest dynamic disasters in coal mine, the numerical simulation is conducted to study the dynamic loading effect of roof vibration on roadway surrounding rocks as well as the impact on stability. The results show that, on one hand, hard-thick roof will result in high stress concentration on mining surrounding rocks; on the other hand, the breaking of hard-thick roof will lead to mining seismicity, causing dynamic loading effect on coal and rock mass. High stress concentration and dynamic loading combination reaches to the mechanical conditions for the occurrence of rock burst, which will induce rock burst. The mining induced seismic events occurring in the roof breaking act on the mining surrounding rocks in the form of stress wave. The stress wave then has a reflection on the free surface of roadway and the tensile stress will be generated around the free surface. Horizontal vibration of roadway surrounding particles will cause instant changes of horizontal stress of roadway surrounding rocks; the horizontal displacement is directly related to the horizontal stress but is not significantly correlated with the vertical stress; the increase of horizontal stress of roadway near surface surrounding rocks and the release of elastic deformation energy of deep surrounding coal and rock mass are immanent causes that lead to the impact instability of roadway surrounding rocks. The most significant measures for rock burst prevention are controlling of horizontal stress and vibration strength.
Key words: hard-thick roof; rock burst; numerical simulation; horizontal stress; stress wave
1 Introduction
Rock burst has currently become one of the major dynamic disasters in coal mining. The existing studies reveal that rock burst occurs at a critical depth [1]. It is generally considered that this critical depth is greater than 400 m. However, some rock bursts occurring in recent years did not support this view. For example, the mining depth of a mine in Xinjiang, China, was only 317 m, but a terrible strong rock burst appeared at a long wall face on October 8, 2010, leading to the broken of ranging arm of shearer, the bending of column of hydraulic support and the death of four miners [2]. Analysis showed that, this rock burst was the product of strong dynamic load resulting from the breaking of siltstone hard roof with a thickness of 12 m above the coal seam.
Actually, rock bursts induced by hard-thick roof are very common. There are now 142 rock-burst coal mines in China [3], and a majority of them are affected by the hard-thick roof. Especially, rock burst struck mining areas like Yima, Huating, Datong, Xuzhou, Yanzhou and Hegang are generally affected by the hard and thick roof [4-5]. The rock bursts emerging in Upper and Lower Silesia of Poland are also caused by the hard and thick roof [6].
Hard-thick roof induced rock burst is mainly reflected in stress concentration formed by stress transfer resulting from hanging roof as well as in destruction of mining surrounding rock induced by instantaneous dynamic load caused by roof movement. This destruction can directly lead to the emergence of rock bursts and other secondary disasters, such as water inrush [7], coal and gas outburst [8], gas explosion and roof fall [9]. Orlecka-Sikora [10] studied the mining induced seismicity in Rudna Mine in the Legnica-Glogow Copper District in Poland, and the results indicated that the area where stress transfer reached 0.05×105 Pa would often experience mining induced seismicity in subsequent mining. By using tomography technology, LURKA [11] investigated rock burst hazards caused by the long wall roof breaking in Zabrze Bielszowice Mine in Poland as well as the dangerous zone, and concluded that P wave velocity is positively correlated with rock burst hazards. LU et al [12] explored the wave spectral characteristics of hard-thick roof induced rock bursts, and the results revealed that the main vibration frequency will reach a very low value when rock burst occurs. In the study of prediction of rock burst by analysis of induced seismicity data, MANSUROV [13] stressed the importance of preparation process in rock burst. In this study, the numerical simulation is conducted to reveal dynamic loading effect of roof vibration on surrounding rocks. In order to reveal the changes in stress of mining surrounding rocks under the effect of hard-thick roof as well as the deformation and destruction process and characteristics of great significance to the revelation of mechanism of hard-thick roof inducing rock burst and the development of reasonable monitoring and control schemes.
2 Mechanism of hard-thick roof inducing rock burst
2.1 Mechanical condition of hard-thick roof inducing rock burst
Hanging roof structure easily forms around the goaf under the hard-thick roof due to the large thickness and fine mechanical performance. The adverse effects of hard-thick roof are in two major aspects: firstly, the load carried by the hard-thick roof hanging transfers to the coal and rock mass around goaf areas, which then generates high stress concentration in coal and rock mass; secondly, when the hard-thick roof reaches to the hanging limit or suffers mining disturbance, breaking will occur and mining-induced seismic events will come into being, causing dynamic load disturbances on mining surrounding rock where high stress concentrates. As long as the static and dynamic combined load meets the rock burst criterion [14-15], namely Eq. (1), rock burst will be induced.
(1)
where σj is the static load carried by mining surrounding rocks; σd is the dynamic load triggered by roof vibration; and [σc] is the critical load for rock burst.
Due to the complexity of geological conditions, the pressure process of hard-thick roof is very complicated, and the weighting steps for roof are also not uniform and equal. In the mining process, some methods and apparatus are often used to monitor the pressure process of the roof. For instance, TRUEMAN et al [16] introduced a specially developed software to identify the control state of long wall roof.
2.2 Characteristics of hard-thick roof fracturing induced dynamic load
Roof breaking will lead to two types of dynamic loads [17]: seismic wave induced stress fluctuation load, expressed as Eq. (2); and constant load increment produced by the broken roof block on coal below.
(2)
where σdp and σds are dynamic loads produced by P wave and S wave, respectively; ρ is medium density; CP and CS are the velocities of P wave and S wave, respectively; vpp and vps are the peak vibration velocities of particle in the propagation process of P wave and S wave, respectively. From the perspective of dynamic load characteristics, the first type of dynamic load is produced rapidly and intensely; The second type of dynamic load will be produced in a slow and weak manner only after the broken roof block gets sunken. As a result, the analysis of the effects of the first type of dynamic load on surrounding rocks of mining space will help to figure out the process of broken roof induced rock burst.
Equation (2) has presented the expression of dynamic load. However, mechanical properties of coal and rock show a strong correlation with loading strain rate, and mechanical response under the effect of dynamic load cannot be analyzed with static methods. Especially, for coal and rock under the complex structure of surrounding rock excavations, their mechanical response is more difficult to analyze and compute. Consequently, FLAC2D, numerical simulation software of rock mechanics, is employed to study the response process of coal under the effect of dynamic load induced by the hard and thick roof fracturing.
3 Numerical simulation model establishment
The numerical model is established based on the geological conditions of 93y3 long wall face in Taoshan Coal Mine. Considering the representativeness of the model, the coal seam thickness and the mining depth are taken as 3 m and 600 m, respectively. The dynamic response of integrated coal beside the roadway around mining-out area under the effect of broken roof induced dynamic load is studied to meet the research goals. The right side of the model presents the mining-out area (goaf) of 93y2 working face, while the left side shows the 93y3 working face. The roadway is 4 m in width and 3 m in height, while the coal pillar is 4 m in width. The model geometry is shown in Fig. 1. The physical and mechanical parameters of strata are listed in Table 1.
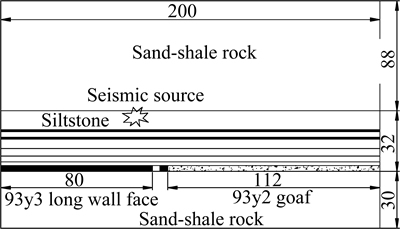
Fig. 1 Model geometry (Unit: m)
Simulation procedures are as follows: Establish the model → Solve the stress equilibrium of origin → Excavate the mining-out area of 93y2 working face → Solve the stress equilibrium→ Excavate the roadway → Solve the stress equilibrium→ Set broken roof induced dynamic load → Study the effect of dynamic load on integrated coal beside the roadway.
According to the energy of mining induced seismic events and the vibration velocity of particle at the hypocenter, the particle at the hypocenter for a mining induced seismic event with a 106 J energy level has a vibration velocity of 8-12 m/s or so. Based on the formula of dynamic load of seismic wave, the dynamic load at the hypocenter is about 60 MPa. Therefore, the dynamic load in the simulation process is taken as 60 MPa; in the meantime, horizontal and vertical dynamic load components are applied. The dynamic load is applied to the roof siltstones 20 m above the integrated coal wall beside the roadway. The line hypocenter with a horizontal length of 10 m is used to simulate the actual surface hypocenter of the mining seismic. The dynamic load lasts for 2 cycles in the form of sine wave with a frequency of 50 Hz, as shown in Fig. 2.
As shown in Fig. 3, two monitoring stations are set up at the depths of 0.5 m and 5 m of integrated coal beside the roadway side to monitor the roadway and show the changes in displacement, particle velocity, and horizontal & vertical stress of the nearby and deep of coal wall.
4 Analyses of simulation results
4.1 Reflection effect of stress wave on free surface
Wave reflection will occur when the seismic wave spreads to the free surface. The superposition of incident and reflected waves will increase the vibration amplitude of particles; when the peaks meet, the amplitude can be increased to 2 times the incident wave. Figure 4 presents the time-history curve of horizontal & vertical vibration velocities of monitoring stations A and B in which the horizontal vibration amplitude of Station B is 1.52 times that of Station A, while the vertical vibration amplitude of Station B is 0.73 times that of Station A, showing a smaller value. If there is no influence of roadway, these two stations will have the same distance with the hypocenter; there is little difference in the relative positions; the difference between the amplitudes of horizontal and vertical vibration velocities would be small. The above results indicate that, significant reflection of the particle can be observed on the roadway free surface when it moves horizontally, while the roadway has a small impact on vertical vibration and the vertical seismic wave does not show any significant reflection phenomenon. As the roadway surface is in the plastic zone, high attenuation exists in seismic wave. Therefore, the amplitude of vertical velocity of Station B is smaller than that of Station A; after the superposition of horizontal incident and transmitted waves, the amplitude of Station A is also less than 2 times that of Station A and the simulation results are consistent with the theoretical analyses. As shown in Fig. 5, due to the reflection of seismic wave, horizontal tensile stress occurs on the roadway free surface; in the meantime, due to the superposition of dynamic and static load, there is also a tensile stress in the vertical direction. The horizontal and vertical tensile stresses reach 1.0 MPa and 1.1 MPa, respectively.
Table 1 Strata properties of model
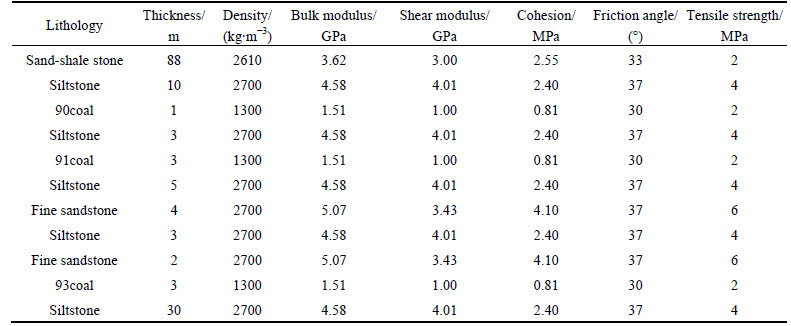
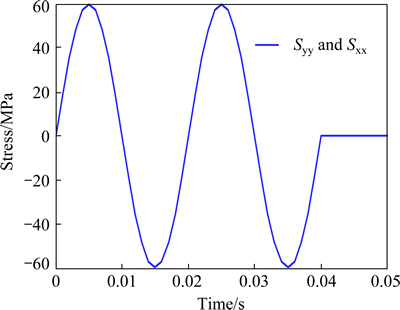
Fig. 2 Stress wave set at hypocenter
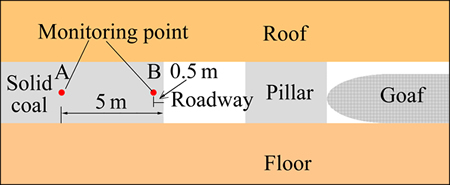
Fig. 3 Settings of monitoring stations
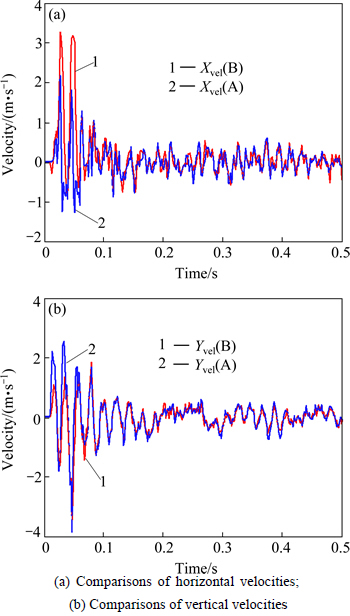
Fig. 4 Comparison of time-history curves of velocities of monitoring stations:
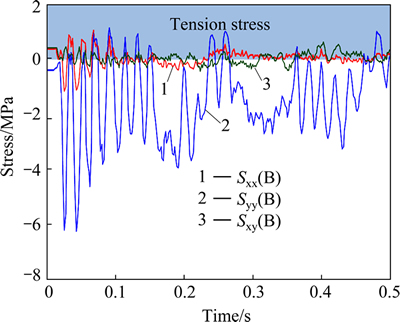
Fig. 5 Tensile stress caused by stress wave reflection on free surface
4.2 Mining dynamic load induced roadway deformation and instability
Roof fracturing induced vibrations spread in the form of stress wave. When they spread near the roadway, surrounding rocks will vibrate and dynamic load will be generated. The superposition of this dynamic and static load of surrounding rocks will cause deformation, destruction or even instability. Figure 6 presents the relationship between horizontal vibration velocity and horizontal stress monitored at Station B. It can be seen from this figure that when stress wave spreads to Station B, a strong horizontal vibration of the particle is generated, which then causes the change in horizontal stress of surrounding rocks; the phase difference between the horizontal stress and the vibration velocity suggests that the change in horizontal stress is a result of particle vibration. After about two cycles of strong vibration, the vibration velocity of particle decreases. However, intense adjustment still exists in horizontal stress of surrounding rocks. This indicates dynamic load can induce the coal and rock in a state of high static load to generate stress and energy release, which then lead to deformation and instability of coal and rock and even the emergence of rock burst.
Figure 7 shows that the instantaneous horizontal displacement on the surface of roadway increases to 140 mm from 72 mm when the dynamic load acts. This process only takes 90 ms. Later, under the disturbance of residual dynamic load, the horizontal displacement does not increase significantly, which suggests that vibration waves play a major role in deformation and instability of coal and rock. The roadway surface instantaneously moves 68 mm. If the roadway does not use reasonable and effective supporting, rock burst will be easily induced. Figure 7(a) indicates that the horizontal displacement of roadway is not directly correlated with the change in vertical stress of surrounding rocks.Figure 7(b) suggests a significant correlation between the displacement of roadway and the change in horizontal stress of surrounding rocks. The horizontal stress experiences significant vibrations in four cycles: in the first and second cycles, horizontal stress changes from compression stress to tensile stress, and roadway displacement increases abruptly two times; in the third and fourth cycles, by although horizontal stress still changes intensely, roadway displacement does not increase obviously.
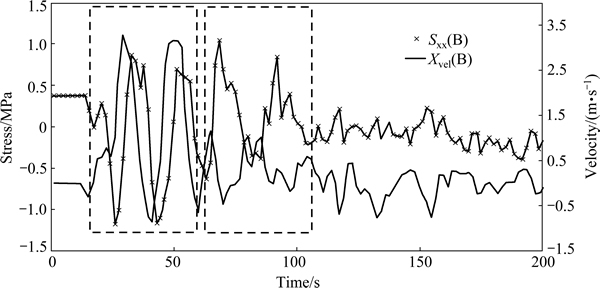
Fig. 6 Relationship between horizontal stress and horizontal vibration velocity of particles at Station B
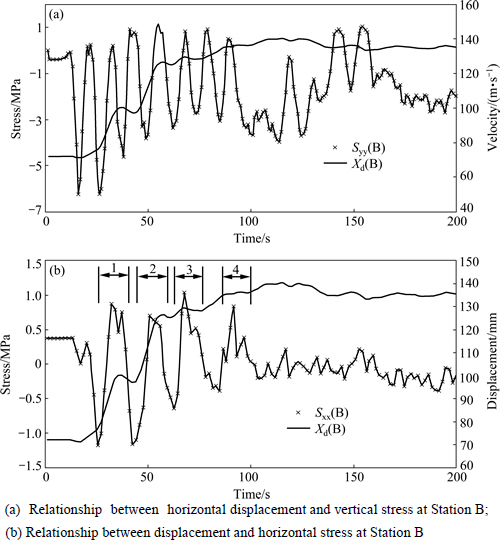
Fig. 7 Relationship between horizontal displacement and stress of surrounding rocks:
Figure 8 presents the four stress fluctuations at Station A corresponding to the four horizontal stress fluctuations at Station B. As stress fluctuates, the residual stress at Station A in the first two cycles declines significantly, which basically reach the residual stress state. This suggests that the displacement of roadway corresponds to the release of stress and energy in the deep coal and rock. The above studies indicate that the combination of mining induced dynamic and static loads lead to the elastic deformation energy stored in the deep coal and rock under static loading, thereby inducing the horizontal displacement of coal and rock around roadway. Figure 9 presents the distribution of stress of surrounding rocks of roadway before and after mining induced dynamic loading, which indicates that after mining induced dynamic loading, coal stress reduces sharply and nearly all the elastic deformation energy stored in the concentrated stress is released.
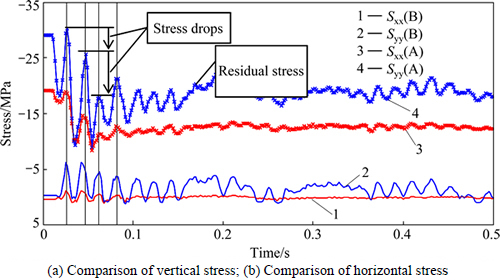
Fig. 8 Comparisons of stresses at Stations A and B:
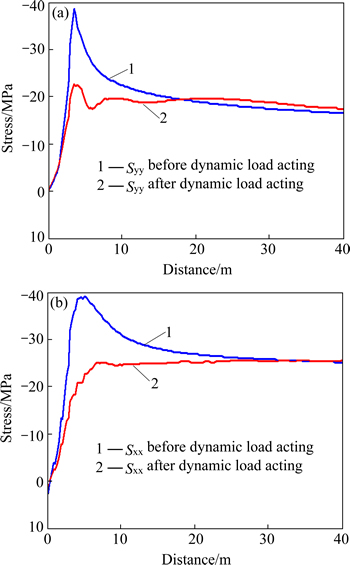
Fig. 9 Comparisons of stresses of roadway surrounding rocks before and after action of seismic waves
5 Conclusions
1) Hard-thick roof induced rock burst is one of the most common and strongest dynamic disasters in coal mine. Hard-thick roof will result in high stress concentration on mining surrounding rocks and the breaking of hard-thick roof will lead to mining seismicity, causing dynamic loading effects on coal and rock mass. High stress concentration and dynamic loading effect combination induces rock burst.
2) The seismic events occurring with roof breaking act on the mining surrounding rock in the form of stress wave that has a reflection on the free surface of roadway and the tensile stress will be generated around the free surface. The horizontal and vertical tensile stresses reach 1.0 MPa and 1.1 MPa, respectively.
3) When the dynamic load acts, the instantaneous horizontal displacement on the surface of roadway increases to 140 mm from 72 mm in 90 ms. Later, under the disturbance of residual dynamic load, the horizontal displacement does not increase significantly, which suggests that vibration waves of dynamic load play a major role in deformation and instability of coal and rock.
4) Horizontal vibration of roadway surrounding particles will cause instant changes in horizontal stress; the horizontal displacement of roadway surrounding rocks is directly related to the horizontal stress but is not significantly correlated with the vertical stress; the increase in horizontal stress of roadway surrounding rocks near surface and the release of elastic deformation energy of deep surrounding coal and rock mass are immanent causes that lead to the impact instability of surrounding rocks of roadway.
References
[1] DOU Lin-ming, HE Xue-qiu. Theory and technology of rock burst prevention [M]. Xuzhou: China University of Mining and Technology Press, 2001. (in Chinese)
[2] LAN Hang, DU Tao-tao, PENG Yong-wei, ZHANG Chuan-jiu, QING Zhi-han. Rock-burst mechanism and prevention in working face of shallow buried coal-seam [J]. Journal of China Coal Society, 2012, 37(10): 1618-1623. (in Chinese)
[3] JIANG Yao-dong, PAN Yi-shan, JIANG Fu-xing, DOU Lin-ming, JU Yang. State of the art review on mechanism and prevention of coal bumps in China [J]. Journal of China Coal Society, 2014, 39(2): 205-213. (in Chinese)
[4] ZHAO Tong-bin, YIN Yan-chun, TAN Yun-liang. Safe mining and new prediction model in coal seam with rock burst induced by roof [J]. Disaster Advances, 2012, 5(4): 961-965.
[5] MU Zong-long, DOU Lin-ming, ZHANG Guang-wen, ZHANG Shi-bin, LI Zhi-hua, ZHANG Jun. Study of prevention methods of rock burst disaster caused by hard rock roof [J]. Journal of China University of Mining and Technology, 2006, 35(6): 737-741. (in Chinese)
[6] HENRYK M. Seismicity in mines due to roof layer bending [J]. Archives of Mining Sciences, 2012, 57(1): 229-250.
[7] DOU Lin-ming, HE Jiang, GONG Si-yuan, SONG Yun-fei, LIU Hui. Case study on microseismic monitoring of goaf water inrush dynamic hazards [J]. Journal of China University of Mining and Technology, 2012, 41(1): 20-25. (in Chinese)
[8] LU Cai-ping, DOU Lin-ming, LIU Hui, LIU Hai-shun, LIU Biao, DU Bin-bin. Case study on microseismic effect of coal and gas outburst process [J]. International Journal of Rock Mechanics and Mining Sciences, 2012, 53: 101-110.
[9] SHEN B, KING A, GUO H. Displacement, stress and seismicity in roadway roofs during mining-induced failure [J]. International Journal of Rock Mechanics and Mining Sciences, 2008, 45(5): 672-688.
[10] ORLECKA-SIKORA B. The role of static stress transfer in mining induced seismic events occurrence, a case study of the Rudna mine in the Legnica-Glogow Copper District in Poland [J]. Geophysical Journal International, 2010, 182(2): 1087-1095.
[11] LURKA A. Location of high seismic activity zones and seismic hazard assessment in Zabrze Bielszowice coal mine using passive tomography [J]. Journal of China University of Mining and Technology 2008, 18(2): 177-181.
[12] LU Cai-ping, DOU Lin-ming, WANG Yao-feng, DU Tao-tao. Microseismic effect of coal materials rockburst failure induced by hard roof [J]. Chinese Journal of Geophysics, 2010, 53(2): 450-456. (in Chinese)
[13] MANSUROV V A. Prediction of rockbursts by analysis of induced seismicity data [J]. International Journal of Rock Mechanics and Mining Sciences, 2001, 38(6): 893-901.
[14] LI Zhen-lei, DOU Lin-ming, CAI Wu, WANG Gui-feng, HE Jiang. Investigation and analysis of the rock burst mechanism induced within fault–pillars [J]. International Journal of Rock Mechanics and Mining Sciences, 2014, 70: 192-200.
[15] HE Jiang, DOU Lin-ming, CAI Wu, LI Zhen-lei, DING Yan-lu. Mechanism of dynamic and static combined load inducing rock burst in thin coal seam [J]. Journal of China Coal Society, 2014, 39(11): 2177-2182. (in Chinese)
[16] TRUEMAN R, LYMAN G, COCKER A. Long wall roof control through a fundamental understanding of shield–strata interaction [J]. International Journal of Rock Mechanics and Mining Sciences, 2009, 46(2): 371-380.
[17] HE Jiang, DOU Lin-ming, CAO An-ye, GONG Si-yuan, LV Jian-wei. Rock burst induced by roof breakage and its prevention [J]. Journal of Central South University, 2012, 19(4): 1086-1091.
[18] QIAN Ming-gao, MIAO Xie-xing, XU Jia-lin. Theory of key stratum in ground control [M]. Xuzhou: China University of Mining and Technology Press, 2003: 10. (in Chinese)
(Edited by DENG Lü-xiang)
Foundation item: Project(51404243) supported by the National Natural Science Foundation of China; Project(2014QNB26) supported by the Fundamental Research Funds for the Central Universities, China
Received date: 2015-04-06; Accepted date: 2015-09-24
Corresponding author: DOU Lin-ming, Professor, PhD; Tel: +86-13952261972; E-mail: lmdou@126.com