
Effects of laser processing parameters on solidification microstructures of ternary Al2O3/YAG/ZrO2 eutectic in situ composite and its thermal property
SU Hai-jun(苏海军), ZHANG Jun(张 军), LIU Lin(刘 林), FU Heng-zhi(傅恒志)
State Key Laboratory of Solidification Processing, Northwestern Polytechnical University, Xi’an 710072, China
Received 10 August 2009; accepted 15 September 2009
Abstract: Rapid surface resolidification with a high powered CO2-laser was performed in preparing directionally solidified Al2O3/YAG/ZrO2 ternary eutectic ceramic in situ composite. The effects of laser processing parameters on the solidification microstructure characteristics and thermal properties were studied by scanning electron microscopy (SEM), energy dispersive spectroscopy (EDS), X-ray diffractometry (XRD) and synthetically thermal analysis (STA). Detailed investigations of the influence of laser power and scanning rate on the preparation and microstructural parameters of the ternary eutectic were presented. Moreover, the eutectic phase separation rule at high temperature was discussed. The results indicate that solidification microstructure of the ternary eutectic composite is greatly influenced by the laser processing parameters. The synthetically thermal analysis shows that the eutectic temperature of ternary Al2O3/YAG/ZrO2 composite is 1 738 ℃, well matching the phase diagram of Al2O3-Y2O3-ZrO2.
Key words: Al2O3/YAG/ZrO2; eutectic in situ composite; ceramic; microstructure; laser resolidification
1 Introduction
In the past few decades, considerable efforts have been devoted to develop high-performance materials with excellent high-temperature strength, which can serve for a long time at above 1 650 ℃ in an oxidizing atmosphere, aiming to improve thermal efficiency of aircraft engines and gas turbines[1-3]. In comparison with conventional superalloys and current SiC/SiC and C/C composites, directionally solidified oxide eutectic ceramic in situ composite possesses higher melting point (above 1 700 ℃), low density (<5 g/cm3), superior high -temperature strength and microstructural stability (almost up to melting point), as well as excellent creep resistance and oxidation resistance. Therefore, it attracts great interest in the fabrication of new ceramic eutectic in situ composite by directional solidification[4-6]. Moreover, the mechanical properties of the oxide eutectics are superior to those of each constituent phase, compared with sintered ceramics, because of the clean and strong interfaces provided by directional solidification[7].
Among a series of oxide eutectic ceramic systems, directionally solidified Al2O3/YAG is considered to be the most promising candidate as ultra-high-temperature structural material[4-9]. For instance, the flexural strength of the composite maintains at 360-500 MPa from room temperature to 1 800 ℃ in air[10]. Also, it possesses outstanding corrosion resistance at high temperatures[11]. However, the binary eutectic ceramic is inherently brittle at room temperature, which prevents its further application[12]. There has been an increasing effort to overcome its shortcoming, such as through microstructure refinement and incorporating third phase reinforcements[13-15]. The incorporation of the third ductile phase, such as ZrO2, is a promising method to overcome the inherent deficiencies of Al2O3/YAG[6]. The three oxides of Al2O3, YAG and ZrO2 are not intermediate compounds, which have good compatibility at high temperatures. It has been reported that flexural strength of Al2O3/YAG/ZrO2 ternary eutectic composite reaches 800 MPa at 1 600 ℃ and the toughness is up to 8.0 MPa?m1/2, which are more than twice as high as that of the Al2O3/YAG binary eutectics[16]. As a result, much more attention has been recently paid to Al2O3/YAG/ZrO2 ternary eutectic composite and various preparation techniques have been developed[13, 17-19].
Laser zone remelting is a recently developed solidification technique for preparing oxide eutectic ceramic in situ composite, which has advantages of nonexistence of crucible, high melting temperature, large thermal temperature gradient (-106 K/m), as well as high growth rate and low cost[14, 20]. This technique has been used to grow Al2O3/YAG binary eutectic composite [14]. However, due to the complexity of oxide physical properties and the non-equilibrium of laser rapid solidification processing, the study on the effects of laser processing parameters on solidification behavior of ternary Al2O3/YAG/ZrO2 eutectic composite is limited up to now. Understanding the solidification behavior and the formation of the composite can help us to recognize the mechanical properties and to gain the expected properties through particular processes. Additionally, the preparation and forming of ternary eutectic ceramic composite are more difficult than those of binary eutectic. In the present study, rapid surface resolidification using a high powered CO2-laser was performed in producing directionally solidified Al2O3/YAG/ZrO2 ternary eutectic ceramic in situ composite, aiming to investigate the effects of laser processing parameters on solidification microstructures and eutectic growth. Also, the high-temperature thermal properties and phase separation rule of the ternary eutectic were investigated.
2 Experimental
2.1 Preparation of eutectic samples
The starting materials were prepared from a mixture of commercial high-purity (>99.99%) nano-powders of Al2O3, Y2O3 and ZrO2 according to the ternary eutectic composition. The molar ratio of eutectic in the Al2O3-Y2O3-ZrO2 ternary system was 65.8/15.6/18.6 [21]. The oxide powders were mechanically mixed by wet ball milling with an aqueous solution of polyvinyl alcohol to obtain homogeneous slurry and then dried at 200 ℃ in air for 1 h. Afterwards, the precursor rods of 7 mm in diameter and up to 60 mm in length were prepared by uniaxially die pressing at 25 MPa for 10 min, followed by sintering at 1 773 K for 2 h to increase the density and provide handing strength.
The directional solidification experiment was performed in a vacuum chamber using a 5 kW CO2 laser (ROFIN-SINAR 850) equipped with a numerically- controlled worktable with 5-axis and 4-direction coupled motion. The precursor samples were located on the laser worktable and exactly moved along the sample axis to scan the sample along the laser direction, as shown in Fig.1. Under the high-energy laser beam, the samples were rapidly remelted and solidified to form the as-solidified eutectic samples. More detailed experimental procedure has been described elsewhere [14]. Finally, the crackless rod eutectic ceramic samples with smooth surface and high density can be obtained.
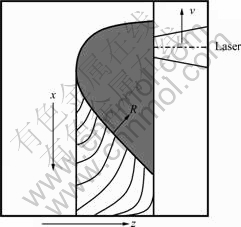
Fig.1 Schematic diagram of laser zone remelting for growing ternary eutectic composite: R-Solidification direction; v-Scanning direction; x, z-Worktable moving direction
2.2 Characterization
The microstructure and phase composition of the ternary eutectic were analyzed by the scanning electron microscopy (SEM, Supra-55), the energy disperse spectroscopy (EDS, Link-Isis) and the X-ray diffraction (XRD, Rigakumsg-158) techniques. Quantitative calculation of the eutectic phase volume fraction was performed by the digital image analysis software of SISC IAS V8.0. The thermal properties and phase separation behavior of the composite were investigated by the simultaneous thermal analyzer (STA, Netzsch-409CD). Approximately 25 mg of the as-solidified sample was heated in a Mo crucible at a heating rate 10 ℃/s up to 1 950 ℃ in a flowing stream of Ar, and the corresponding cooling rate was 10 ℃/s.
3 Results and discussion
3.1 Thermal behavior
The DTA-DDTA curves in the heating and cooling processes are shown in Figs.2(a) and (b), respectively.
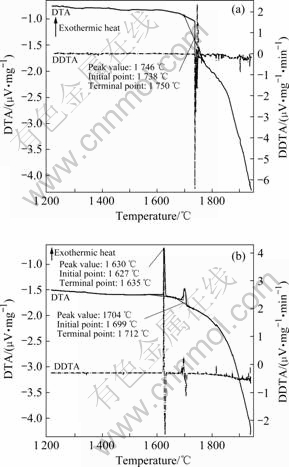
Fig.2 DTA-DDTA curves of laser remelted Al2O3/YAG/ZrO2 ternary eutectic composite: (a) Heating process; (b) Cooling process
For the heating state (Fig.2(a)), it can be clearly seen that there is only a sharp endothermic peak occurring in the temperature range of 1 738-1 750 ℃, indicating the melting of ternary eutectic structure, which well agrees with the eutectic reaction point (1 715 ℃) of the phase diagram of the Al2O3-Y2O3-ZrO2 system[21] and verifies that the composition of the composite lies in the eutectic point or very near the eutectic point. However, during the cooling, there are two exothermic peaks appearing at the temperatures of 1 704 ℃ and 1 630 ℃, respectively, and the latter is much more sharp than the former, as shown in Fig.2(b). The formation may be attributed to the following reasons. On one hand, in the Al2O3-Y2O3-ZrO2 ternary system, there is a very narrow coupled growth zone for eutectic solidification. In common, the ZrO2 is relatively easy to volatilize than Al2O3 or Y2O3 at elevated temperatures. A little volatilization of ZrO2 may drive the composite to deviate the eutectic composition, consequently, resulting in a hypoeutectic composition. Therefore, during the solidification, the first exothermic peak may be due to the nucleation of the primary Al2O3/YAG phase, and then the composite enters into the Al2O3/YAG/ZrO2 equilibrium eutectic solidification process, accordingly, occurring the second obvious exothermic peak. Moreover, in the experimental investigation of Al2O3/YAG/ZrO2 ternary hypoeutectic, the Al2O3/YAG also commonly acts as the primary phase to nucleate[19], thus leading to two exothermic heats. Similar DTA exothermic heats during the solidification process had also been observed by YASUDA et al[22] in the Al2O3-Y2O3-ZrO2 hypoeutectic composite.
On the other hand, for the Al2O3-Y2O3-ZrO2 ternary system the phase selection is determined by the maximum melting temperature before solidification and nucleation of YAG[23-24]. MIZUTANI et al[24] systemically investigated the phase selection of the Al2O3-Y2O3-ZrO2 system. The study indicates that there are two eutectic reactions in the system: one is the Al2O3/YAG/ZrO2 equilibrium eutectic system and the other is Al2O3/YAP/ZrO2 metastable eutectic system. When the melt is cooled from temperature above 1 977 ℃, the nucleation of YAG is completely inhibited and consequently, the Al2O3/YAP/ZrO2 metastable eutectic system is selected. On the contrary, when the eutectic melt is cooled from temperature below 1 977 ℃, the solidification obeys the Al2O3/YAG/ZrO2 equilibrium eutectic path, and is relatively easy to form the Al2O3/YAG/ZrO2 eutectic. Moreover, the addition of ZrO2 can remarkably inhibit the YAG nucleation to form Al2O3/YAP/ZrO2 metastable eutectic. So, the first weak exothermic peak during solidification may also result from the formation of the metastable phase YAP, and then the YAP transforms into YAG, afterwards the melt enters into ternary eutectic solidification. Thus, a strong exothermic peak appears.
3.2 Ternary eutectic microstructure
Fig.3(a) shows the typical microstructure of the cross section of the laser remelted Al2O3/YAG/ZrO2 eutectic ceramic composite. The XRD and EDS analyses show that the directionally solidified Al2O3/YAG/ZrO2 ternary eutectic prepared by laser zone melting consists of α-Al2O3 (the black), YAG (the gray) and ZrO2 (the white) phases without any other phases. The process which effectively avoids existence of other compounds such as YAlO3 or Y2Al4O9 commonly appears in the primary sintered precursor with the sample composition. The Al2O3 and YAG phases are homogenously distributed and interconnected each other, and fine ZrO2 is embedded at the interface of Al2O3/YAG without grain boundaries. Fig.3(b) shows the microstructure of the interface between the solidified zone and non-processed zone in the Al2O3/YAG/ZrO2 eutectic ceramic. It can be seen that the microstructure in the solidified zone exhibits a typical irregular lamellar structure with fine eutectic spacing and has no pore and crack, belonging to typical rapid solidification microstructure. However, in the non-processed zone, the microstructure is coarse and has obvious pores and grain boundaries. In the heat affected zone, it starts to appear coarse solidification microstructure, the grain boundaries gradually disappear and faceted Al2O3 primary phase appears. Further nearing to the solidified zone, the microstructure gradually transits to ternary couple growth with fine eutectic spacing. The above analysis indicates that laser zone remelting can efficiently improve the microstructure and phase constituents of the oxide ceramic. Using the digital image analysis method, as shown in Fig.4, the volume ratio of the eutectic phases is measured to be Al2O3?YAG?ZrO2=40?43?17, which well coincides with that expected for ternary eutectic composition[21].
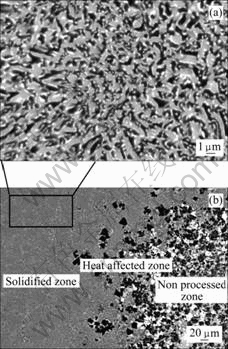
Fig.3 Typical solidification microstructure of cross section of laser remelted Al2O3/YAG/ZrO2 ternary eutectic ceramic (a) and microstructure of interface between solidified zone and non-processed zone (b)
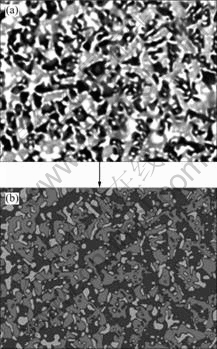
Fig.4 Digital image analysis method for measuring eutectic phase volume ratio: (a) Original SEM image; (b) SEM image processed by two-value segmentation method
3.3 Effects of laser processing parameters on solidification microstructures
Fig.5 shows the typical longitudinal microstructures of the ternary Al2O3/YAG/ZrO2 eutectic ceramic grown at different laser powers and scanning rates. During the laser zone remelting, the laser scanning rate and laser power are the primary solidification processing parameters for successfully preparing the oxide eutectic ceramic composite and determining the microstructure characteristic. Oxide ceramic has a high absorption of laser radiation (>60%)[20], thus only low power (about200 W) can melt the ceramic precursor.
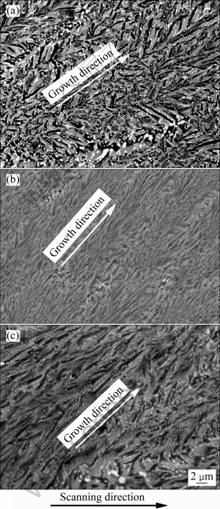
Fig.5 Typical longitudinal microstructures of ternary Al2O3/YAG/ZrO2 eutectic grown at different laser powers (p) and scanning rates (v): (a) P=200 W, v=100 mm/s; (b) P=200 W, v=600 mm/s; (c) P=300 W, v=100 mm/s
One of the important microstructural characteristics during rapid solidification of oxide eutectic composite is the eutectic spacing. Eutectic spacing depends on the thermal effects during solidification and influences the mechanical properties of the solidified ceramic. Figs.5(a) and (b) indicate that with increasing the laser scanning rate, the eutectic microstructure is rapidly refined when the laser power is kept at 200 W. The average eutectic spacing decreases from 0.7 mm down to 0.2 mm as the scanning rate increases from 100 mm/s to 600 mm/s. The ternary eutectic spacing is much smaller than that of the binary eutectic[10, 14]. The refinement of the ternary eutectic microstructure obtained is mainly attributed to rapid solidification rate and high temperature gradient during the laser zone remelting process. Moreover, the addition of ZrO2 also greatly contributes to the microstructure refinement. Furthermore, Figs.5(a) and (c) show that when the laser scanning rate is kept constant, with increasing the laser power, the eutectic spacing is increased. The average eutectic spacing increases from 0.7 mm up to 1.1 mm as the laser power increases from 200 W to 300 W. This is mainly because that when the laser power increases, the corresponding cooling rate is decreased, thus leading to the increase of the eutectic spacing. Similar phenomenon is also found in the laser surface modified alumina ceramic. When further increasing the laser power, the pores may be produced. Additionally, because the solidification direction is gradually changed from bottom to top of the solidified eutectic sample (Fig.1), the growth direction has a deviation from the scanning direction, as the arrows show in Fig.5.
Moreover, laser zone remelting is a non-equilibrium solidification process. The asymmetry of the temperature field, the convection caused by the melt surface tension and gravity, and instability of laser worktable commonly lead to the non-homogenous microstructure in local zone, as shown in Fig.6. In Fig.6, the coarse eutectic microstructure and fine eutectic microstructure coexist in the ternary composite, which will influence the mechanical properties of the composite[6]. Furthermore, the non-steady eutectic growth is intrinsic to faceted crystals[25]. Because Al2O3, YAG and ZrO2 have high entropies of fusion[19], they commonly exhibit faceted growth interface. When the faceted crystal grows, frequent branching of three eutectic phases will occur, as the arrow shows in Fig.6. As a result, a complex entangled ternary eutectic microstructure is formed.
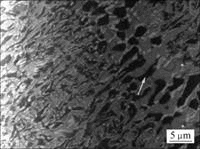
Fig.6 Non-homogenous microstructure and branching of faceted phases in ternary Al2O3/YAG/ZrO2 eutectic composite
4 Conclusions
1) Directionally solidified Al2O3/YAG/ZrO2 ternary eutectic ceramic in situ composite with fine irregular lamellar microstructure was obtained by laser rapid resolidification. The volume ratio of the eutectic phases is Al2O3?YAG?ZrO2=40?43?17. Laser zone remelting efficiently improves the microstructure of the ceramic composite.
2) Synthetically thermal analysis indicates that the eutectic temperature of Al2O3/YAG/ZrO2 obtained is 1 738 ℃, well matching the phase diagram of Al2O3- Y2O3-ZrO2 ternary system.
3) The thermal effects associated with the laser zone remelting significantly influenced the rapid solidification microstructure in laser remelted Al2O3/YAG/ZrO2 ternary eutectic composite. The eutectic spacing decreases with increasing the laser scanning rate, but increases with increasing the laser power.
4) The complex entangled ternary eutectic microstructure in the Al2O3/YAG/ZrO2 composite is formed mainly due to the faceted growth and the branching of the eutectic phases, and non-equilibrium of laser rapid solidification process.
References
[1] CHEN C M, Zhang L T, Zhou W C, Hao Z Z, Jiang Y J, Yang S L. Microstructure, mechanical performance and oxidation mechanism of boride in situ composites [J]. Comp Sci Tech, 2001, 61(7): 971-975.
[2] Williams J C, Starke J E A. Progress in structural materials for aerospace systems [J]. Acta Mater, 2003, 51(19): 5775-5799.
[3] Hirano K. Application of eutectic composites to gas turbine system and fundamental fracture properties up to 1 700 ℃ [J]. J Eur Ceram Soc, 2005, 25(8): 1191-1199.
[4] Waku Y, Nakagawa N, Wakamoto T, Ohtsubo H, Shimizu K, Kohtoku Y. A ductile ceramic eutectic composite with high strength at 1 873 K [J]. Nature, 1997, 389: 49-52.
[5] Martinez F J, Sayir A, Farmer S C. High temperature creep deformation of directionally solidified Al2O3/Er3Al5O12 [J]. Acta Mater, 2003, 51(6): 1705-1720.
[6] Llorca J, Orera V M. Directionally solidified eutectic ceramic oxides [J]. Prog Mater Sci, 2006, 51(6): 711-809.
[7] Orera V M, Merino R I, Pardo J A, Larrea A, Pe?a J I, González C, Poza P, Pastor J Y, Llorca J. Microstructure and physical properties of some oxide eutectic composites processed by directional solidification [J]. Acta Mater, 2000, 48(18/19): 4683-4689.
[8] Waku Y. A new ceramic eutectic composite with high strength at 1 873 K [J]. Adv Mater, 1998, 10(8): 615-617.
[9] Su Hai-jun, Zhang Jun, DeNG Yang-fang, LIU Lin, FU Heng-zhi. A modified preparation technique and characterization of directionally solidified Al2O3/Y3Al5O12 eutectic in situ composites [J]. Scripta Mater, 2009, 60(2): 362-365.
[10] Waku Y, Nakagawa N, Wakamoto T, Ohtsubo H, SHIMIZU K, Kohtoku Y. The creep and thermal stability characteristics of a unidirectionally solidified eutectic composite [J]. J Mater Sci, 1998, 33(20): 4943-4951.
[11] Otsuka A, Waku Y, Kitagawa K, Arai N. Effect of hot corrosive environment on ceramics [J]. Energy, 2005, 30(2/4): 523-533.
[12] Ochiai S, Ueda T, SATO K, Hojo M, Waku Y, Nakagawa N, Sakata S, Mitani A, Takahashi T. Deformation and fracture behavior of an Al2O3/YAG composite from room temperature to 2 023 K [J]. Comp Sci Tech, 2001, 61: 2117-2128.
[13] Calderon-Moreno J M, Yoshimura M. Al2O3-Y3Al5O12 (YAG)-ZrO2 ternary composite rapidly solidified from the eutectic melt [J]. J Eur Ceram Soc, 2005, 25(8): 1365-1368.
[14] Su Hai-jun, Zhang Jun, Cui Chun-juan, Liu Lin, Fu Heng-zhi. Rapid solidification behaviour of Al2O3/Y3Al5O12 (YAG) binary eutectic ceramic in situ composites [J]. Mater Sci Eng A, 2008, 479(1/2): 380-388.
[15] Park D Y, Yang J M. Fracture behavior of directionaaly solidified CeO2- and Pr2O3-doped Y3Al5O12/Al2O3 eutectic composites [J]. Mater Sci Eng A, 2002, 332(1/2): 276-284.
[16] Waku Y, Sakata S, Mitani A, SHIMIZU K, Ohtsuka K, Hasebe M. Temperature dependence of flexural strength and microstructure of Al2O3/Y3Al5O12/ZrO2 ternary melt growth composites [J]. J Mater Sci, 2002, 37(14): 2975-2982.
[17] LEE J H, Yoshikawa A, Murayama Y, Waku Y, Hanada S, FUKUDA T. Microstructure and mechanical properties of Al2O3/Y3Al5O12/ZrO2 ternary eutectic materials [J]. J Eur Ceram Soc, 2005, 25(8): 1411-1417.
[18] Oliete P B, Pe?a J I, Larrea A, ORERA V M, Llorca J, Pastor J Y, Martin A, Segurado J. Ultra-high strength nanofibrillar Al2O3-YAG-YSZ eutectics [J]. Adv Mater, 2007, 19(17): 2313-2318.
[19] Su Hai-jun, Zhang Jun, Tian Jun-jie, Liu Lin, Fu Heng-zhi. Preparation and characterization of Al2O3/Y3Al5O12/ZrO2 ternary hypoeutectic in situ composite by laser rapid solidification [J]. J Appl Phys, 2008, 104(2): 023511-7.
[20] Larrea A, de la Fuente G F, Merino R I, ORERA V M. ZrO2-Al2O3 eutectic plates produced by laser zone melting [J]. J Eur Ceram Soc, 2002, 22(2): 191-198.
[21] Lakiza S M, Lopato L M. Stable and metastable phase relations in the system alumina-zirconia-yttria [J]. J Am Ceram Soc, 1997, 80(4): 893-902.
[22] Yasuda H, MIZUTANI Y, OHNAKA I, Kirihara M, Sakimura T. Application of an optical dta for morphological transition in the Al2O3-YAP-ZrO2 metastable eutectic system [J]. ISIJ International, 2003, 43(11): 1733-1741.
[23] YASUDA H, Ohnaka I, Sugiyama A, Mizutani Y, Sakimura T, Kawaguchi A, Waku Y. Undercooled melt shaping of Al2O3-YAG eutectic composite by melting the Al2O3-YAP eutectic structure [J]. Mater Sci Forum, 2005, 475/479: 2709-2712.
[24] Mizutani Y, Yasuda H, Ohnaka I, Sugiyama A, Takeshima S, Kirihara M, Waku Y. Undercooled melt formation by melting of metastable eutectic structure in Al2O3-Y2O3- ZrO2 System [J]. Mater Trans, 2002, 43(11): 2847-2854.
[25] Nagira T, YASUDA H, Takeshima S, Sakimura T, Waku Y, UESUGI K. Chain structure in the unidirectionally solidified Al2O3-YAG-ZrO2 eutectic composite [J]. J Crystal Growth, 2009, 311(14): 3765-3770.
Foundation item: Project(50772090) supported by the National Natural Science Foundation of China; Project(04G53048) supported by the Aeronautical Science Foundation of China; Project(20040699035) supported by the Specialized Research Fund for the Doctoral Program of Higher Education of China; Project(W018101) supported by the Foundation Research Fund of Northwestern Polytechnical University, China; Project(2007AMM004) supported by the Opening Project of State Key Laboratory for Advanced Metals and Materials, China; Project supported by the Fund of the State Key Laboratory of Solidification Processing in Northwestern Polytechnical University, China; Project supported by the Scientific Research Start-up Foundation for Outstanding Persons in Northwestern Polytechnical University, China
Corresponding author: SU Hai-jun; Tel: +86-13772141015; E-mail: shjnpu@yahoo.com.cn
DOI: 10.1016/S1003-6326(09)60065-0
(Edited by YANG Hua)