DOI: 10.11817/j.ysxb.1004.0609.2021-37702
α钛富氧层增厚动力学模型
崔 岩1,张志波2,陈玉东3,孙新军3
(1. 华北理工大学 冶金与能源学院,唐山 063210;
2. 昆明理工大学 冶金与能源工程学院,昆明 650093;
3. 钢铁研究总院工程用钢研究所,北京 100081)
摘 要:为了实现在工业化生产中对α钛富氧层厚度预测和控制,通过实验研究α钛富氧层在高温空气环境中的形成及增厚过程,讨论热处理温度和时间的影响作用,建立高温(750~850 ℃)空气环境下关于温度、时间的富氧层增厚动力学模型。结果表明:当恒温热处理温度为750~850 ℃时,α钛富氧层厚度x与保温时间t0.5呈正比例关系,且升高热处理温度可显著提高富氧层增厚速度。在此温度范围内,氧原子的扩散激活能约为203473 J/mol,计算曲线与实验数据吻合性较好。结合文献中已有的扩散系数方程和实验测得的富氧层厚度数据,推导得到5个富氧层增厚动力学方程,其中3个方程的计算曲线与实验数据吻合性较好,可为实际生产中预估富氧层厚度提供理论支持。
关键词:α钛;富氧层;氧原子;扩散;动力学计算
文章编号:1004-0609(2021)-01-0057-07 中图分类号:TG166 文献标志码:A
引文格式:崔 岩, 张志波, 陈玉东, 等. α钛富氧层增厚动力学模型[J]. 中国有色金属学报, 2021, 31(1): 57-63. DOI: 10.11817/j.ysxb.1004.0609.2021-37702
CUI Yan, ZHANG Zhi-bo, CHEN Yu-dong, et al. Kinetic model for thickening of α-titanium rich oxygen layer[J]. The Chinese Journal of Nonferrous Metals, 2021, 31(1): 57-63. DOI: 10.11817/j.ysxb.1004.0609.2021-37702
钛及钛合金具有比强度高、耐蚀性能好、生物相容性高等优点,在航空航天、海洋工程、生物医药等领域广泛应用,但因为较低的强度导致硬度低、耐磨性能差[1],特别是α型TA1、TA2等纯钛耐磨性能很差。为了扩展钛材特别是α型纯钛应用于高摩擦环境领域,往往采用多种表面硬化处理技术进行处理[2-3]。氧作为α稳定化元素,在α钛中有很高的固溶度,作为间隙固溶强化元素,能显著提高材料的硬度[4-5],在板坯加热、高温锻造、热轧等生产过程中,由于氧原子的扩散渗入,在钛材表面附近基体内形成一定厚度的富氧硬化区,称之为富氧层[6]。因为间隙固溶原子氧对塑性的损害[7-8],需要采取“抛丸+酸洗”工艺去除富氧层,但富氧层可以作为耐磨材料的天然耐磨层,可以应用于一些对塑韧性要求不高但耐磨要求高的零部件。
钛及钛合金中富氧层的形成过程是氧原子的扩散过程,有较多相关领域的研究报道[9-17]。本文通过实验模拟在高温空气环境中不同温度、时间条件下富氧层的形成及增厚过程,结合理论计算推导得到了α钛富氧层增厚动力学方程,为实现工业化生产中富氧层厚度的预测和控制提供理论支持。
1 实验
实验材料为TA1纯钛热轧板,其化学成分(质量分数)为w(O)=0.05%,w(C)<0.01%,w(N)<0.01%,w(Fe)<0.05%,w(H)<0.001%,余量为Ti。
1) 将热轧钛板切面进行打磨、抛光后,对距离表面分别为9.4、14.8、20.2、23.4、37.4、45.3、61.8和72.6 μm处进行显微硬度测量,压头载荷为9.8×10-2 N。距离表面同一深度测7个位置,为了防止偶然误差的产生,去掉最大值和最小值,而后取该深度的平均硬度。
2) 采用波谱分析仪,对打磨、抛光后的热轧板切面进行氧元素含量测量,测量离表面1、2、3、5、7、10、15、20、25、30和40 μm不同深度氧的含量(质量分数),为了防止偶然误差的产生,每个深度测三个平行值,而后取平均值。
3) 通过酸洗去除富氧层厚度,然后将无富氧层的试样放入箱式空气加热炉中进行热处理,热处理温度为750、800和850 ℃,热处理保温时间分别为0、10、20、35、50、75、100、150和200 min,冷却方式为水冷。整个加热过程实现空气中氧原子向钛基体中的渗透。通过对表面附近不同深度进行显微硬度测量(压头载荷为9.8×10-2 N),以判断富氧层厚度。
2 实验结果
2.1 通过波谱分析法测得富氧层厚度
TA1为典型的α型钛组织,选取TA1热轧钛板作为实验原料,采用波谱分析方式测量距离金属表面不同深度氧原子浓度。由图1可知,高氧原子浓度区域(即富氧层)厚度约为5 μm。
钛暴露在空气中与氧气反应,几分钟之内在金属表面生成几个纳米厚的致密氧化膜,并阻碍钛材进一步氧化。由于试样表面氧化膜的存在,波谱分析测得的氧含量高于实际值,但不影响通过氧原子的浓度梯度分布来判断富氧层的厚度。
2.2 硬度法测量富氧层厚度
受到实验条件限制,无法测量距表面深度低于9 μm的显微硬度值。由图2可见,随着离表面深度x逐渐增大,显微硬度几乎没有变化,表明所测深度范围没有富氧层,由此可知富氧层厚度小于9.4 μm,这个结果与波谱分析测量的富氧层厚度5 μm是一致的。
2.3 加热温度和保温时间对TA1富氧层厚度的影响
因为富氧层中的氧原子有很强的间隙固溶硬化作用,因此富氧层即为硬化层,可以采用硬度法测量富氧层厚度。将750、800和850 ℃保温处理的一系列试样,采用硬度法测量不同保温时间试样的富氧层厚度,得到图3曲线。由图3拟合曲线可见,富氧层厚度x与保温时间t0.5呈正比例关系。加热温度750、800和850 ℃对应的曲线斜率
、
和
分别为
、
和
,这表明温度越高富氧层增厚速度越快。
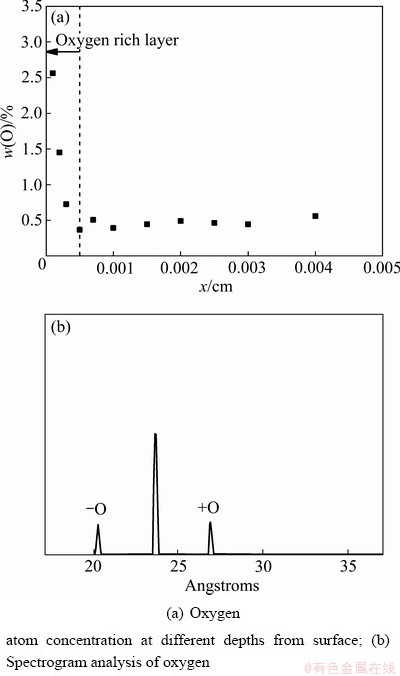
图1 距离基体表面不同深度O元素浓度波谱分析结果
Fig. 1 Spectrum analysis results of oxygen atom concentration at different depths from surface:
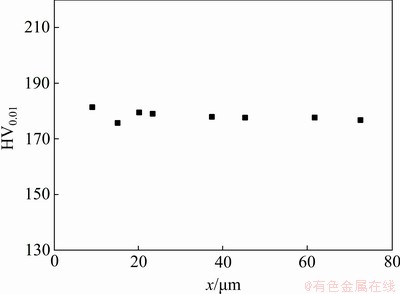
图2 随距离基体表面深度增加,TA1热轧钛板的硬度变化
Fig. 2 Hardness change of TA1 hot rolled titanium plate with increase of depth from surface
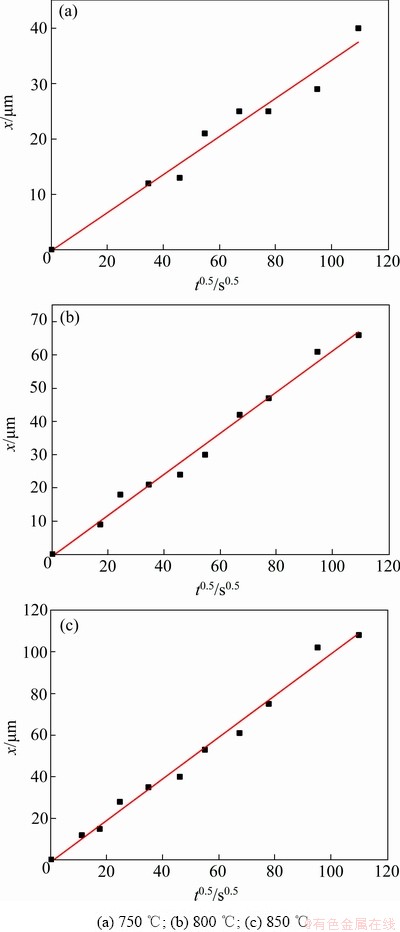
图3 不同保温温度下,α钛富氧层厚度与保温时间之间的关系曲线
Fig. 3 Relationship between thickness of oxygen rich layer and holding time of α-titanium at different holding temperatures
3 分析与讨论
3.1 高温环境下富氧层形成的客观条件
高温时钛金属表面氧化膜为脆性相,受到热轧变形及热内应力作用会出现裂纹,从而促进氧原子向钛基体内进行渗入固溶。
高温环境下会显著增大氧原子向钛基体内扩散渗透速度,促使富氧层快速形成。例如:同样保温200 min,850 ℃加热产生的富氧层厚度分别是800 ℃和750 ℃加热产生富氧层厚度的1.64和2.7倍(见图3)。
在高温时钛金属对氧原子有很高的热力学平衡固溶能力,促进形成高氧原子浓度的富氧层。氧原子在α钛金属中的平衡固溶度w(O)与热力学温度T之间符合如下形式的方程[18]:
(1)
式中:A、B为大于0的常数。由式(1)可知,温度越高,富氧层中平衡固溶氧含量越高。
3.2 富氧层增厚动力学方程
由图3可知,富氧层厚度x与保温时间t0.5接近成正比例关系,根据菲克第二定律推导得到的富氧层厚度与保温时间之间的关系[18]:
(2)
式中x为扩散距离,也是富氧层厚度,cm;λ为常数;DO-α为扩散系数,cm2/s;t为扩散时间,s。
扩散系数通常以Arrhenius形式的关系式给出[18]:
(3)
式中:D0是数值常数(单位一般为cm2/s);R是摩尔气体常数;T是温度;Q是扩散激活能(单位为J/mol)。
将式(3)代入式(2)中,得到
(4)
图3曲线表明,一定温度T下,O原子扩散距离(富氧层厚度)x和保温时间t0.5之间近似呈正比例关系,即:
(5)
式中:kT为温度T时的曲线斜率。
将式(5)代入式(4),得到
(6)
将T1=1023 K、T2=1073 K和T3=1123 K及对应的
3.44×10-5、
6.18×10-5和
10.0025×10-5代入式(6),两两联立求解,得到Q值的三个解,分别为213757 J/mol、203797.5 J/mol和192864.3 J/mol,取平均值得到氧原子向α-Ti扩散的扩散激活能
为203473 J/mol。
将
=203473 J/mol、
3.44×10-5、
6.18×10-5和
10.0025×10-5代入式(6)中,得到
的三个解,取平均值
。将
和
J/mol代入式(3),得到α-钛中富氧层增厚动力学方程:
(1023~1123 K) (7)
根据式(7)进行动力学计算,得到关于t和x的曲线。将动力学计算法与硬度法测得的曲线对比(见图4),发现两种方法得到的曲线基本吻合。式(7)作为富氧层增厚动力学模型,不仅可为耐磨型α型钛合金材料的热处理工艺设计、生产、理论分析提供理论依据,也可以用于预测富氧层厚度,为优化工艺减小富氧层对冷弯等力学性能损害提供理论支持。
3.3 采用扩散系数方程结合富氧层厚度实验数据推导富氧层增厚动力学方程
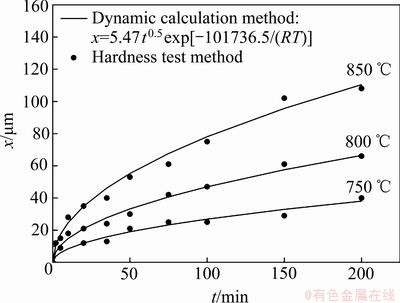
图4 不同保温温度下硬度法和动力学计算法得到富氧层厚度对比
Fig. 4 Comparison of thickness of rich oxygen layer obtained by hardness method and dynamic calculation method at different holding temperatures
表1所列为高温α-Ti中典型的扩散系数方程[19-22]中的D0、Q。结合扩散系数方程和图3实验数据,也可以推导得到富氧层增厚动力学方程(温度适用范围与所对应的扩散系数方程一样),见表2。
举例说明采用扩散系数方程并结合图3实验数据推导富氧层增厚动力学方程。选取如下扩散系数方程[21]进行计算:
(973-1223 K) (8)
由式(6)得到
(9)
将
、
、
及扩散激活能QO-α=201 kJ/mol、D0= 0.45 cm2/s代入式(9)中,得到λ值的三个解,取平均值得到λ=7.10。将 Q=201 kJ/mol、D0=0.45 cm2/s、λ=7.10代入式(4)得到富氧层增厚动力学方程:
(973-1223 K) (10)
3.4 由扩散系数方程推导的富氧层增厚动力学方程的可靠性
选取表2由扩散系数方程推导的增厚动力学方程,发现由不同方程计算的动力学曲线与实验数据吻合性存在差异(见图5)。例如,表2中的2#、3#、5#增厚动力学计算曲线与硬度法测得富氧层厚度曲线吻合良好,比较可靠,可用于预估富氧层厚度。
表1 高温α-钛扩散系数方程中的D0、扩散激活能Q和λ值
Table 1 Pre-exponential constants and activation energies for diffusion of oxygen in high temperature α-titanium
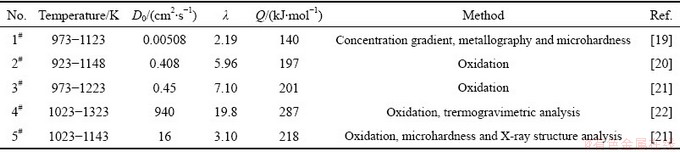
表2 扩散系数方程及对应的增厚动力学方程[19-22]
Table 2 Diffusion coefficient equation and corresponding thickening dynamic equation[19-22]
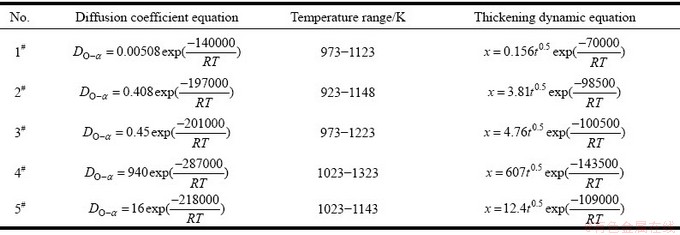
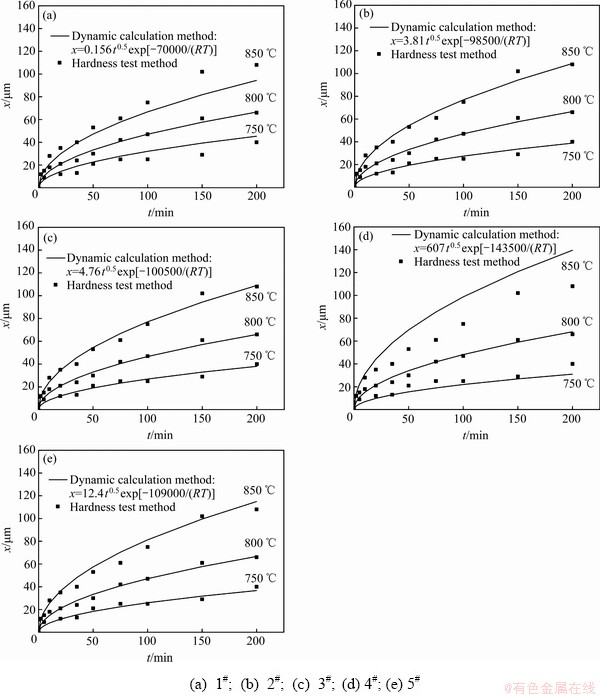
图5 硬度法和动力学计算法得到富氧层厚度对比
Fig. 5 Comparison of thickness of rich oxygen layer obtained by hardness method and dynamic calculation method at different holding temperatures
4 结论
1) 在800~900 ℃高温空气环境中,TA1钛合金中氧原子扩散激活能Q约为203473 J/mol;富氧层增厚动力学模型为
(1023~1123 K),该模型可用于工业化生产中计算和预估富氧层厚度,优化生产工艺。
2) 采用波谱分析法对TA1热轧钛板富氧层厚度的测量值为5 μm。发现当恒温热处理温度在750~850 ℃时,TA1钛板富氧层厚度x与保温时间t0.5之间呈正比例关系,且温度越高富氧层增厚速度越快。
3) 采用扩散系数方程结合富氧层厚度实验数据,推导得到5个富氧层增厚动力学方程,并选取了3个比较可靠的富氧层增厚动力学方程。
4) 高温环境下富氧层的形成与3个因素有关:热变形及热内应力导致氧化皮破裂,从而使金属钛暴露在空气环境中;高温下氧原子扩散速度快;高温环境下氧原子在钛合金基体固溶度高。
REFERENCES
[1] 郭周强, 葛利玲, 袁 航, 等. 钛合金TC4表面纳米化及其热稳定性[J]. 材料热处理学报, 2012, 33(3): 116-120.
GUO Zhou-qiang, GE Li-ling, YUAN Hang, et al. Surface nano-crystallization of TC4 titanium alloy and its thermal stability[J]. Transactions of Materials and Heat Treatment, 2012, 33(3): 116-120.
[2] USTEL F, ZEYTIN S. Growth morphology and phase analysis of titanium based coating produced by thermo chemical method[J]. Vacuum, 2006, 81(3): 360-365.
[3] 刘洪涛, 邓长城. 钛合金镀镍在航空航天工业中应用的可行性研究[J]. 功能材料, 2010, 41(2): 249-252.
LIU Hong-tao, DENG Chang-cheng. Feasibility of Ti alloy plating Ni used in the air industry[J]. Journal of Functional Materials, 2010, 41(2): 249-252.
[4] 陈长军, 马红岩, 张 敏, 等. 钛合金的表面渗氧强化研究进展[J]. 热加工工艺, 2007, 36(14): 63-65.
CHEN Chang-jun, MA Hong-yan, ZHANG Min, et al. Research progress of surface oxygen diffusion hardening of titanium alloy[J]. Hot Working Technology, 2007, 36(14): 63-65.
[5] BLOYEE A. Wear protection of titanium alloy[A]. Surface Performance of Titanium[C]. Cincinnati: The Minerals, Metals and Materials Society, 1996: 155-169.
[6] SHIDA Y, ANADA H. The influence of ternary element addition on the oxidation behavior of TiAl intermetallic compound in high temperature air[J]. Corrosion Science, 1993, 35(5/8): 945-953.
[7] 李铁蕃. 金属的高温氧化和热腐蚀[M]. 北京: 国防工业出版社, 2003.
LI Tie-fan. High temperature oxidation and hot corrosion of metals[M]. Beijing: National Defense Industry Press, 2003.
[8] 贾蔚菊, 曾卫东, 俞汉清, 周义刚. 热暴露对Ti60合金性能及断裂行为的影响[J]. 中国有色金属学报, 2009, 19(6): 1032-1037.
JIA Wei-ju, ZENG Wei-dong, YU Han-qing, ZHOU Yi-gang. Effect of thermal exposure on properties and fracture behaviors of Ti60 alloy[J]. The Chinese Journal of Nonferrous Metals, 2009, 19(6): 1032-1037.
[9] CHAZE A M, CODDET C. Influence of alloying element on the dissolution of oxygen in the metallic phase during the oxidation of titanium alloys[J]. Journal of Materials Science, 1987(4): 1206-1214.
[10] TILEY J, SHAFFER J, SHIVELEY A, et al. The effect of lath orientations on oxygen ingress in titanium alloys[J]. Metallurgical and Materials Transactions A: Physical Metallurgy and Materials Science, 2014, 45(2): 1041-1048.
[11] PRYTULA A O, POGRELYUK I N, FEDRIKO V N. Effect of impregnating atmosphere oxygen on boriding of titanium alloys[J]. Metal Science and Heat Treatment, 2008, 50(5/6): 232-237.
[12] BOLOBOV V I. Mechanism of self-ignition of titanium alloys in oxygen[J]. Combustion Explosion & Shock Waves, 2002, 38(6): 639-645.
[13] KIM Y W. Ordered intermetallic alloys, Part III: Gamma titanium aluminides[J]. JOM, the Journal of the Minerals Metals & Materials Society, 1994, 46(7): 30-39.
[14] DONG H, BELL T. Enhanced wear resistance of titanium surfaces by a new thermal oxidation treatment[J]. Wear, 2000, 238(2): 131-137.
[15] DONG H, LI X Y. Oxygen boost diffusion for the deep-case hardening of titanium alloys[J]. Materials Science and Engineering A, 2000, 280(2): 303-310.
[16] DONG H, BLOYCE A, MORTON P H, et al. Surface engineering to improve tribological performance of Ti-6AI-4V[J]. Surface Engineering, 1997, 13(5): 402-406.
[17] 马红岩, 王茂才, 魏 政. 钛合金的高温固态渗氧-扩散固溶复合处理[J]. 稀有金属材料与工程, 2007, 36(4): 685-689.
MA Hong-yan, WANG Mao-cai, WEI Zheng. Solid oxygen- permeation & diffusion-solution treatment of titanium alloy at high temperature[J]. Rare Metal Materials and Engineering, 2007, 36(4): 685-689.
[18] 雍岐龙. 钢铁材料中的第二相[M]. 北京: 冶金工业出版社, 2006.
YONG Qi-long. The Second phase in iron and steel[M]. Beijing: Metallurgical Industry Press, 2006.
[19] ROE W P, PALMER H R, OPIE W R. Diffusion of oxygen in alpha and beta titanium[J]. Trans ASM, 1960, 52: 191-200.
[20] MICHEL, DECHAMPS, PIERRE, et al. Sur l'oxydation du titane α en atmosphèred'oxygène: Role de la coucheoxydée et mécanismed'oxydation[J]. Journal of the Less Common Metals, 1977, 56(2): 193-207.
[21] LIU Z, WELSCH G.Literature survey on diffusivities of oxygen, aluminum, and vanadium in alpha titanium, beta titanium, and in rutile[J]. Metallurgical Transactions A, 1988,19: 1121-1125.
[22] TIKHOMIROV V I, DYACHKOV V I. Kinetics of the oxygen oxidation of titanium at high temperatures[J]. Russian Journal of Applied Chemistry (Sankt-Peterburg, Russian Federation), 1967, 40(11): 2405-2413.
Kinetic model for thickening of α-titanium rich oxygen layer
CUI Yan1, ZHANG Zhi-bo2, CHEN Yu-dong3, SUN Xin-jun3
(1. College of Metallurgy and Energy, North China University of Science and Technology, Tangshan 063210, China;
2. Faculty of Metallurgical and Energy Engineering, Kunming University of Science and Technology, Kunming 650093, China;
3 Institute of Structural Steels, Central Iron and Steel Research Institute, Beijing 100081, China)
Abstract: In order to predict and control the thickness of oxygen rich layer of α-titanium in industrial production, the formation and thickening processes of oxygen rich layer of α-titanium in high temperature air environment were studied by experiments. The effects of heat treatment temperature and time on the thickness of oxygen rich layer were discussed, and a kinetic model for the thickening of α-titanium rich oxygen layer at high temperature (750-850 ℃) was established. The results show that when the constant temperature is 750-850 ℃, the thickness of oxygen rich layer x and holding time t0.5 of α-titanium plate increase linearly, and the increase of heat treatment temperature can significantly increase the thickening rate of oxygen rich layer. In this temperature range, the diffusion activation energy of oxygen atom is about 203473 J/mol, and the calculated curves are in good agreement with the experimental data. Based on the existing diffusion coefficient equation in the literature and the thicknesses of the oxygen rich layer measured by the experiments, five dynamic equations of oxygen rich layer thickening are derived, three of which are in good agreement with the experimental data and can be used to predict the thickness of oxygen rich layer.
Key words: α-Ti; oxygen rich layer; oxygen atom; diffusion; dynamic calculation
Foundation item: Project(2016YFB0301200) supported by the National Basic Research Development Program of China; Project(E2016209390) supported by the Natural Science Foundation of Hebei Province, China
Received date: 2020-03-09; Accepted date: 2020-09-17
Corresponding author: CUI Yan; Tel: +86-15931928561; E-mail: jackcui5090@163.com
(编辑 王 超)
基金项目:国家重点研发计划资助项目(2016YFB0301200);河北省自然科学基金资助项目(E2016209390)
收稿日期:2020-03-09;修订日期:2020-09-17
通信作者:崔 岩,副教授,博士;电话:15931928561;E-mail:jackcui5090@163.com