Trans. Nonferrous Met. Soc. China 29(2019) 1836-1841
Influence of anodic oxide film structure on adhesive bonding performance of 5754 aluminum alloy
Ying-dong LI, Pi-zhi ZHAO, Ying-juan FENG, Hai-long CAO
Chinalco Material Applications Research Institute Co., Ltd., Beijing 102209, China
Received 22 December 2018; accepted 9 June 2019
Abstract: Three types of anodic films (unsealed, hot water sealed and agent sealed) were prepared to study the effects of anodic film structure on the adhesive bonding performance of AA5754 automotive sheets. The morphology of the anodic films was examined by using scanning electron microscope (SEM) and the composition was examined by glow discharge optical emission spectroscopy (GDOES). The adhesive bonding strength and the durability in corrosive environment were investigated by using single lap-hear test and salt spray test (SST), respectively. The results showed that the unsealed sample could provide high initial bonding strength, but the durability was poor in corrosive environment. The hot water sealed sample could provide high durability, but the bonding strength was low. In contrast, the agent sealed sample displayed porous structure at outer layer and partially plugged nano pores structure at inner layer, providing both excellent bonding strength and durability.
Key words: anodizing; sealing agent; glow discharge optical emission spectroscopy (GDOES); lap-shear; bonding
1 Introduction
Adhesively bonded aluminum joints have been widely used for the realization of lightweight design in industrial applications like automotive and aerospace industry [1,2]. Durable bonds which are capable of taking structural loads are created due to a careful consideration of the way in which the joint is formed, the type of adhesive and the surface treatment procedure [3-6]. Experiences demonstrate that the surface treatment of aluminum alloy is the most critical step to achieve long-term service capability [7]. In practice, chromic acid anodizing (CAA) process provides an anodized layer which has good corrosion resistance while also exhibiting useful adhesion characteristics [8]. However, the use of Cr(VI) is not recommended from the view of environment protection. Phosphoric acid anodizing (PAA) process [8] shows a more open pore structure that allows the penetration of high molecular primers/adhesives and thus is the most widely used pre-surface treatment suitable for adhesive bonding. However, PAA process is not widely applied for a major concern of corrosion. Recently, modified sulfuric acid anodizing (SAA) process has been used as base coating for the adhesion of lacquers in aerospace manufacturing [9]. LUNDER et al [10] found that anodizing in hot sulfuric acid was promising as a simple, very rapid and environmentally friendly pre-treatment for adhesive bonding. SAEEDIKHANI et al [11] studied the process of phosphoric/boric/sulfuric acids anodizing and found that it was a promising pre-treatment for adhesive bonding of aluminum alloys instead of the chromic acid anodizing process. In our previous works, we systematically studied the anodic oxidation behavior of 5xxx aluminum alloy [12-14]. It was found that the traditional hot water sealing process provided certain corrosion resistance, but destroyed the porous structure of anodic film. The bond strength would decrease accordingly and was not suitable for structure bonding [15].
In this work, a simple SAA process including anodizing and sealing procedure was investigated as the pretreatment of 5754 aluminum alloy for structural bonding. A special sealing agent was employed to adjust the structure of anodizing pores. The bonding properties of anodic films with different pores structures were systematically studied.
2 Experimental
5754 aluminum alloy plates (major elements, wt.%: Mg 2.0-4.0, Si 0.05-0.2, Fe 0.2-0.4, Cu 0.05-0.1, Mn 0.1-0.4 ) were cut in sizes of 125 mm × 80 mm × 2 mm for anodizing and single lap-shear testing.
Prior to anodizing, the samples were degreased and alkaline etched in 90 g/L sodium hydroxide electrolyte at 68 °C, and then de-smutted in 200 g/L nitric acid electrolyte at room temperature. Anodizing was carried out in 180 g/L sulfuric acid electrolyte for 15 min with applied current density of 1.4 A/dm2. The applied 5754 aluminum alloy was used as anode while the steel plates were used as cathode. The electrolyte was vigorously stirred to maintain the temperature at 18 °C. Each step was followed by thoroughly rinsed in distilled water.
After anodizing, three samples were prepared.
(1) The unsealed sample was only anodized and rinsed without any further treatments.
(2) The hot water sealed sample was anodized and immerged in de-ionized water for a period of 20 min at 98 °C.
(3) The agent sealed sample was anodized and immerged in de-ionized water added with 3 g/L sealing agent for a period of 20 min at 98 °C. The sealing agent was a mixture of phosphino carboxylic homopolymers.
Afterward, all samples were dried in an air circulation cabinet.
Scanning electron microscopy (SEM) was used to examine the morphology of aluminum alloy after anodizing treatments. Glow discharge optical emission spectroscopy (GDOES) combined sputtering and atomic emission was used for element depth profiling.
Single lap-shear testing was performed by using a commercial epoxy based structural adhesive, Dow betamate 4600F, with joints of 20 mm overlap to evaluate the bonding strength (Fig. 1). The thickness of adhesive was controlled at 0.2 mm by using glass beads and each couple was locked by using two metallic clips. Curing was performed for 30 min at 180 °C in an air circulation cabinet. The bonding tests were carried out with a servo tensile test machine MTS Model C64, where five parallel samples were tested in each case.
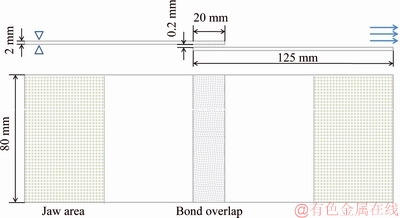
Fig. 1 Shape and dimension of bonding samples
Salt spray test (SST) was performed according to ASTM B117. A solution of 5% NaCl was sprayed on the samples in a closed testing chamber (35 °C). The samples were taken out after different exposure time.
3 Result and discussion
3.1 Characterization of anodizing pores structure
The anodizing process provided uniform and flat anodic layer on the surface and a porous morphology was observed (Fig. 2(a)). The porous film was composed of hexagonal cells with pores size of 5-20 nm in diameter. The hot water sealing procedure modified the surface of anodic layer through hydration reaction, developing a fine and loosen morphology and the porous layer was totally covered (Fig. 2(b)). In contrast, the agent sealed sample displayed a similar porous structure as the unsealed sample (Fig. 2(c)). The presence of sealing agent seemly prevented the formation of hydration compounds on the surface; however, it was still unclear whether the anodized pores at inner layer were effectively plugged.
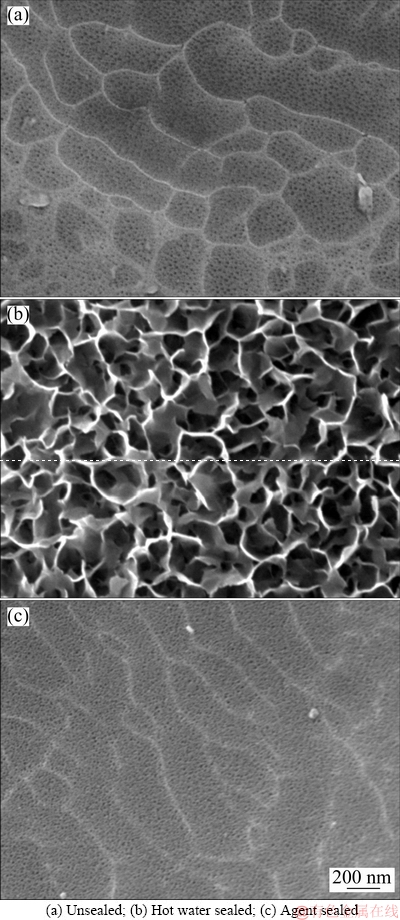
Fig. 2 Surface morphologies of anodized 5754 aluminum alloys
The morphology of inner anodic layer showed that the unsealed sample (Fig. 3(a)) displayed regular pores structure. The channels of pores were uniformly connected from the surface to interior. In contrast, the hot water sealed and agent sealed samples (Figs. 3(b) and (c)) showed that the channels of pores at inner anodic layer were partially plugged. This confirmed that some hydrothermal reactions occurred for both hot water sealed and agent sealed samples during sealing procedure. The anodic film was filled by aluminum hydroxide through volume expansive transformation and the channels of pores from the surface to interior were blocked.
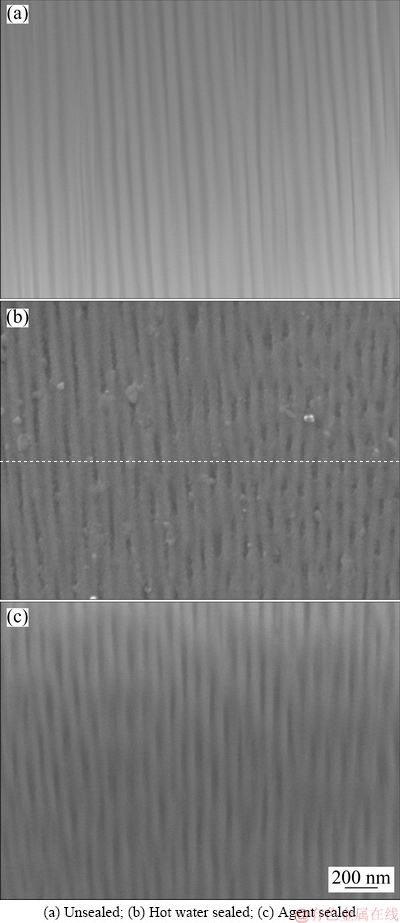
Fig. 3 Cross section morphologies of anodic films
Quantitative GDOES depth profiles of anodized aluminum alloy surfaces provided sufficient composition information along the anodic layer. The concentration of Al element increased gradually while O element decreased with the increase of sputter depth (Fig. 4). Meanwhile, Mg element gradually increased from almost 0 to a constant concentration of 3.5%, which was in good agreement with the alloy composition. The presence of S element was expected to be associated with Al2(SO4)3 that formed during sulfuric acid anodizing process. A peak value of S element was observed locating at the depth position of ~6 μm. This agreed well with the thickness of anodic oxide film.
The distribution of Al element of the three types of anodic films displayed similar variations (Fig. 4(b)). That was associated with the thickness of anodic films. However, the variations of H and O elements displayed some differences (Figs. 4(c) and (d)). In literatures, two major hydrothermal reactions occurred during sealing procedure[16]:
Al2O3+H2O→2AlO(OH) (1)
2Al(OH)+2H2O→Al2O3·3H2O (2)
and thus, the distributions of H and O elements are the critical evidence to determine the distribution of sealing products.
It should be noted that the hot water sealed sample showed significantly high concentrations of H and O elements on the surface. That was related to the flower-like sealing products of Al2O3·3H2O. At inner layer, both hot water sealed and agent sealed samples showed higher concentrations of H and O elements than the unsealed sample. Moreover, the concentrations of H and O elements at inner layer were lower than those on the surface. Thus, the AlO(OH) might be the main substance that plugged the nano-pores.
Therefore, it could be deduced that the presence of sealing agent effectively prevented the formation of Al2O3·3H2O and maintained the original porous morphology of anodic film. However, the inner anodic layer was effectively plugged similar to the hot water sealed sample.
3.2 Bonding property of different pore structures
The bonding property was evaluated by single lap-shear test. Although the eccentricity of the tensile axis of a single lap-shear joint resulted in transverse and peel stress, the tensile test results reported here were in terms of maximum strength or stress at break, assuming that the stresses generating in the adhesive layer were uniform. The curves in Fig. 5 showed that the three types of samples displayed similar yield force, ~24 kN, which was associated with the nature property of the base aluminum alloy materials.
However,the hot water sealed sample rapidly fractured after entering into the plastic deformation zone, displaying the lowest bonding strength, ~26 kN (~16.3 MPa). The fractured surface exhibited an adhesive fracture feature. This implied that the presence of aluminum hydroxide products on the surface significantly impaired the bonding force between the adhesive and anodic film. In contrast, the unsealed samples showed the highest bonding strength,~31 kN (~18.9 MPa). This was because the fluid adhesive could easily penetrate into the nano pores during bonding procedure. After curing, the adhesive would retain in the nano pores, providing a strong bonding strength. The agent sealed sample showed similar bonding strength, ~30 kN (~18.8 MPa), as the unsealed sample.
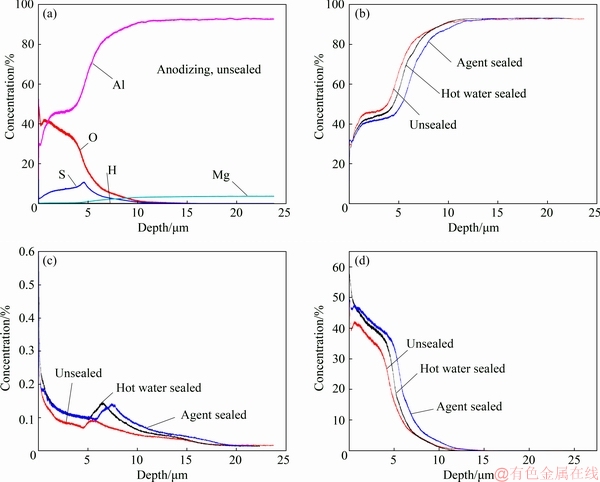
Fig. 4 GDOES depth profiles of anodized 5754 aluminum alloy surfaces: Unsealed sample (a), and separate element profiles of Al (b), H (c) and O (d) for unsealed, hot water sealed and agent sealed samples
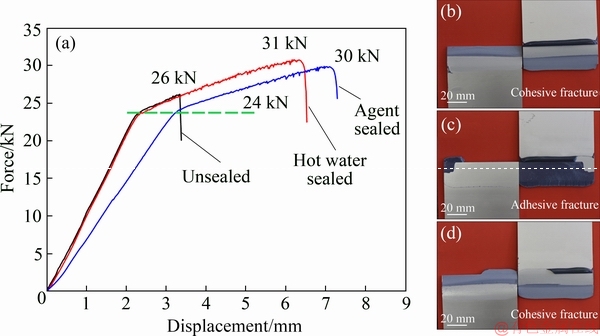
Fig. 5 Representative shear force/displacement curves for samples of different anodizing processes (a), fractured surfaces of unsealed sample (b), hot water sealed sample (c) and agent sealed sample (d)
3.3 Corrosion resistance of bonding joints
As the bonding joint might be prone to degradation in corrosive environment, it was necessary to conduct corrosion tests to investigate the durability of the adhesive joints. The morphologies of different anodizing samples after salt spray test were shown in Fig. 6.
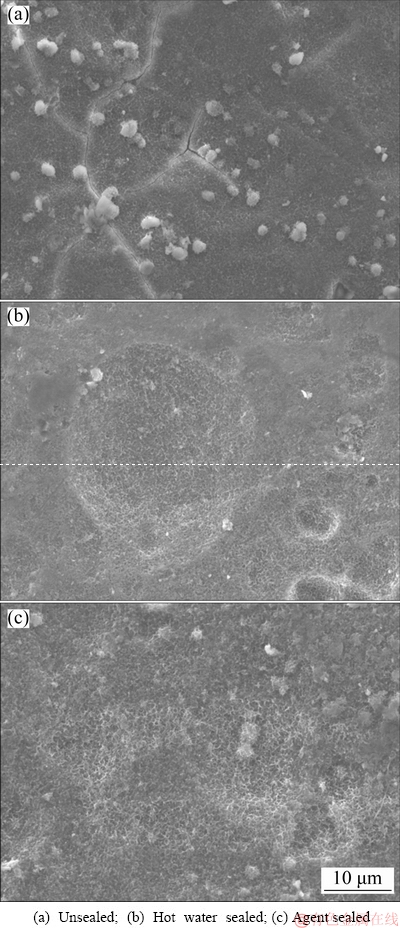
Fig. 6 Surface morphologies of anodized 5754 aluminum alloys after 1000 h of SST
After 1000 h of SST, the unsealed sample showed several cracks on the surface, suggesting that the substrate alloy was severely corroded by the penetration of corrosive ions. The bonding strength would be influenced accordingly. In contrast, both hot water sealed and agent sealed samples were hardly corroded. The two sealed anodic films inhibited the permeation of corrosive ions and provided sufficient corrosion resistance for substrate alloy.
After 1000 h of SST, the single lap-shear test results in Fig. 7 showed that the sealed joints (hot water sealed and agent sealed) hardly displayed any clear loss of bonding strength, approximately less than 2%. However, the strength of unsealed lap joints was significantly lower than the initial strength, displaying nearly 10% of strength loss. This might relate to the corrosion cracks observed on the surface.
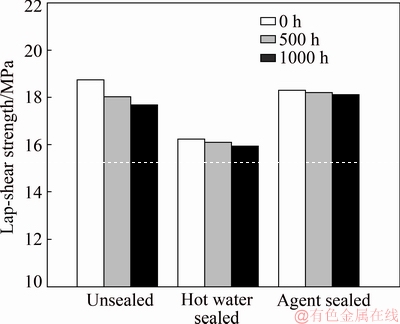
Fig. 7 Single lap-shear test results obtained for adhesive bonded joints of different anodizing processes after 500 and 1000 h of exposure in salt spray
In summary, the agent sealed sample could provide both bonding strength and durability in corrosive environment. The reason was related to its special pores structure. A simple schematic diagram in Fig. 8 illustrated the special structure of agent sealed sample. The outer layer of the anodic film was porous, providing sufficient contact area with the adhesive. However, the inner layer of the anodic film was partially plugged, inhibiting the penetration of corrosive ions. Consequently, both excellent bonding strength and durability were obtained for agent sealed sample.
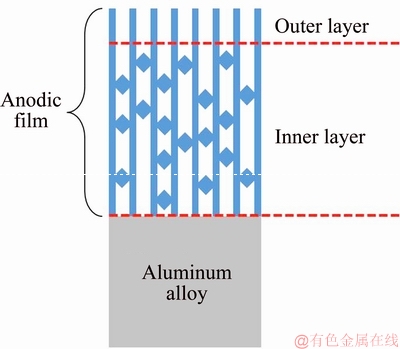
Fig. 8 Schematic diagram of agent sealed pores structure
4 Conclusions
(1) The anodizing pores structures were different for different treatments: The hot water sealed sample formed flower-like hydration products on the surface, the agent sealed sample showed sealing products only within the anodized film.
(2) The hot water sealed sample displayed low bonding strength, and the unsealed sample displayed high initial bonding strength but low durability. In contrast, the agent sealed sample showed both excellent bonding strength and durability.
References
[1] Boutar Y, NaImi S, Mezlini S, Ali M B S. Effect of surface treatment on the shear strength of aluminium adhesive single-lap joints for automotive applications [J]. International Journal of Adhesion & Adhesives, 2016, 67: 38-43.
[2] Barnes T A, Pashby I R. Joining techniques for aluminium spaceframes used in automobiles. Part II: Adhesive bonding and mechanical fasteners [J]. Journal of Materials Processing Technology, 2000, 99: 72-79.
[3] Herrmann J, Suttner S, Merklein M. Experimental investigation and numerical modeling of the bond shear strength of multi-layered 6000 series aluminum alloys [J]. Procedia Engineering, 2017, 183: 283-290.
[4] Leena K, Athira K K, Bhuvaneswari S, Suraj S, Rao V L. Effect of surface pre-treatment on surface characteristics and adhesive bond strength of aluminium alloy [J]. International Journal of Adhesion & Adhesives, 2016, 70: 265-270.
[5] Wu Yong-rong, Lin Jian-ping, Carlson B E, Lu Peng, Balogh M P, Irish N P, Mei Yu. Effect of laser ablation surface treatment on performance of adhesive-bonded aluminum alloys [J]. Surface & Coatings Technology, 2016, 304: 340-347.
[6] Babington C C. Effect of material, geometry, surface treatment and environment on the shear strength of single lap joints [J]. International Journal of Adhesion & Adhesives, 2009, 29: 621-632.
[7] SHI Jian-ming, Pries H, Stammen E, Dilger K. Chemical pretreatment and adhesive bonding properties of high-pressure die cast aluminum alloy: AlSi10MnMg [J]. International Journal of Adhesion & Adhesives, 2015, 61: 112-121.
[8] Thrall E W, Shannon R W. Adhesive bonding of aluminum alloys [J]. Metal Finishing, 2007, 105(9): 49-56.
[9] Elaish R, Curioni M, Gowers K, Kasuga A, Habazaki H, HASHIMOTO T, Skeldon P. Effect of fluorozirconic acid on anodizing of aluminium and AA 2024-T3 alloy in sulphuric and tartaric-sulphuric acids [J]. Surface and Coatings Technology, 2018, 342: 233-243.
[10] LUNDER O, Olsen B, Nisancioglu K. Pre-treatment of AA6060 aluminium alloy for adhesive bonding [J]. International Journal of Adhesion and Adhesives, 2002, 22: 143-150.
[11] Saeedikhani M, Javidi M, Yazdani A. Anodizing of 2024-T3 aluminum alloy in sulfuric-boric-phosphoric acids and its corrosion behavior [J]. Transactions of Nonferrous Metals Society of China, 2013, 23: 2551-2559.
[12] LI Song-mei, Li Ying-dong, Zhang You, Liu Jian-hua, Yu Mei. Effect of intermetallic phases on the anodic oxidation and corrosion of 5A06 aluminum alloy [J]. International Journal of Minerals Metallurgy and Materials, 2015, 22: 167-174.
[13] LI Ying-dong, Zhang You, LI Song-mei, Zhao Pi-zhi. Influence of adipic acid on anodic film formation and corrosion resistance of 2024 aluminum alloy [J]. Transactions of Nonferrous Metals Society of China, 2016, 26: 492-500.
[14] LI Ying-dong, Zhao Pi-zhi, LI Song-mei. Optimization for anodizing conditions of 5A06 aluminum alloy based on doehlert design matrix method [J]. Materials Science Forum, 2017, 877: 498-507.
[15] Hu Nai-ping, Dong Xue-cheng, He Xue-ying, Browning J F, Schaefer D W. Effect of sealing on the morphology of anodized aluminum oxide [J]. Corrosion Science, 2015, 97: 17-24.
[16] Din R U, Gudla V C, Jellesen M S, Ambat R. Accelerated growth of oxide film on aluminium alloys under steam. Part I: Effects of alloy chemistry and steam vapour pressure on microstructure [J]. Surface & Coatings Technology, 2015, 276: 77-88.
阳极氧化膜的结构对5754铝合金胶接性能的影响
李英东,赵丕植,冯莹娟,曹海龙
中铝材料应用研究院有限公司,北京 102209
摘 要:采用5754汽车用铝合金板材制备三种不同类型的阳极氧化膜(未封孔、热水封孔、封闭剂封孔),研究阳极氧化膜的结构对铝合金板材胶接性能的影响。采用扫描电子显微镜(SEM)和辉光放电光谱(GDOES)分别研究阳极氧化膜的显微形貌和成分,采用胶接实验研究不同阳极氧化处理下铝合金板材的胶接接头强度,并结合盐雾实验(SST)研究阳极氧化膜和胶接接头的耐腐蚀性能。结果表明,未封闭试样可以提供高初始胶接强度,但是在腐蚀环境下胶接强度下降;热水封闭的试样在腐蚀环境下胶接强度未出现明显下降,但是胶接强度整体偏低;采用封闭剂处理的试样,膜层结构为外部呈现多孔,内部纳米孔洞被部分封堵,初始胶接强度和耐久性匀很高。
关键词:阳极氧化;封闭剂;辉光放电光谱(GDOES);搭接剪切;胶接
(Edited by Xiang-qun LI)
Foundation item: Project (2018MXJH17) supported by the Technology Foundation of Aluminum Corporation of China
Corresponding author: Pi-zhi ZHAO; Tel: +86-10-66600118; E-mail: zhao_pz@sinr.cn
DOI: 10.1016/S1003-6326(19)65091-0