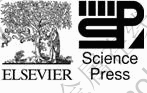
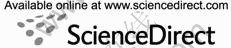
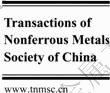
Trans. Nonferrous Met. Soc. China 22(2012) 2529-2534
Synthesis of nanostructured Li2FeSiO4/C cathode for
lithium-ion battery by solution method
YANG Rong1, LIU Xiao-yan1, QU Ye1, LEI Jing1, Jou-Hyeon AHN2
1. School of Science, Xi’an University of Technology, Xi’an 710048, China;
2. Department of Chemical and Biological Engineering and Engineering Research Institute,
Gyeongsang National University, 900 Gajwa-dong, Jinju 600-701, Korea
Received 9 July 2012; accepted 2 August 2012
Abstract: Nanosphere-like Li2FeSiO4/C was synthesized via a solution method using sucrose as carbon sources under a mild condition of time-saving and energy-saving, followed by sintering at high temperatures for crystallization. The amount of carbon in the composite is less than 10% (mass fraction), and the X-ray diffraction result confirms that the sample is of pure single phase indexed with the orthorhombic Pmn21 space group. The particle size of the Li2FeSiO4/C synthesized at 700 ℃ for 9 h is very fine and spherical-like with a size of 200 nm. The electrochemical performance of this material, including reversible capacity, cycle number, and charge-discharge characteristics, were tested. The cell of this sample can deliver a discharge capacity of 166 mA·h/g at C/20 rate in the first three cycles. After 30 cycles, the capacity decreases to 158 mA·h/g, and the capacity retention is up to 95%. The results show that this method can prepare nanosphere-like Li2FeSiO4/C composite with good electrochemical performance.
Key words: lithium-ion batteries; cathode material; Li2FeSiO4/C; solution method
1 Introduction
Li2MSiO4 (M=Mn, Fe, Co) is a new group of electrochemically active silicate-based materials for lithium ion batteries [1-8]. Among these types of materials, Li2FeSiO4, which is a potentially low-cost and safe cathode material for large-scale Li-ion battery application, can deliver a good reversibility on cycling because the presence of strong Si—O bonds can promote the same lattice stabilization effect as that in LiFePO4. In the case of Li2FeSiO4, iron and silicon are the most abundant and lowest cost elements, and hence offer the prospect of preparing cheap and safe cathodes from rust and sand. Both Fe and Si are low cost components, so do the synthesis methods and their precursors. Furthermore, the synthesis methods can be scalable if low cost electrodes can be obtained [9-11].
In the field of electrochemical energy conversion and storage devices, nanostructured materials are becoming increasingly important. It is well known that materials with proper nano-scale dimensions have the potential to dramatically enhance the transportation of electrons and ions, significantly accelerating the rate of chemical and energy transformation processes. So, the development of nanostructured electrode materials is considered the most promising path towards to these goals, such as high energy density, high power density, longer cycle life, and improved safety [12]. Thus far, many efforts have been made to optimize the properties of Li2FeSiO4 materials by reducing the particle size to nanometer scale [13-15]. GONG et al [13] prepared carbon-coated nanostructured Li2FeSiO4 (40-80 nm in diameter) via hydrothermal-assisted sol-gel process. This material has a discharge capacity as high as 160 mA·h/g at a current density of C/16, and it also shows superior high rate capability. ZHANG et al [15] prepared Li2FeSiO4 with 80 nm as average size by a sol-gel method with citric acid. The Li2FeSiO4 has a monoclinic unit cell in space group P21. The maximum discharge capacity of Li2FeSiO4 was 153.6 mA·h/g in the third cycle and 98.3% of the maximum discharge capacity is retained after 80 charge-discharge cycles. However, most of these methods employed complex steps which consumed lots of time and energy (such as reflux).
Therefore, it is necessary to explore a facilitated and effective route for synthesis of Li2FeSiO4 materials with carbon-coating to improve electrical contact among the isolated particles. In the present work, a simple and easy-operation method was used to prepare the precursor at 50 ℃ via a one-step solution approach and then the heat treatment was employed on the Li2FeSiO4/C composite. This approach not only decreases the manufacture time but also allows the creation of unique, sphere-like nano-morphologies. In order to obtain good performance, the duration of sintering was also investigated.
2 Experimental
The precursor of Li2FeSiO4/C was prepared by a solution route. LiCOOCH3·2H2O (purity 99%, Aldrich), FeC2O4·2H2O (purity 99%, Aldrich), and Si(OC2H5)4 (purity 98%, Aldrich), were used as the starting materials and sucrose was used as carbon sources according to the carbon contents (in mass fraction) in the final Li2FeSiO4/C composites (about 10%). All the materials were dissolved in ethanol-water solution(including a amount of CTAB as surfactants) in a molar ratio of 2.1:1:1. The mixture was stirred at 50 ℃ for 4 h and the ethanol was evaporated. The mixture was vacuum dried at 80 ℃ for 12 h. The resulting powders were heat-treated in a horizontal quartz tube in Ar+H2 (4%) atmosphere. The heat-treatments of the materials were carried out at 700 ℃ for 6, 9 and 12 h respectively, thus Li2FeSiO4/C composites were obtained.
The crystallographic structure of the synthesized material was characterized by X-ray powder diffraction analysis (XRD: D8 Adbance Bruker AXS) using Cu Kα radiation. Elemental carbon analysis was performed by elemental analysis (EA1110 CE instruments). Moreover, surface morphologies were observed by field emission scanning electron microscopy (TEM, 200 kV, JEM-2010, JEOL).
The Li2FeSiO4/C composite was mixed with Super-P carbon black (Alfa) and poly(vinylidene difluoride) (PVdF, Aldrich) binder in a mass ratio of 8:1:1. Viscous slurry was obtained by thorough grinding the mixture in nmethyl-2-pyrrolidene (NMP) as the solvent and then was cast uniformly on a thin aluminum foil and dried at 60 ℃ for 12 h. The film was cut into circular discs of area 0.95 cm2 and mass 2.5 mg, and dried in vacuum at 80 ℃ for 12 h to be used as cathodes. The Swagelok cell was assembled with lithium metal anode (300 μm in thickness, Cyprus Foote Mineral Co.), Celgard 2300 separator film, 1 mol/L lithium bis(trifluoromethylsulfonyl)imide (LiTFSI) dissolved in a mixture of ethylene carbonate (EC), propylene carbonate (PC) with a volume ratio of 1:1 as organic solvents, and Li2FeSiO4/C cathode in an argon-filled glove box (H2O content <10×10-6). Then, charge- discharge tests and cycling properties of the cells were evaluated between 1.5 and 4.6 V at C/20 rate using an automatic galvanostatic charge-discharge unit (WBCS3000 battery cycler, WonA Tech. Co.) at room temperature.
3 Results and discussion
3.1 Structure characterization of materials
The thermo-gravimetric spectrum of the precursor is shown in Fig. 1. In the temperature sweep, about 50 % of mass loss is between 35 ℃ and 500 ℃, and the mass loss between 500 and 900 ℃ is insignificant. The spectrum can be divided into three temperature regions, i.e., 35-200 ℃, 200-480 ℃ and 480-900 ℃.
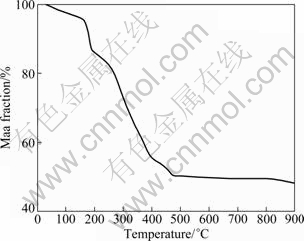
Fig. 1 Thermo-gravimetric trace of starting material for synthesis of Li2FeSiO4/C in N2 atmosphere
There is a mass loss of 15% in the first low temperature range, which can be attributed to the evaporation of water and ethanol. In the temperature range of 200-480 ℃, the mass loss is about 50 %, which is caused by the pyrolysis of the remaining organic compounds and oxalate. The insignificant mass loss in the temperature range of 600-900 ℃ can be considered an indication of reaction completion.
The XRD patterns of the three samples of Li2FeSiO4/C powder shown in Fig. 2 can be indexed to the orthorhombic system with Pmn21 space group. No traces of unreacted starting materials or any iron oxide phase remain in the product. However, no impurity peak was observed in the diffractogram, indicating the reactions for the synthesis went perfectly as expected. The Miller indexes of the diffraction peaks were indexed by MDI Jade 5.0 (Materials Date Inc., Japan) software. With increasing the sintering duration from 6 h to 12 h, the diffraction peaks become high and sharp, indicating the crystallinity increases. The crystal sizes calculated from Scherrer’s equation are 38 nm and 43 nm for the samples sintered at 700 ℃ for 9 h and 12 h respectively. Crystal imperfections such as stress, deformation, twinning, and composition inhomogeneity can also contribute to the peak broadening; hence, the actual value of the crystal size should be larger than that given by Scherrer’s equation. Rietveld refinements were carried out for all the samples and the obtained lattice parameters are listed in Table 1. These results agree with those of TARTE and CAHAY [16], and DOMINKO et al [17], and NISHIMURA et al [3], and closely match those of NYTEN et al [1], while Li2FeSiO4 was proposed to be isostructural with β-Li3PO4. The carbon contents of the samples determined by element analyses are 8.3%, 8.1% and 7.6% for the samples heated at 700 ℃ for 6 h, 700 ℃ for 9 h, and 700 ℃ for 12 h, respectively.
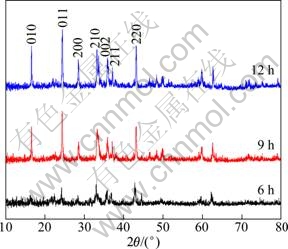
Fig. 2 XRD patterns of Li2FeSiO4/C nanocomposite cathodes sintered at 700 ℃ in Ar+H2(4%) atmosphere and preserved for various time
Table 1 Lattice parameters of Li2FeSiO4 /C samples sintered at 700 ℃ for 6 h, 700 ℃ for 9 h and 700 ℃ for 12 h
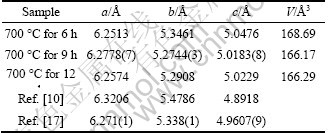
3.2 Morphology of materials
The SEM images of all the samples are shown in Fig. 3. All samples are composed of agglomerated nanoparticles with size of 200-300 nm. Figure 3(a) shows that there are many fine particles and agglomeration in the sample sintered at 700 ℃ for 6 h, which has a wide particle size distribution. There is an improvement in the samples sintered at 700 ℃ for 9 h and 700 ℃ for 12 h, as shown in Figs. 3(b) and (c). With increasing sintering duration, the small particles on the surface grow up and the morphology of particle tends to regulate with increasing crystallinity gradually. Figure 3(d) shows that the particles of the samples sintered at 700 ℃ for 9 h are nanoparticles with a good crystallinity, quite uniform with about 200 nm in radius. Nevertheless, the nanosphere-like morphology of this sample is beneficial for increasing the tap density as the larger size of the secondary particles (~200 nm) allows for a denser packing, while the smaller size of the primary particles (~20 nm) improves the lithium ion and electronic conduction. In Fig. 3(b), the particle size of the sample sintered at 700 ℃ for 12 h become larger than 500 nm.
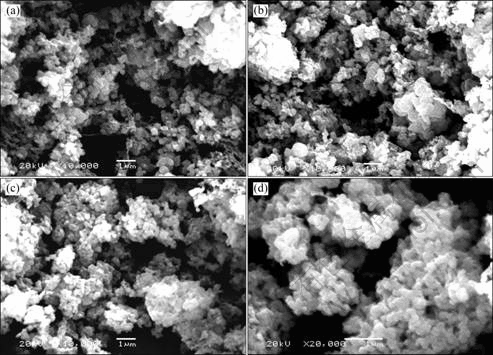
Fig. 3 SEM images of Li2FeSiO4/C samples sintered at 700 ℃ for 6 h (a), 12 h (b) and 9 h (c,d)
TEOS can deliver hydrolysis and alcoholysis in the ethanol-water solution and further a uniform precursor was obtained by this step. After sintering, the small Li2FeSiO4/C particles were gained because not only the precursor was prepared by solution method, but also the sucrose as carbon source was mixed evenly with the starting material in the solution. Small particles are not benefited to the tap density of cathode materials; on the contrary, big particles supply longer distance to the diffusion of Li+ ion in the solid phase. So, appropriate particle size and particle size distribution are benefited for increasing volumetric energy density.
Figure 4 illustrates TEM images of the Li2FeSiO4/C nanocomposites prepared through this solution method and sintered at 700 ℃ for 9 h. These images clearly indicate the appearance of nanoparticles with size less than 30 nm. Consistent with the SEM images, Li2FeSiO4/C adopts a nanosphere-like morphology. The TEM image in Fig. 4(b) shows amorphous carbon coating on the surface of Li2FeSiO4/C nanoparticles. This TEM reveals that the precursor obtained in the system of alcoholysis and hydrolysis of TEOS is easy to product spherical shape particles after heating and the effective use of sucrose as carbon sources during synthesis process prevents particles from growth in the solution phase reaction. Thereafter, during high temperature sintering under inert atmosphere, the sucrose was carbonized and formed as carbon on the surface, which prevents the growth of particles as well as enhances the electronic conductivity of the material.
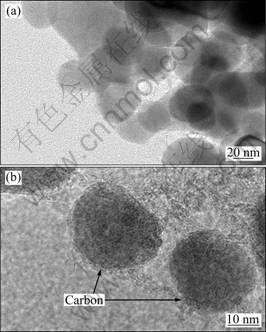
Fig. 4 TEM images of Li2FeSiO4/C nanocomposites prepared by solution method and sintered at 700 ℃ for 9 h
The nanosphere-like Li2FeSiO4/C particles provide not only large surface area to increase the contact area between electrode and electrolyte, but also shorten diffusion length to effectively reduce the distance of the traveling during cycling in the solid state between Li+ ions and electrons, while the carbon coating connects the nanoparticles in close proximity, providing a highly conductive channel for the electron mobility between adjacent Li2FeSiO4/C nanoparticles.
3.3 Electrochemical performance of materials
Galvanostatic charge-discharge measurements were carried out with lithium cells at C/20 rate to evaluate the electrochemical properties of the nanosphere-like Li2FeSiO4/C composite cathodes. The initial three charge-discharge profiles of all the samples at room temperature are shown in Fig. 5. In order to obtain the full charge-discharge behavior of these samples, a wide potential range of 1.5-4.6 V (vs. Li+/Li) was used in the initial cycle. For all these samples, the charge potential in the first cycle is higher than that in the subsequent cycle. The plateau slight above 3 V can be attributed to the phase transition process involving the exchange of lithium and iron between their sites during the first charge [18]. In addition, there is no major structural change occurring after the first charge because the first and second discharge curves are similar.
The samples sintered at 700 ℃ for 6 h and 12 h play initial discharge capacities of 103 mA·h/g and 127 mA·h/g respectively. The nanosphere-like Li2FeSiO4/C sintered at 700 ℃ for 9 h delivers a first three discharge capacity of around 166 mA·h/g, which is close to the value expected for the extraction of one lithium per formula unit (167 mA·h/g) and the oxidation of Fe2+ to Fe3+. It may be due to the good structural stability of the crystal for the cathode obtained at a suitable soaking time.
Figure 6 shows the cycleability of the Li2FeSiO4/C nanocomposite cathodes at room temperature. The nanosphere-like Li2FeSiO4/C sintered at 700 ℃ for 9 h exhibits a stable cycle life with nearly 99% capacity retention in the first 10th cycle, demonstrating its good reversibility and structural integrity during cycling. After the 30th cycle, the discharge capacity of this sample is 158 mA·h/g and the capacity retention is up to 95%. For the sample sintered at 700 ℃ for 12 h, the initial discharge capacity is 127 mA·h/g and the capacity retention is only 79%. The initial discharge capacity of the sample sintered at 700 ℃ for 6 h is 103 mA·h/g, and after 30 cycles it is 88.5 mA·h/g with a capacity retention up to 85%.
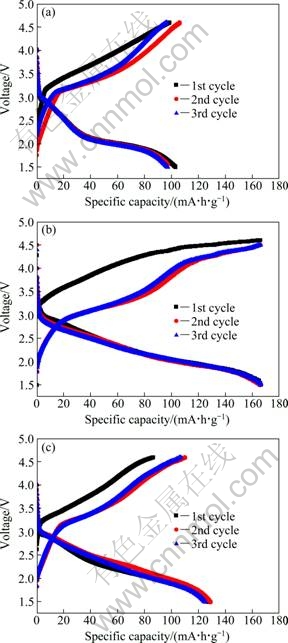
Fig. 5 Initial three charge-discharge profiles of Li2FeSiO4/C nanocomposite cathodes sintered at 700 ℃ for 6 h (a), 9 h (b) and 12 h (c)
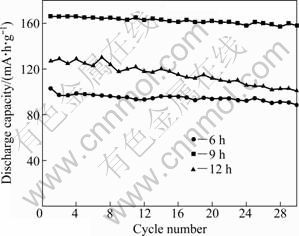
Fig. 6 Cycleability of Li2FeSiO4/C nanocomposite cathodes sintered at 700 ℃ and preserved for various time
Combining the results of SEM,the particles of nanosphere-like Li2FeSiO4/C sintered at 700℃ for 9 h are uniform, fine with good crystallinity, which can promote the diffusion of Li+ ions and increase the practical discharge capacity further.
4 Conclusions
1) The nanosphere-like Li2FeSiO4/C was synthesized via a facilitate approach. The structure, morphology and electrochemical properties of Li2FeSiO4/C were investigated by XRD, SEM, TEM and electrochemical measurements.
2) The XRD and TEM results show that the obtained Li2FeSiO4/C nano particles, with good crystallinity, uniform and about 200 nm in radius, are of an orthorhombic structure with Pmn21 space group, and the lattice parameters of the Li2FeSiO4/C sintered at 700 ℃ for 9 h are a=6.2778(7) ?, b=5.2744(3) ?, c=5.0183(8) ?.
3) The Li2FeSiO4/C nanocomposite can display a discharge capacity of 166 mA·h/g at the first three cycles and a good reversibility.
Acknowledgements
This work was co-supported by the Ministry of Education Key Laboratory of Synthetic and Natural Functional Molecular Chemistry, Education Department of Shaanxi Province (2010JK765) and a fellowship from the NRF of Korea.
References
[1] NYTEN A, ABOUIMRANE A, ARMAND M, GUSTAFFUN T, THOMAS J O. Electrochemical performance of Li2FeSiO4 as a new Li-battery cathode material [J]. Electrochemistry Communications, 2005, 7: 156-160.
[2] LI Xiang-qun, GUO Hua-jun, LI Li-ming, LI Xin-hai, WANG Zhi-xing, OU Hui, XIANG Kai-xiong. Effects of calcination temperature on properties of Li2SiO3 for precursor of Li2FeSiO4 [J]. Transactions of Nonferrous Metals Society of China, 2011, 21: 529-534.
[3] NISHIMURA S I, HAYASE S, KANNO R, YASHIMA M, NAKAYAMA N, YAMADA A. Structure of Li2FeSiO4 [J]. Journal of the American Chemical Society, 2008, 130: 13212-13213.
[4] DOMINKO R, CONTE D E, HANZEL D, GABERSCEK M, JAMNIK J. Impact of synthesis conditions on the structure and performance of Li2FeSiO4 [J]. Journal of Power Sources, 2008, 178: 842-847.
[5] KUGANATHAN N, ISLAM M S. Li2MnSiO4 lithium battery material: Atomic-scale study of defects, lithium mobility, and trivalent dopants [J]. Chemical Materials, 2009, 21: 5196-5202.
[6] GUO Hua-jun, XIANG Kai-xiong, CAO Xuan, LI Xin-hai, WANG Zhi-xing, LI Li-ming. Preparation and characteristics of Li2FeSiO4/C composite for cathode of lithium ion batteries [J]. Transactions of Nonferrous Metals Society of China, 2009, 19: 166-169.
[7] LAN Jian-yun, ZHAO Min-shou. Research progress in cathode material Li2MnSiO4 for Li- ion battery [J]. Battery Bimonthly, 2012, 42(1): 46-49. (in Chinese)
[8] LIU Wen-gang, XU Yun-hua, YANG Rong, HOJAMBERD I M, ZHOU Zhi-bin. Effect of heat treatment temperature on the electrochemical performances of the Li2MnSiO4 /C composite prepared through polyol process [J]. Journal of Inorganic Materials, 2010, 25: 327-331.
[9] DOMINKO R, SIRISOPANAPORN C, MASQUELIER C, HANZEL D, ARCON I, GABERSCEK M. On the origin of the electrochemical capacity of Li2Fe0.8Mn0.2SiO4 [J]. Journal of the Electrochemical Society A, 2010, 157: 1309-1316.
[10] ARMSTRONG A R, KUGANATHAN N, ISLAM M S, BRUCE P G. Structure and lithium transport pathways in Li2FeSiO4 cathodes for lithium batteries [J]. Journal of the American Chemical Society, 2011, 133: 13031-13035.
[11] ISLAM M S, DOMINKO R, MASQUELIER C, SIRISOPANAPORN C, ARMSTRONG A R, BRUCE P G. Silicate cathodes for lithium batteries: Alternatives to phosphates? [J]. Journal of Materials Chemistry, 2011, 21: 9811-9818.
[12] FU L J, LIU H, LI C, WU Y P, RAHM E, HOLZE R, WU H Q. Surface modifications of electrode materials for lithium ion batteries [J]. Solid State Science, 2006, 8: 113-119.
[13] GONG Z L, LI Y X, HE G N, LI J, YANG Y. Nanostructured Li2FeSiO4 electrode material synthesized through hydrothermal-assisted sol-gel process [J]. Electrochemical and Solid-State Letters A, 2008, 11: 60-63.
[14] LI Y X, GONG Z L, YANG Y. Synthesis and characterization of Li2MnSiO4/C nanocomposite cathode material for lithium ion batteries [J]. Journal of Power Sources, 2007, 174(2): 528-532.
[15] ZHANG S, DENG C, YANG S Y. Synthesis of nano-orthosilicate materials for lithium ion batteries [J]. Electrochemical and Solid State Letters A, 2009, 12: 136-139.
[16] TARTE P, CAHAY R. Physical chemistry synthesis and structure of a new family of compounds Li2X2SiO4 and Li2X2GeO4 structurally related to a Li3PO4 [J]. C R Seances Acad Sci, Ser, 1970, C271:777-779. (in French)
[17] DOMINKO R, BELE M, GABERSCEK M, MEDEN A, REMSKAR M, JAMNIK J. Structure and electrochemical performance of Li2MnSiO4 and Li2FeSiO4 as potential Li-battery cathode materials [J]. Electrochemistry Communications, 2006, 8: 217-222.
[18] NYTEN A, KAMAIL S, HAGGSTROM L, GUSTAFFON T, THOMAS J O. The lithium extraction/insertion mechanism in Li2FeSiO4 [J]. Journal of Materials Chemistry, 2006, 16: 2266-2272.
溶液法合成锂离子纳米Li2FeSiO4/C电池正极材料
杨 蓉1,刘晓艳1,曲 冶1,雷 京1,Jou-Hyeon AHN2
1. 西安理工大学 理学院,西安 710048;
2. Department of Chemical and Biological Engineering and Engineering Research Institute,
Gyeongsang National University, 900 Gajwa-dong, Jinju 600-701, Korea
摘 要:以蔗糖为碳源,利用溶液法在温和条件下合成Li2FeSiO4/C的前驱体,煅烧后得到纳米球形Li2FeSiO4/C正极材料。用X射线衍射(XRD)、扫描电子显微镜(SEM)和透射电子显微镜(TEM)对材料的结构和形貌进行表征。通过恒流充放电对材料的电化学性能进行测试。结果表明,采用此法合成的前驱体在700 ℃ 煅烧9 h得到的纳米Li2FeSiO4/C在室温、1.5~4.6 V的电压范围内,于 C/20倍率下前3次放电容量达到166 mA·h/g,30次循环后容量仍保持有158 mA·h/g,容量保持率达95%,表明其具有良好的电化学性能。
关键词:锂离子电池;正极材料;Li2FeSiO4/C;溶液法
(Edited by YANG Hua)
Foundation item: Project supported by Ministry of Education Key Laboratory of Synthetic and Natural Functional Molecular Chemistry, China; Project (2010JK765) supported by the Education Department of Shaanxi Province, China
Corresponding author: YANG Rong; Tel: +86-29-82066360; E-mail: yangrong8622@yahoo.com.cn
DOI: 10.1016/S1003-6326(11)61496-9