
Preparation and photocatalytic activity of neodymium doping titania loaded to silicon dioxide
YANG Xue-ling, ZHU Li, YANG Le-min, ZHOU Wu-yi, XU Yue-hua
Institute of Biomaterial, College of Science, South China Agricultural University, Guangzhou 510642, China
Received 22 March 2010; accepted 15 August 2010
Abstract: Neodymium doping titania was loaded to silicon dioxide to prepare Nd/TiO2-SiO2 by sol-gel method and Nd/TiO2-SiO2 was characterized by X-ray diffractometry (XRD), scanning electron microscopy (SEM), Fourier transform-infrared spectroscopy (FT-IR) and diffuse reflectance spectra (DRS). Photocatalytic activities of Nd/TiO2-SiO2 with different neodymium contents were evaluated by degradation of methyl orange. The light absorption of Nd/TiO2-SiO2 increased with increasing doping neodymium in a visible light range of 388-619 nm, and Nd doping was in favor of decreasing the recombination of photo-generated electrons with holes. Nd and SiO2 improved the photocatalytic activity of TiO2. The optimal molar fraction of Nd to Ti was 0.1%, and the optimum calcination temperature was 600 °C. The highest degradation rate of methyl orange was 82.9% after irradiation for 1 h.
Key words: titania; silicon dioxide; neodymium doping; photocatalysis; sol-gel method
1 Introduction
Photocatalysis allows pollution abatement under mild condition (room temperature and pressure), and TiO2 is the most widely used photocatalyst because of its high photocatalytic activity, non-toxicity, low cost and photochemical stability[1]. However, the light energy used for titania must be equal to or exceed the bandgap of 3.2 eV for anatase, thus, ultraviolet light with wavelength (λ) shorter than 387 nm is required as excitation source[2]. Rare earth ions are known for their f-orbitals to form complexes with various Lewis bases and their oxides having characteristics of adsorption selectivity and thermal stability[3]. In recent years, many groups have examined the effect of rare earth ions doping on photocatalytic properties of TiO2 and found the bandgap of the catalysts shift into the visible region[4-6]. However, practical applications of photocatalysis are limited because of the difficulty in separation and recycling of TiO2 powder from the reaction medium. An alternative method is to immobilize TiO2 powder on supporters, such as glass[7], SiO2[8] and activated carbon[9]. Supported photocatalysts are often prepared by different methods, such as sol-gel processes[10], liquid phase deposition[11] and electrochemical deposition[12]. Compared with other methods, sol-gel method presents certain advantages, such as the possibility of deposition onto complex-shaped substrates, obtaining uniform pore size, high pure and homogeneous products at low temperature and easy control of the doping level.
In this work, neodymium doping TiO2 was loaded to SiO2 to prepare Nd/TiO2-SiO2 using inexpensive SiO2 as supporter. The Nd/TiO2-SiO2 samples were characterized by XRD, SEM, DRS and FT-IR, and their photocatalytic activity to the degradation of methyl orange was also investigated. The aim of this work was to obtain high efficient photocatalysts which were convenient for recycling, and the description of the chemical and physical properties providing a deeper understanding of the photocatalytic mechanism.
2 Experimental
2.1 Preparation of Nd/TiO2-SiO2 photocatalysts
Nd/TiO2-SiO2 photocatalysts with Nd-doping content from 0.05% to 0.8% (molar fraction) were prepared by sol-gel method. The size of commercial SiO2 particles was in the range of 75-150 μm. The preparation was carried out as follows: pure alcohol and diethanolamine (DEA) were added to titanium(IV)-n-butoxide (Ti(OBu)4), and then a given amount of alcohol solution of neodymium nitrate was added dropwise under stirring for 30 min. The molar ratio of Ti(O-Bu)4 to EtOH to DEA was 10:234:10. SiO2 of 2 g was heated at 180 °C in an oven for 2 h and mixed with 50 mL pure alcohol, 0.5 mL redistilled water and 1.2 g cetyltrimethylammonium bromide (CTAB) under stirring at 25 °C. After the adsorption equilibrium was reached over 12 h, the solution of Ti(O-Bu)4 was added dropwise under ultrasonic treatment for different time. The solution was refluxed and most solvent was evaporated at 50 °C. Then the samples were dried at 70 °C. The above samples were annealed in air at different temperatures for 3 h and sieved using standard sieves with sizes of 0.075 and 0.150 mm, respectively. A series of Nd/TiO2-SiO2 photocatalysts with Nd-doping content ranging from 0.05% to 0.8% were obtained. TiO2/SiO2 without neodymium nitrate was prepared for comparison.
2.2 Characterization
X-ray diffraction (XRD) spectra were recorded with a MSAL-XD2 diffractometer (λ=0.154 06 nm) using Cu Kα radiation and standard Bragg-Brentano diffraction geometry. The morphology was characterized by scanning electron microscope (SEM, FEI-XL30). FT-IR spectra were recorded using a Nicolet-510P infrared spectrophotometer and referenced to KBr. Diffuse reflectance spectra were performed using a HITACHI U-3010 UV-Vis spectrophotometer in the range of 200-700 nm and referenced to BaSO4.
2.3 Photocatalytic activity
Aqueous slurries were prepared by adding 0.4 g photocatalyst to 800 mL methyl orange aqueous solution with concentration of 20 mg/L, and irradiations were performed using a high-pressure mercury lamp of 125 W. The aqueous slurries were stirred and bubbled with oxygen for 30 min prior to the irradiation. At 10 min intervals, aliquots of suspension were extracted. The filtrates were analyzed with a spectrophotometer by measuring their absorbances at 465 nm. The methyl orange photocatalytic degradation was evaluated using a plot of co/c versus time (t), where co and c denote the methyl orange concentrations at t=0 and t=t, respectively.
3 Results and discussion
3.1 X-ray diffraction analysis
Fig.1 shows the XRD patterns of 0.1% Nd/TiO2- SiO2 calcined at different temperatures. The crystal phase of Nd/TiO2-SiO2 calcined from 450 to 800 °C was all anatase. With further increase of the calcination temperature to 900 °C, Nd/TiO2-SiO2 was a mixture of
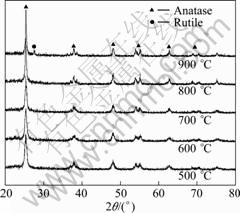
Fig.1 XRD patterns of 0.1% Nd/TiO2-SiO2 calcined at different temperatures
anatase and rutile. It was shown that Nd/TiO2-SiO2 displayed good thermal stability because both SiO2 and Nd inhibited the anatase-rutile phase transformation of TiO2[13-14].
3.2 SEM analysis
The SEM images of SiO2 and 0.1% Nd/TiO2-SiO2 calcined at 600 °C are shown in Fig.2. Fig.2(a) shows that the surface of commercial SiO2 was slick, and some nanometer particles were on its surface. Fig.2(b) shows that a layer of Nd/TiO2 was obvious on the surface of SiO2, and its surface was uniform.
3.3 FT-IR spectra analysis
Fig.3 shows the FT-IR spectra of 0.1% Nd/TiO2- SiO2 calcined at different temperatures. The multiple absorption bands in the region of 420-800 cm-1 could be assigned to various vibration modes of Ti—O bond, and the absorption bands at about 3 500 and 1 640 cm-1 were attributed to stretching vibrations of physically adsorbed water and hydroxyl. With increasing calcination temperature, the broad peak at 450 cm-1 gradually sharpened, indicating the increase of particle size[15], and the absorption band intensity of physically adsorbed water and hydroxyl increased. The broad peaks at 962 and 1 095 cm-1 were assigned to the vibration of Si—O—Ti and the asymmetric stretching vibration of Si—O—Si, respectively. The broad peak at 962 cm-1 indicated that TiO2 was supported on the surface of SiO2, and a Ti—O—Si bond formed[16].
3.4 DRS analysis
The diffuse reflectance spectra indicated the effect of Nd-doping content on the UV-vis absorption of Nd/TiO2-SiO2 calcined at 600 °C, as shown in Fig.4. It was seen that the light absorption of Nd/TiO2-SiO2 in the visible region of 506-619 nm (see the inset in Fig.4) was
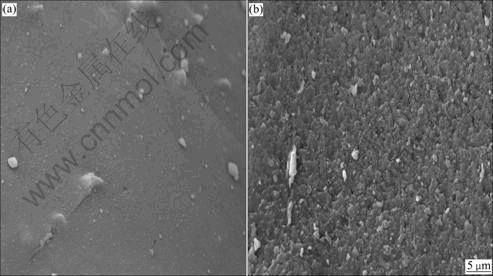
Fig.2 SEM images of SiO2 (a) and 0.1% Nd/TiO2-SiO2 (b)
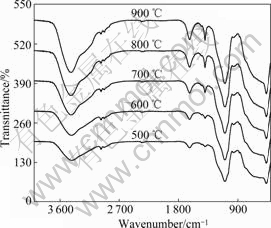
Fig.3 FT-IR spectra of 0.1% Nd/TiO2-SiO2 calcined at different temperatures
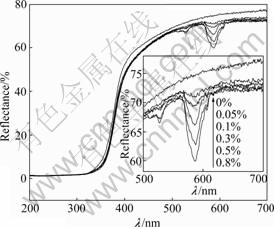
Fig.4 DRS of Nd/TiO2-SiO2 with different Nd-doping contents calcined at 600 ℃
higher than that of TiO2-SiO2, and increased with increasing neodymium content. It was generally thought that the visible light absorption was caused by the new energy level in the bandgap[17], and the visible light absorption of Nd/TiO2-SiO2 could be attributed to the charge transfer between the TiO2 valence or conduction band and the 4f level of neodymium ion[4, 17]. As a result, Nd/TiO2-SiO2 showed a trapping level which decreased the TiO2 band-gap, and Nd/TiO2-SiO2 had visible-light absorption.
3.5 Photocatalytic studies
Fig.5 shows the effect of Nd-doping contents on the photocatalytic activity of Nd/TiO2-SiO2. It was shown that the photocatalytic activity of Nd/TiO2-SiO2 increased with increasing neodymium content and then decreased when Nd-doping content was up to 0.1%. The optimum Nd-doping content was 0.1%, and the degradation rate of methyl orange was 82.9%. The photocatalytic activity of Nd/TiO2-SiO2 was lower than that of TiO2-SiO2, and the degradation rate of methyl orange was 72.1% when the Nd-doping amount was up to 0.3%. Metal ions were the acceptors of electrons, and Nd3+ could trap photoexcited electrons at the TiO2 conduction band. So Nd3+ could trap electrons to inhibit the recombination of electrons with holes. Moreover, the electrons trapped in Nd3+ sites were subsequently transferred to the adsorbed O2, and the recombination rate of electrons with holes decreased. However, it became the recombination center of electrons with holes, when the neodymium doping content increased further [18]. Consequently, it was understandable that an optimum Nd-doping content existed. The UV-vis spectra showed that the neodymium doping improved the light utilization efficiency of Nd/TiO2-SiO2, and generated more electron-hole pairs under light irradiation, which
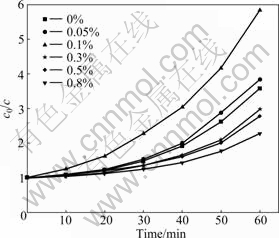
Fig.5 Effect of neodymium content on photocatalytic activity of Nd/TiO2-SiO2
helped to enhance the photocatalytic activity of Nd/TiO2-SiO2. However, when the Nd-doped content was surplus, even though the visible-light absorption ability was improved, the photocatalytic activity was low due to more recombination centers. At this time, the recombination center of electron-hole pairs was the main factor of photocatalytic activity for photocatalysts.
3.6 Interband photocatalysis mechanism for Nd/TiO2- SiO2
Fig.6 shows interband photocatalysis mechanism of Nd/TiO2-SiO2 under irradiation by a high-pressure mercury lamp. The atomic f shells of neodymium were partially filled with electrons. The electrons in Nd3+ 4f3 orbital which absorbed proper photons energy of visible light could be excitated and then transited [19]. A new electronic state in the middle of the TiO2 band-gap would appear due to neodymium doping. This interband Nd3+ trap site in Nd/TiO2-SiO2 captured electrons which were excited from the valence band of TiO2 by absorbing visible-light photons [20]. The 2p electrons of the oxygen anion of oxide generally transited into the valence orbitals of neodymium cations during the charge transfer process (O2-→ Nd3+). In addition, the irradiation
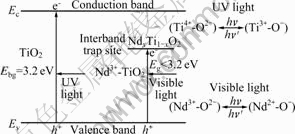
Fig.6 Interband photocatalysis mechanism of Nd/TiO2-SiO2 under irradiation by high-pressure mercury lamp
by high-pressure mercury lamp gave rise to the different charge transfer excited state ion-pairs (Nd2+-O-), which was attributed to the additional interband. Therefore, neodymium doping accelerated the interfacial electron transferring process and changed the band-gap energy of semiconductor TiO2, and Nd/TiO2-SiO2 could be excited by a visible light.
4 Conclusions
1) The crystal phases of Nd/TiO2-SiO2 calcined from 450 to 800 °C were all anatase. With further increasing the calcination temperature to 900 °C, Nd/TiO2-SiO2 was a mixture of anatase and rutile. Both SiO2 and Nd inhibited the anatase-rutile phase transformation of TiO2.
2) FT-IR analysis showed that Ti—O—Si bond in Nd/TiO2-SiO2 was formed. Nd/TiO2-SiO2 had visible absorption in the range of 400-600 nm, and the light absorption at 584 nm increased with increasing neodymium content. An optimal Nd-doping content was beneficial to decreasing the recombination of photo-generated electrons with holes and enhancing the photocatalytic activity of Nd/TiO2-SiO2.
3) 0.1% Nd/TiO2-SiO2 calcined at 600 °C exhibited the highest photocatalytic activity to methyl orange photocatalytic oxidation, and the degradation rate of methyl orange was 82.9% after irradiation for 1 h. The high photocatalytic activity of Nd/TiO2-SiO2 was mainly attributed to the visible light absorption and the separation of photoexcited electrons and holes.
References
[1] WANG Feng, MENG Xiang-fu, WEN Bin, ZHANG Jun-hua, DING Yan-fen, ZHANG Shi-min, ZHAO Jin-cai, LIU Shao-ren, YANG Ming-shu. Preparation and photocatalytic activity of PET/TiO2 hybrid nanofibers via in-situ growth method [J]. Chinese Science Bulletin, 2010, 55(19): 1873-1878. (in Chinese)
[2] TRAPALIS C C, KEIVANIDIS P, KORDAS G, ZAHARESCU M, CRISAN M, SZATVANYI A, GARTNER M. TiO2 (Fe3+) nanostructured thin films with antibacterial properties [J]. Thin Solid Films, 2003, 433(1-2): 186-190.
[3] CHEN Jun-tao, LI Xin-jun, YANG Ying, WANG Liang-yan, HE Ming-xing. Effect of Sm3+ doping on photocatalytic properties of TiO2 thin films [J]. Journal of Catalysis 2004, 25(5): 397-402. (in Chinese)
[4] LIANG Chun-hua, LI Fang-bai, LIU Cheng-shuai, LU Jia-long, WANG Xu-gang. The enhancement of adsorption and photocatalytic activity of rare earth ions doped TiO2 for the degradation of orange I [J]. Dyes Pigments, 2008, 76(2): 477-484.
[5] EL-BAHY Z M, ISMAIL A A, MOHAMED R M. Enhancement of titania by doping rare earth for photodegradation of organic dye (Direct Blue) [J]. J Hazard Mater, 2009, 166(1): 138-143.
[6] KIISK V, SAVEL M, REEDO V, LUKNER A, SILDOS I. Anatase-to-rutile phase transition of samarium-doped TiO2 powder detected via the luminescence of Sm3+ [J]. Phys Procedia, 2009, 2(2): 527-538.
[7] WANG Ru, XU Yue-hua, CHEN Ming-jie, JIA Jin-liang. Effect of erbium doping on photocatalytic properties of TiO2 films on glass surface [J]. The Chinese Journal of Nonferrous Metals, 2009, 19(10): 1866-1871. (in Chinese)
[8] JOSHI U A, CHOI S H, JANG J S, LEE J S. Transesterification of dimethylcarbonate and phenol over silica supported TiO2 and Ti-MCM 41 catalysts: Structure insensitivity [J]. Catal Lett, 2008, 123: 115-122.
[9] XU Xin, WANG Xiao-jing, HU Zhong-hua, LIU Ya-fei, WANG Chen-chen, ZHAO Guo-hua. Influence of sol-gel and dip-hydrothermal preparation methods on structure and performance of TiO2/AC photocatalysts [J]. Acta Physico-Chimica Sinica, 2010, 26(1): 79-86. (in Chinese)
[10] HOUMARD M, RIASSETTO D, ROUSSEL F, BOURGEOIS A, BERTHOME G, JOUD J C, LANGLET M. Enhanced persistence of natural super-hydrophilicity in TiO2-SiO2 composite thin films deposited via a sol-gel route [J]. Surf Sci, 2008, 602: 3364-3374.
[11] XU Jing-jing, AO Yan-hui, FU De-gang, YUAN Chun-wei. Synthesis of fluorine-doped titania-coated activated carbon under low temperature with high photocatalytic activity under visible light [J]. J Phys Chem Solids, 2008, 69(10): 2366-2370.
[12] NGUYEN T V, LEE H C, ALAM KHAN M, YANG O B. Electrodeposition of TiO2/SiO2 nanocomposite for dye-sensitized solar cell [J]. Sol Energy, 2007, 81(4): 529-534.
[13] LI Zhi-jie, HOU Bou, XU Yao, WU Dong, SUN Yu-han. Hydrothermal synthesis, characterization, and photocatalytic performance of silica-modified titanium dioxide nanoparticles [J]. J Colloid Interf Sci, 2005, 288(1): 149-154.
[14] ZHANG Yu-hong, XU Hai-liang, ZHANG Hua-xing, WANG Yan-guang. The effect of lanthanide on the degradation of RB in nanocrystalline Ln/TiO2 aqueous solution [J]. J Photochem Photobiol A, 2005, 170(3): 279-285.
[15] SU Wen-yue, FU Xian-zhi, WEI Ke-mei. Photocatalytic degradation of bromomethane on sol-gel derived titania [J]. Chemical Research in Chinese Universities, 2001, 22(2): 272-275. (in Chinese)
[16] NILCHI A, JANITABAR-DARZI S, MAHJOUB A R, RASOULI-GARMARODI S. New TiO2/SiO2 nanocomposites— Phase transformations and photocatalytic studies [J]. Colloid Surface A, 2010, 361(1-3): 25-30.
[17] XU Y, ZENG Z. The preparation, characterization, and photocatalytic activities of Ce-TiO2/SiO2 [J]. J Mol Catal A, 2008, 279(1): 77-81.
[18] ZHOU Wu-yi, TANG Shao-qiu, ZHANG Shi-ying, ZHOU Xi-rong, WAN Long. Preparation and photocatalytic properties of nano-TiO2 photocatalysis doped with various rare earth [J]. Jounal of the Chinese Ceramic Society, 2004, 32(10): 10-13.
[19] XIE Yi-bing, YUAN Chun-wei. Photocatalysis of neodymium ion modified TiO2 sol under visible light irradiation [J]. Appl Surf Sci, 2004, 221: 17-24.
[20] XIE Yi-bing, YUAN Chun-wei. Visible-light responsive cerium ion modified titania sol and nanocrystallites for X-3B dye photodegradation [J]. Appl Catal B, 2003, 46(2): 251-259.
硅胶负载掺钕TiO2的制备及其光催化性能
杨学灵,朱 丽,杨乐敏,周武艺,徐悦华
华南农业大学 理学院 生物材料研究所,广州 510642
摘 要:采用溶胶-凝胶法将掺钕二氧化钛负载在二氧化硅上制备复合光催化剂(Nd/TiO2-SiO2),利用XRD、SEM、FT-IR和DRS对Nd/TiO2-SiO2进行表征,并通过甲基橙溶液的光催化降解评价其光催化性能。结果表明:Nd/TiO2-SiO2在可见光区388-619 nm范围内的光吸收性能随着钕掺杂量的增大而增强,钕掺杂有利于降低电子-空穴的复合率,钕和二氧化硅提高TiO2的光催化活性。在600 °C煅烧的0.1% Nd/TiO2-SiO2的光催化活性最高,1 h后甲基橙降解率为82.9%。
关键词:二氧化钛;二氧化硅;钕掺杂;光催化;溶胶-凝胶法
(Edited by FANG Jing-hua)
Foundation item: Project(2009B010100001) supported by the Key Academic Program of the 3rd Phase “211 Project”of South China Agricultural University, China; Project(2007B030103019) supported by Guangdong Science and Technology Development Foundation, China
Corresponding author: XU Yue-hua; Tel: +86-20-85281989; E-mail: xuyuehua@scau.edu.cn
DOI: 10.1016/S1003-6326(11)60718-8