
Microstructure and mechanical properties of Mg-Zn-Gd-based alloys strengthened with quasicrystal and Laves phase
LIU Yong(刘勇)1, YUAN Guang-yin(袁广银)1, 2, LU Chen(卢晨)1, DING Wen-jiang(丁文江)1
1. National Engineering Research Center of Light Alloy Net Forming, Shanghai Jiao Tong University,
Shanghai 200030, China;
2. State Key Laboratory of Metal Matrix Composites, Shanghai Jiao Tong University, Shanghai 200030, China
Received 15 July 2007; accepted 10 September 2007
Abstract: One kind of Mg3.5Zn0.6Gd-based alloy strengthened with quasicrystals was designed, and the effect of alloying elements on microstructure and mechanical properties of as-cast Mg-Zn-Gd alloy at room temperature and elevated temperatures were studied. The results indicate that MgZnCu Laves phase, which coexists with quasicrystal at grain boundary, emerges with the addition of copper element in Mg-Zn-Gd alloy. The strength of alloys exhibits the parabola curve with the increase of copper content. The alloy with 1.5% (mole fraction) Cu shows better mechanical properties at room temperature: tensile strength 176 MPa, yield strength 176 MPa and elongation 6.5%. The existence of MgZnCu Laves phase can effectively improve the heat resistance and elevated temperature properties of the alloy. The alloy with 1.5% Cu has better mechanical properties at 200 ℃: tensile strength 130 MPa and elongation 18.5%. The creep test of the alloys at 200 ℃ and 50 MPa for 102 h indicates that Mg3.5Zn0.6Gd alloy reinforced with quasicrystal has better creep properties than AE42, which can be further improved with the introduction of Laves phase in the alloy.
Key words: Mg alloy; Mg-Zn-Gd; Laves phase; quasicrystal; mechanical properties
1 Introduction
As the lightest metallic structural materials, magnesium and its alloys have received increasing attention in the last decade because of their high potential in automotive and aerospace applications[1-2]. Major growth areas in automotive use for Mg alloys are the powertrain applications, such as the transmission case and the engine block[3]. These applications require high elevated temperature performance at 150-200 ℃ and 50-70 MPa. Commercial AM and AZ types of magnesium alloy do not meet such requirement because they are prone to excessive creep deformation when exposed to moderate levels of load at 100-200 ℃[4-5]. In order to improve the elevated temperature performance of Mg alloy, the strengthening phase with high thermal stability must be obtained at the grain boundary. YUAN et al[6-7] have reported that icosahedra quasicrystal phase (I-phase) and Laves phase can act as the effective elevated temperature strengthening phase in cast Mg-based alloys. When an alloy contains quasicrystals as the second phase, they are stable against coarsening at high temperatures due to the low interfacial energy of quasicrystals[8]. Similar to the I-phase, some Laves phases have high hardness, excellent wear and corrosion resistance[9]. During recent decades, Laves phases have been successfully used as a high temperature strengthening phase in some intermetallics[9-10].
However, the combination of quasicrystal and Laves phase must be further optimized in order to obtain the optimum properties for Mg-Zn-Gd-based alloy. In the present work, the effect of alloy element on microstructure and mechanical properties of as-cast Mg-Zn-Gd alloy at room temperature and elevated temperatures was investigated. The composition of Mg-Zn-Gd-based alloy was finally optimized through modifying the combination of the quasicrystal and Laves phase.
2 Experimental
Four alloys with the composition of Mg3.5Zn- 0.6Gd-xCu (x=0, 1.0%, 1.5%, 2%) were designed in this study. It was prepared by induction melting a mixture of high purity Mg, Zn, Gd and Cu in a mixed gas atmosphere of SF6 (1%, volume fraction) and CO2 (99%, volume fraction), and cast into a steel mould. Flat tensile test specimens were prepared by electric spark machining from the cast sample. Tensile tests were performed at room temperature(RT) by the Zwick/Roell Z020 testing machine with a computer interface. Tensile tests at 200 ℃ were performed in a WD-10A testing machine with temperature control system. Samples were heated by an electric resistance furnace and kept at the tensile test temperature for 5 min before performing the test. All tensile tests were performed at a strain rate of 10-3. The creep tests of alloys were conducted in one multi-functional machine of CSS—3902 at 200 ℃ and 50 MPa. Analysis of I-phase and Laves phase was performed by optical microscopy(OM), X-ray diffraction (XRD) with the Cu Kα radiation, scanning electron microscope(SEM) using Philips-Sirion200 attached with energy-dispersive spectroscopy(EDS).
3 Results and discussion
3.1 Effect of Cu element on microstructure and phase of Mg3.5Zn0.6Gd-xCu alloys
The microstructures of Mg3.5Zn0.6Gd-xCu alloys are shown in Fig.1. These alloys have a dendritic cast structure with large intergranular phase. With the addition of Cu element, the microstructure of Mg3.5Zn0.6Gd-xCu alloys was refined, and the content of grain boundary phase also increased, as shown in Figs.1(b)-(d). More equiaxed grains and homogeneous microstructure were obtained for Mg3.5Zn0.6Gd1.5Cu alloy. The XRD patterns of Mg3.5Zn0.6Gd and Mg3.5Zn0.6Gd1.5Cu alloys are shown in Fig.2. Mg3.5Zn0.6Gd alloy is composed of α-Mg and I-phase with a quasilattice parameter of 0.568 nm, which can be calculated by the Elser’s indexing method [11]. For Mg3.5Zn0.6Gd1.5Cu quaternary alloy, the analysis of XRD indicates the existence of C15 Laves phase MgCuZn (Cubic, MgCu2 type), in addition to α-Mg and I-phase.
The SEM images of the Mg3.5Zn0.6Gd and Mg3.5Zn0.6Gd1.5Cu alloys are shown in Fig.3. As shown in Fig.3 (a), the microstructure of Mg3.5Zn0.6Gd alloy has a discontinuous distribution. The micro- structure of Mg3.5Zn0.6Gd1.5Cu alloy has a closed and continuous network as a hard skeleton, as shown in Fig.3(b). The EDS analysis of Fig.3 is shown in Table 1. It can be found that the eutectic phase in Mg3.5Zn0.6Gd alloy is rich in Gd and Zn elements, and the interior of which has higher content of Gd and Zn than the exterior. This means that there exists some degree composition segregation during solidification of alloy. The corresponding TEM image of Mg3.5Zn0.6Gd alloy has been reported in Ref.[7]. The intergranular phase in this ternary alloy has been identified as an icosahedra quasicrystalline phase through the selected area diffraction patterns with five-fold symmetry. Meanwhile, it can also be observed that this five-fold pattern has some degree distortion, which indicates that the I-phase has linear phason strains. It could be ascribed to the composition segregation detected by EDX.
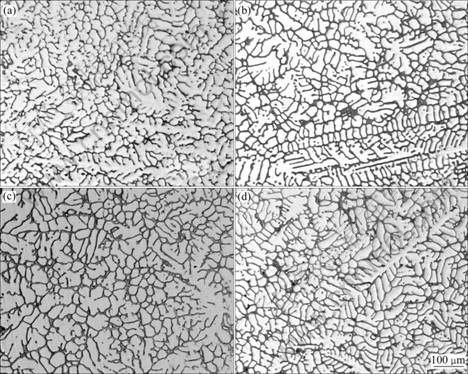
Fig.1 Microstructures of as-cast Mg3.5Zn0.6Gd-xCu alloys: (a) Without Cu; (b)1.0% Cu; (c) 1.5% Cu; (d) 2.0%Cu
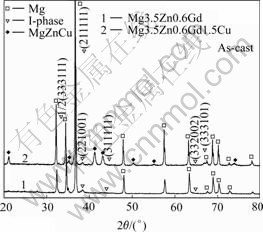
Fig.2 XRD patterns of as-cast alloys
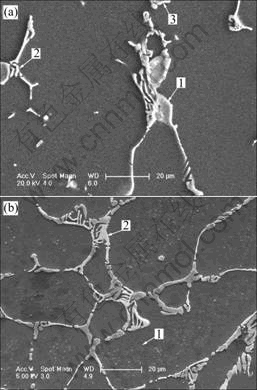
Fig.3 SEM images of as-cast alloys: (a) Mg3.5Zn0.6Gd; (b) Mg3.5Zn0.6Gd1.5Cu
Table 1 EDS analysis of alloys corresponding to SEM images in Fig.3 (mass fraction, %)
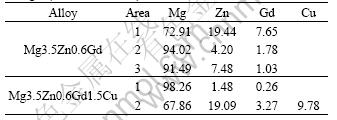
For Mg3.5Zn0.6Gd1.5Cu alloy, the Cu element does not exist in the matrix but in the area of intergranular phase, which means Laves phase coexists with I-phase in this intergranular area. The coexistence of I-phase and C15 Laves phase also has been confirmed by TEM in Ref.[7]. Moreover, the MgZnCu Laves phase has a cubic structure with the lattice parameter of a=0.723 nm, slightly larger than the standard data a=0.717 nm[12]. However, it is difficult to identify the I-phase and the Laves phase from the morphology, unless microanalysis is performed.
3.2 Effect of Cu element on mechanical properties of Mg3.5Zn0.6Gd-xCu alloys
3.2.1 Mechanical properties at RT
The effect of Cu content on the mechanical properties of alloys at RT is shown in Fig.4(a). Mg3.5Zn0.6Gd alloy shows 160 MPa tensile ultimate strength(UTS), 81 MPa yield strength(YS) and 3.7% elongation. After the addition of Cu element, the mechanical properties of alloys are improved to some degree, and show a parabolic curve with the increase of Cu content. When the content of Cu is about 1.5%, the alloy exhibits higher mechanical properties with 176 MPa UTS, 106 MPa YS and 6.5% elongation. With the addition of Cu element, Mg-Zn-Gd-Cu alloy has larger content of intergranular phase and forms a closed and continuous net microstructure due to the formation of Laves phase. The strength of alloys could be improved due to the higher particle strengthening effects.
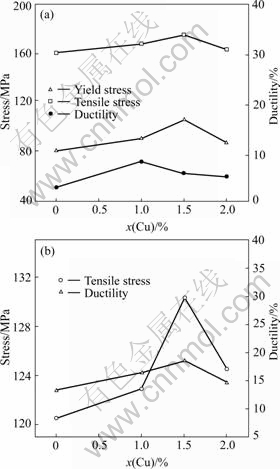
Fig.4 Effects of Cu content on mechanical properties of Mg3.5Zn0.6Gd-xCu alloys: (a) RT; (b) 200 ℃
3.2.2 Mechanical properties at elevated temperatures
The effects of Cu content on mechanical properties of alloys at elevated temperatures are shown in Fig.4(b). It also exhibits a parabolic tendency similar to that of alloy at RT. Alloy containing 1.5% Cu exhibits higher mechanical properties at elevated temperature, 130 MPa UTS and 18.5% elongation. This attributes to the higher thermal stability of alloy with MgZnCu Laves phase and I-phase. The combination of MgZnCu Laves phase and I-phase could effectively embarrass the softening of Mg alloy, and provide higher grain boundary pining effect[13]. YUAN et al[7] have tested the hardness of Mg3.5Zn0.6Gd and Mg3.5Zn0.6Gd1.5Cu alloys at elevated temperature. The Mg3.5Zn0.6Gd1.5Cu alloy exhibits higher hardness and lower softening rate than Mg3.5Zn0.6Gd alloy.
With further increasing the Cu content, it will result in higher volume fraction of Laves phase and lower content of I-phase in Mg-Zn-Gd-Cu alloy. However, Laves phase has low symmetry and topology closed-pack crystal structure[14], which makes it become cleave easily and brittle; while the I-phase has a very high symmetry and is isotropic in nature [15]. Thus, the mechanical properties of the Mg-Zn-Gd-Cu alloy will be deteriorated when the Cu content exceeds 1.5%.
3.2.3 Creep properties of alloys
Fig.5 shows the tensile creep curves of the Mg3.5Zn0.6Gd and Mg3.5Zn0.6Gd1.5Cu alloys at 200℃ and 50 MPa. As shown in Fig.5, the alloys studied, like most other materials or alloys tested under the tensile creep mode, exhibit two stages of creep within a limited test time, i.e. primary creep or transient creep and secondary or steady-state creep. For comparison, the data of AE42 was also recorded from Ref.[16]. The creep results are summarized in Table 2. It is seen that the Mg3.5Zn0.6Gd alloy containing I-phase shows significant improvements over commercial heat-resistant magnesium alloy AE42 in both total creep strain and steady-state creep rate. The steady-state creep rate
for the AE42 alloy is 4.34×10-8 s-1 and the creep strain after 102 h is 2.75%, while those for the Mg3.5Zn0.6Gd alloy are significantly reduced to 4.72×10-9 s-1 and 0.294%, respectively, almost reduced by one order of magnitude. These results indicate that I-phase plays an important role in the enhancement of creep resistance for Mg alloys at elevated temperatures. This means that grain boundary softening and gliding during creep test could be restrained by I-phase due to its low interface energy. After the addition of Cu element, the creep resistance of the Mg3.5Zn0.6Gd1.5Cu alloy can be further improved, the steady-state creep rate
and the creep strain after 102 h are 1.9×10-9 s-1 and 0.084%, respectively. This also means that the high temperature creep properties of the Mg-Zn-Gd-Cu alloy simultaneously containing I-phase and Laves phase are better than those of the Mg-Zn-Gd alloy reinforced with single I-phase.
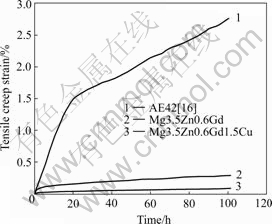
Fig.5 Creep strain vs time of alloys under conditions of 200 ℃ and 50 MPa for 102 h
Table 2 Creep strain ε and creep rate
of alloys under conditions of 200 ℃ and 50 MPa after 102 h
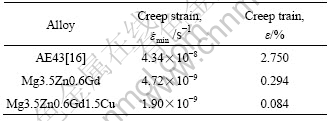
4 Conclusions
1) Mg3.5Zn0.6Gd alloy contains icosahedral quasicrystal phase. After the addition of Cu element, there forms one Laves phase MgZnCu with MgCu2 type coexisting with I-phase in the intergranular area.
2) The mechanical properties at RT and elevated temperatures of Mg3.5Zn0.6Gd-xCu alloy show a parabolic curve with the increase of Cu content. Mg3.5Zn0.6Gd1.5Cu alloy shows higher mechanical properties at RT with 176 MPa UTS, 106 MPa YS and 6.5% elongation. The formation of MgZnCu Laves phase can effectively improve the properties of alloy at elevated temperatures. The Mg3.5Zn0.6Gd1.5Cu alloy has higher mechanical properties at elevated temperatures with 130 MPa UTS and 18.5% elongation.
3) I-phase plays an important role in the improvement of creep resistance of Mg alloys, the combination of I-phase and Laves phase can further improve the creep resistance of Mg alloys. At 200 ℃ and 50 MPa, the steady-state creep rate and the creep strain after 102 h of the Mg3.5Zn0.6Gd1.5Cu alloy are 1.9×10-9 s-1 and 0.084%, respectively.
References
[1] SCHUMANN S, FRIEDRICH H. Current and future use of magnesium in the automobile industry [J]. Mater Sci Froum, 2003, 419/422(1): 51-56.
[2] MORDIKE B L, KAINER K U. Proc Magensium Alloys and Their Applications [C]. Frankfurt: Werkstoff-Informationsgesell Schaft, 1998: 123-126.
[3] LUO A A. Current and future use of magnesium in the automobile industry [J]. Mater Sci Forum, 2003, 419/422(1): 57-66.
[4] POLMEAR I J. Light alloys: Metallurgy of the light metals [M]. 3rd ed. London: Arnold, 1995: 256-278.
[5] HUMBLE P. Towards a cheap creep resistant magnesium alloy [J]. Mater Forum, 1997, 21: 45-56.
[6] YUAN G Y, AMIYA K, KATO H, INOUE A. Structure and mechanical properties of cast quasicrystal-reinforced Mg-Zn-Al-Y base alloys [J]. J Mater Res, 2004, 19: 1531-37.
[7] YUAN G Y, KATO H, AMIYA K, INOUE A. Excellent creep properties of Mg-Zn-Cu-Gd-based alloy strengthened by quasicrystals and Laves phases [J]. J Mater Research, 2005, 20(5): 1278-86.
[8] PIERCE F S, POON S J, GUO Q. Electron localization in metallic quasicrystals [J]. Science, 1993, 261: 737-39.
[9] HALSTEAD A, RAWLINGS R D. The fracture behavior of two Co-Mo-Cr-Si wear resistant alloys [J]. J Mater Sci, 1985, 20: 1248-56.
[10] WASIKOWSKA A, BARTSCH M, STEIN F, PALM M, SAUTHOFF G, MESSERSCHMIDT U. Plastic deformation of Fe-Al polycrystals strengthened with Zr-containing Laves phases (II): Mechanical properties [J]. Mater Sci Eng A, 2004, 381: 1-15.
[11] ELSER V. Indexing problems in quasicrystal diffraction [J]. Phys Rev B, 1985, 32: 4892-8.
[12] ASTM X-Ray Powder Diffraction Data File [S]. 65-7003.
[13] LIU Y, YUAN G Y, LU C, DING W J. Deformation behavior of Mg-Zn-Gd-based alloys reinforced with quasicrystal and Laves phases at elevated temperatures [J]. J Alloys Compd, 2007, 427: 160-165.
[14] LIU C T, TORTORELLI P F, HORTON J A, CARMICHAEL C A. Effects of alloy additions on the microstructure and properties of Cr-Cr2Nb alloys [J]. Materials Science and Engineering A, 1996, 214: 23-32.
[15] JANOT C. Quasicrystals [M]. Clarendon Press: Oxford, 1994.
[16] HUANG X F, WANG Q D, LIU L F. Effect of RE on the microstructure and properties of Mg-5Al-1Si alloy [J]. Rare Metal Materials and Engineering, 2005, 34(5): 795-798. (in Chinese)
Foundation item: Project(50501013) supported by the National Natural Science Foundation of China; Project(05JC14023) supported by the Science and Technology Committee of Shanghai City, China
Corresponding author: YUAN Guang-yin; Tel: +86-21-62932508; E-mail: gyyuan@sjtu.edu.cn
(Edited by PENG Chao-qun)