
Effects of solution heat treatment on corrosion resistance of 5083F Al alloy
Seong-Jong KIM, Seok-Ki JANG
Division of Marine Engineering, Mokpo Maritime University, Mokpo City, Jeonnam, 530-729, Korea
Received 18 June 2008; accepted 10 March 2009
Abstract: Aluminum alloys are used as substitutes for fiber reinforced polymer(FRP) in ships and boats. However, ships constructed with 5000-series Al alloy suffer a little corrosion in the marine environment, when they run in high speed, and high flow rate, etc. Therefore, solution heat treatment was carried out to prevent corrosion. The optimal heat treatment involved heating specimens for 120 min at 420 ℃ and then cooling them in water. In addition, the optimal ageing condition involved ageing specimens for 240 min at 180 ℃. The slow strain rate test(SSRT) in a seawater revealed that heat treatment under optimal conditions produced improved elongation, time-to-fracture and amount of dimples compared with the as-received specimen.
Key words: aluminum alloy; seawater; solution heat treatment
1 Introduction
The fiber reinforced polymer(FRP) ships, including small fishing boats, have many environmental and recycling problems, and there is no method to decommission an FRP ship. In addition, medium and large ships cannot detect these FRP and wooden vessels, since the ability of composite materials to reflect radar waves is poor[1-2]. Therefore, a large proportion of ship accidents involved. If aluminum was used as a substitute for FRP in ships and boats, the result would be more environmentally friendly vessels that are easy to recycle and have the added value of reduced fuel consumption, higher speed, and increased load capacity [3-4]. Ships constructed with 5000-series Al alloy suffer a little corrosion in the marine environment[1, 5-7], when they run in high speed, high flow rate etc. In this work, the solution heat treatment was carried out to prevent corrosion for Al alloy(5083F) specimen.
2 Experimental
In the electrochemical experiments, the specimen was mounted in an epoxy resin so as to leave an exposed area of 100 mm2 that was polished with 600# emery paper. The electrochemical apparatus consisted of a Pt coil as the counter electrode and an Ag/AgCl reference electrode. A scanning rate of 2 mV/s was applied in natural seawater. Anodic polarization experiments were executed from an open-circuit potential(OCP) to a range from -0.5 to 3.0 V. Cathodic polarization experiments were undertaken from OCP to -2.0 V. The hardness measurement was executed by micro-Vickers hardness tester with applied load of 9.807 N, and keeping time of 10 s. The slow strain rate test(SSRT) specimen was immersed in seawater using an Instron instrument (#8516) of 10 t capacity. The size of test specimens is 235 mm×4 mm×6 mm. Notches, 1 mm wide and deep, were made on both sides of parallel parts of the specimens to cause a fracture. The SSRT test was carried out at a strain rate of 0.002 mm/min.
3 Results and discussion
Fig.1 shows the hardness of specimens artificially aged at 180 ℃ after being heat treated for 60 min at 420 ℃ and then cooled in water. Each hardness measurement was repeated several times, and the black circles indicate the mean values. The hardness of the un-heat-treated specimen was HV80.72. The greatest hardness was produced after aging of 120 min (HV 88.16) and the second-highest value (HV86.02) was produced after ageing for 240 min. A tendency to cathodic polarization was observed in specimens aged at 180 ℃ after they were heated at 420 ℃ for 60 min and then cooled in water. The heat-treated specimens had a lower current density at cathodic polarization than the un-heat- treated specimen.
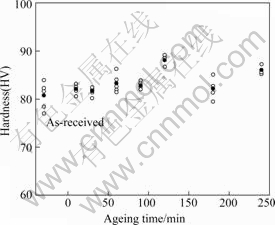
Fig.1 Hardness of specimen aged at 180 ℃ after heat treatment for 60 min at 420 ℃
The polarization trends in all specimens revealed the effects of concentration polarization due to oxygen reduction and activation polarization due to hydrogen generation[8-13].
Fig.2 presents the potential at the turning point for specimens aged at 180 ℃ after being heated for 60 min at 420 ℃ and then cooled in water. ‘Turning point’ means the potential between concentration polarization and activation polarization[5]. Here, the turning point is an important factor for evaluating hydrogen embrittlement. The cathodic protection potential is the best with a high potential compared with the turning point, as well as the region of dissolved oxygen reduction reaction. Higher values indicate strong resistance to hydrogen embrittlement during corrosion protection, since the turning point has a low potential.
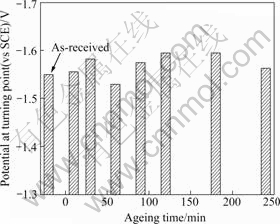
Fig.2 Potential at turning point with ageing time at 180 ℃
As shown in Fig.2, the un-heat-treated specimens had a higher potential at ‘turning point’ than the heat- treated specimens, with the exception of specimens aged for 60 min. Specimens aged for 120 and 180 min had the lowest negative potential at the turning point. Therefore, specimens aged for 120 and 180 min were more resistant to hydrogen embrittlement.
Fig.3 displays the current density at a potential of -1.1 V for specimens aged at 180 ℃ after being heated for 60 min at 420 ℃ and then cooled in water. The current density at a potential of -1.1 V is the cathodic protection range, i.e. the region of concentration polarization resulting from the dissolved oxygen reduction reaction[2]. Hence, a low current density indicates high resistance to corrosion. The anti-corrosion property is improved by heat treatment, since the current densities of heat-treated specimens were lower than those of the un-heat-treated specimen. When the electro- chemical properties were tested in seawater, specimens aged for 240 min at 180 ℃ had the lowest current density, and were therefore the most stable. The anodic polarization of specimens aged at 180 ℃ after being heated for 60 min at 420 ℃ and then cooled in water followed a similar trend, and the current densities at a potential greater than -0.4 V were almost identical. Therefore, it had little effect on stress corrosion cracking properties.
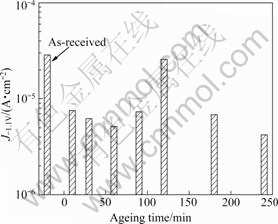
Fig.3 Current density at potential of -1.1 V with ageing time at 180 ℃
Fig.4 compares the corrosion current density of specimens aged at 180 ℃ after being heated for 60 min at 420 ℃ and then cooled in water. The corrosion current density was calculated using Tafel analysis with anodic and cathodic polarization curves around the OCP. Resistance to corrosion is greater for low bar graph values, since the corrosion rate is low.
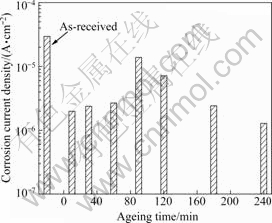
Fig.4 Corrosion current density of specimen with ageing time at 180 ℃
Therefore, heat treatment improved the anti- corrosion properties because it lowered the corrosion current density. The least corrosion current density appeared after specimens were aged for 240 min. The comparison of the various characteristics achieved with artificial ageing revealed that an aging time of 120 min produced the greatest hardness. The un-heat-treated specimen was the least hard. Artificial ageing resulted in the hardening of the 5083F alloy. Artificial ageing for 240 min produced an excellent corrosion current density and a current density at potential of -1.1 V. The comparison of various properties revealed that 240 min was the optimal time of heat treatment. The comparison revealed that artificial ageing for 240 min at 180 ℃ after heating at 420 ℃ for 60 min and then cooling in water yielded excellent results.
Fig.5 presents the hardness of specimen with heat treatment time at 420 ℃. Subsequently, the specimens were aged for 240 min at 180 ℃ and then cooled in water. The un-heat-treated specimens had lower hardness values than all of the heat-treated specimens. In general, heat treatment resulted in precipitation strengthening of the Al alloy[3]. Although precipitation strengthening does not occur in 1000, 3000, 4000, and 5000 series Al alloys, this study verified that heat treatment and artificial ageing treatment strengthened 5083F[14-15]. The greatest hardness was produced with a heat treatment time of 360 min (HV89.08). Treating specimens for longer than 360 min did not result in any improvement over the specimens treated for 30-360 min. The cathodic polarization trends were analyzed in specimens aged for 240 min at 180 ℃ after being heated at 420 ℃ and then cooled in water.
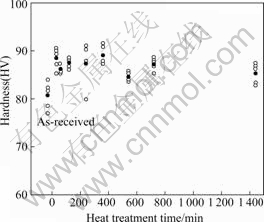
Fig.5 Hardness with heat treatment time at 420 ℃
Fig.6 presents the potential at the turning point with different heat treatment time at 420 ℃. Specimens were then aged for 240 min at 180 ℃ and then cooled in water. The specimens heated for 30-240 min had a greater negative potential at the turning point compared with the un-heat-treated specimen, and the specimens heated for longer than 540 min exhibited noble potential. These results indicated that 240 min is a reasonable heating time limit for corrosion protection during cathodic protection.
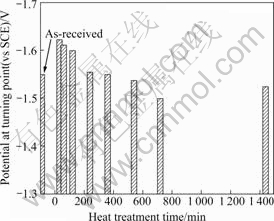
Fig.6 Potential at turning point with heat treatment time at 420 ℃
Fig.7 plots the current density at a potential of -1.1 V with the time of heat treatment at 420 ℃. The specimens were then aged for 240 min at 180 ℃ and then cooled in water. Heat treatment improved the anti-corrosion property of the specimen compared with the non-heat-treated specimen, since the heat-treated specimen had a low current density. The most stable property in seawater was seen after heat treatment for 120 min at 420 ℃, since specimen had the lowest current density. In addition, heat treatment lowered the corrosion current density in the anodic polarization curve. Artificial ageing produced similar, not higher, values of the current density at potentials above -0.4 V. Therefore, stress corrosion cracking characteristics have little effect.
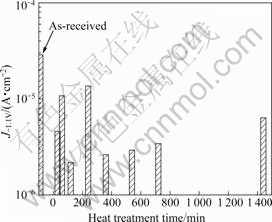
Fig.7 Current density of specimen at potential of -1.1 V with heat treatment time at 420 ℃
Fig.8 shows the corrosion current density with the heat treatment time at 420 ℃. The specimens were aged for 240 min at 180 ℃ and then cooled in water. The highest corrosion current density in the un-heat-treated specimen was 3×10-5 A/cm2. The best corrosion current density values were produced after heat treatment for 30 min (2×10-6 A/cm2). In general, heat treatment improved the anti-corrosion property. A comparison of hardness and the cathodic and anodic polarization curves revealed that the optimal conditions were heat treatment for 120 min at 420 ℃, followed by cooling in water and artificial ageing for 240 min at 180 ℃.
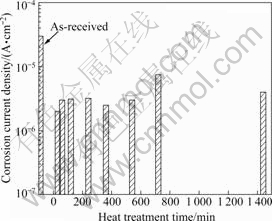
Fig.8 Corrosion current density of specimen with heat treatment time at 420 ℃
Table 1 presents the effects of heat treatment on properties calculated using a SSRT for heat-treated and un-heat-treated specimens in seawater. The maximum tensile strength was similar regardless of the heat treatment condition. However, heat treatment improved elongation and the time-to-fracture by approximately 21% compared with the un-heat-treated specimen. Therefore, heat treatment increased the anti-corrosion property and hardness.
Table 1 Effect of solution heat treatment on properties of specimens by SSRT in seawater
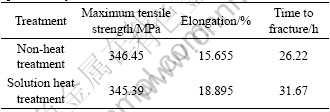
Fig.9 shows SEM photographs of the fractured surfaces of heat-treated and un-heat-treated specimens subjected to the SSRT. The dimple-type surface shows the typical surface morphology of fractured Al. The fractured surface of the un-heat-treated specimen reveals a partial quasi-cleavage fracture mode, which is not seen in the heat-treated specimen, but the latter shows a remarkably increased shear lip, which indicates increased toughness. Heat treatment reduced the strength of the specimens, but increased the elongation and the time-to- fracture[16].
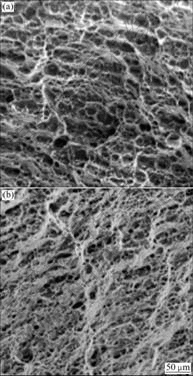
Fig.9 SEM photographs of fractured surface after SSRT: (a) As-received; (b) Solution heat treated
4 Conclusions
The comparison of the various characteristics of 5083F alloy achieved with artificial ageing revealed that an ageing time of 120 min produced the greatest hardness. The un-heat-treated specimen was the least hard. Artificial ageing resulted in hardening of the 5083F alloy. The comparison of hardness and the cathodic and anodic polarization curves revealed that the optimal conditions were heat treatment for 120 min at 420 ℃, followed by cooling in water and artificial ageing for 240 min at 180 ℃. Heat treatment reduced the strength of specimens, but increased the elongation and the time-to- fracture.
References
[1] KIM S J, KO J Y. Investigation on optimum protection potential of high-strength Al alloy(5456-H116) for application in ships [J]. The Korean Journal of Marine Engineering, 2006, 30(1): 157-169.
[2] KIM S J. Evaluating the electrochemical properties in the protection potential of material for use in Al vessels in seawater [J]. Materials Science Forum, 2006, 510/511: 158-161.
[3] KIM S J, KO J Y. Investigation on annealing treatment affecting electrochemical characteristics of Al-Mg alloy[C]//International Welding/Joining Conference-2007. 2007: 207-208.
[4] KIM S J, KO J Y. The electrochemical investigation on corrosion protection potential in anodic and cathodic protection application of Al-Mg alloy[C]//International Welding/Joining Conference-2007. 2007: 194-195.
[5] KIM S J, KO J Y, HAN M S. Evaluation of the characteristics using slow strain rate tests of 5456 Al-Mg alloy for ship construction [J]. The Korean Journal of Chemical Engineering, 2006, 23(6): 1028- 1033.
[6] KIM S J, JANG S K. A slow strain rate test experiment to evaluate the characteristics of high-strength Al-Mg alloy for application in ships [J]. Materials Science Forum, 2006, 510/511: 162-165.
[7] WANG X F, WU X Q, LIN J G, MA M. The influence of heat treatment on the corrosion behaviour of as-spun amorphous Al88Ni6La6 alloy in 0.01 M NaCl solution [J]. Materials Letters, 2007, 61(8/9): 1715-1717.
[8] KIM S J, KIM J I, JANG S K. Electrochemical property and optimum cathodic protection potential of welded high strength steel in natural sea water solution [C]//6th Japan-Korea Symposium on Materials & Interfaces. Beppu, Japan, 2004: 129.
[9] KIM S J, KIM J K, MOON K M. A electrochemical property study on the corrosion behavior of welding part of RE36 steel for marine structure [J]. Journal of Korean Welding Society, 2000, 18(5): 610- 616.
[10] KIM S J, PARK T W, SHIM I O, KIM J H, KIM Y S, MOON K M. A study of hydrogen embrittlement limit potential of Cu-containing high strength low alloy steel for marine structure by potentiostatic SSRT method [J]. Journal of Korean Welding Society, 2001, 19(2): 45.
[11] KIM S J, MOON K M. The relationship between corrosion protection and hydrogen embrittlement properties of HAZ in flux cored arc welding [J]. Metals and Materials International, 2002, 8(4): 387-393.
[12] KIM S J, OKIDO M, MOON K M. An electrochemical study of cathodic protection of steel used for marine structures [J]. The Korean Journal of Chemical Engineering, 2003, 20(3): 560-565.
[13] KIM S J, JANG S K, KIM J I. Electrochemical study of hydrogen embrittlement and optimum cathodic protection potential of welded high strength steel [J]. Metals and Materials International, 2005, 11(1): 63-69.
[14] JUNG K, CONRAD H. External electric field applied during solution heat treatment of the Al-Mg-Si alloy AA6022 [J]. Journal of Materials Science, 2004, 39(21): 6483-6486.
[15] GARRETT R P, LIN J, DEAN T A. An investigation of the effects of solution heat treatment on mechanical properties for AA 6xxx alloys: Experimentation and modeling [J]. International Journal of Plasticity, 2005, 21(8): 1640-1657.
[16] MOUSTAFA M A, SAMUEL F H, DOTY H W. Effect of solution heat treatment and additives on the microstructure of Al-Si automotive alloys [J]. Materials Science Forum, 2004, 467/470(1): 399-406.
Corresponding author: Seong-Jong KIM; Tel: +82-61-2407226; E-mail: ksj@mmu.ac.kr
DOI: 10.1016/S1003-6326(08)60371-4
(Edited by YUAN Sai-qian)