J. Cent. South Univ. Technol. (2011) 18: 1646-1653
DOI: 10.1007/s11771-011-0884-2
Geochemistry of rare earth elements in groundwater from deep seated limestone aquifer in Renlou Coal Mine, Anhui Province, China
SUN Lin-hua(孙林华)1, GUI He-rong(桂和荣)1, CHEN Lu-wang(陈陆望)2, CHEN Song(陈松)1
1. School of Earth Sciences and Engineering, Suzhou University, Suzhou 234000, China;
2. School of Resources and Environmental Engineering, Hefei University of Technology, Hefei 230009, China
? Central South University Press and Springer-Verlag Berlin Heidelberg 2011
Abstract: Rare earth element (REE) concentrations were measured by ICP-MS for groundwater collected from deep seated Taiyuan Fm limestone aquifer (from -400 to -530 m) in Renlou Coal Mine, northern Anhui Province, China. It can be concluded that the groundwater is warm (34.0-37.2 °C) Cl-Ca, Na type water with circum-neutral pH (7.35-8.28) and high total dissolved solids (TDS, 1 746-2 849 mg/L). The groundwater exhibits heavy REEs enrichment relative to light REEs compared with Post Archean Average Shale (PAAS), as well as their aquifer rocks (limestone). The enrichment of REEs is considered to be controlled by terrigeneous materials (e.g. zircon) in aquifer rocks, whereas the fractionation of REEs is controlled by marine derived materials (e.g. calcite), to a less extent, terrigeneous materials and inorganic complexation. The Ce anomalies normalized to PAAS and aquifer rocks are weak, which probably reflects the signature of the aquifer rock rather than redox conditions or pH. The similarities of REE patterns between groundwater and aquifer rocks imply that aquifer rocks play important roles in controlling the REE characteristics of groundwater, and then provide a probability for discrimination of groundwater sources by using REEs.
Key words: rare earth elements; geochemistry; water-rock interaction; limestone aquifer; groundwater
1 Introduction
Because of their unique characteristics [1], rare earth elements (REEs) were highly interested by many geologists and thus had been used in studying many geological processes: magma generation, crustal evolution, weathering process, provenance of sedimentation and water–rock interactions etc [2-6]. However, for the REE study of water systems, much attention had been paid towards the surface water geochemistry of REEs, with less study focused on REEs in groundwater systems although the number of such investigations is increasing [7-11]. Especially, because of the difficulty of sampling, the similar work related to the deep seated environment is still far from understanding.
The previous work revealed that REE abundances and their characteristics (Ce and Eu anomalies, fractionation of light and heavy REEs etc) in groundwater systems were mainly controlled by the rocks through which they flowed over, pH, redox conditions, solution chemistry, organic and/or inorganic complexation, colloidal and particulate matter transport [9]. The most significant finding among these studies is the similarity between groundwater and aquifer rock REE patterns. Indeed, many groundwater samples exhibit REE patterns that closely resemble the REE patterns of the rocks through which they flow. However, for some of the groundwater samples, they have highly fractionated REE patterns compared with the aquifer rocks [11].
The deep coal mining in northern Anhui Province, China, provides us opportunities to trace REE characteristics of groundwater in deep seated environment. Additionally, because the discrimination of water source is important in the safety of coal mine production, a series of studies based on major ions, isotopic and trace elements had been published in previous literatures [12-15]. However, the method related to REEs had not been reported yet, which might be useful in water source discrimination because of 1) the relative stability of REEs during water-rock interactions, 2) the similarity of REE patterns between groundwater and aquifer rock, and 3) different rock compositions of the aquifers.
In this work, the preliminary results of REE concentrations, distribution patterns, as well as inorganic speciation modelling of the groundwater from limestone aquifer (LW) located between -400 and -530 m in Renlou Coal Mine, Anhui Province, China, are reported, and the controlling factors for the REE concentration, fractionation and Ce anomaly are discussed based on the comparison of REEs of groundwater, average shale and aquifer rocks. It will provide additional information about the water-rock interactions in deep seated environment, and provide new information for water source discrimination by using REEs.
2 Hydrogeological setting
The Renlou Coal Mine is located southwest to Suzhou City, northern Anhui Province, China (Fig.1), which belongs to the northeastern part of the Huaibei mining area. The Renlou Coal Mine is separated by the north Jiegou Fault and south Xuting Fault from Suntuan and Xutuan coal mines in north and south, respectively. The length of Renlou Coal Mine from north to south is 9.8-14 km, and the width from east to west is 1.2- 3.5 km with a total area of 43 km2. The depth of basement rock in the mine increases from north to south significantly, about -200 m and -280 m below the surface, respectively. The basement of Renlou Coal Mine is composed of Archean and early to middle Proterozoic metamorphic rocks, with stable sedimentation between late-Proterozoic and Permian. The total thickness is nearly 3 000 m with lack of sedimentation between late-Ordovician to Triassic.
The groundwater system in the region is subdivided into four aquifers from shallow to deep: the Quaternary aquifer, the Coal bearing aquifer (Meixi aquifer in the previous study [12]), the Taiyuan Fm Aquifer and the Ordovician limestone aquifer. The characteristics of each aquifer synthesized according to the previous work [12] are listed in Table 1. Hydrodynamic conditions of these four aquifers are different based on carbon and oxygen isotopic study [13]. Due to shallow depth and coal mining, Quaternary aquifer is an “open” system; Coal bearing aquifer is relatively “closed” with only small amount of groundwater in it, which can be recharged by the upper Quaternary and lower Taiyuan Fm Aquifer (Fig.2); the limestone aquifers (including Taiyuan Fm and the Ordovician limestone aquifer) are also closed systems, whereas the groundwater in them flows quickly.
3 Methods
The groundwater from Taiyuan Fm limestone aquifer was focused on; aquifer rocks were collected from drilling cores; water samples were collected via drainage holes in the alley. Water temperature (T), pH and total dissolved solids (TDS) were measured in the field with a portable pH and TDS meter. Water samples were filtered through 0.45 μm pore-size membrane and collected into polyethylene bottles that had been cleaned using trace element clean procedures. Two bottles of water (2.0 L for analysis of major ions, and 0.5 L for REE analysis) were collected for each sample. The samples for REE analysis were immediately acidified to less than pH 2 using high-purity HNO3 and stored at <4 °C before analysis.
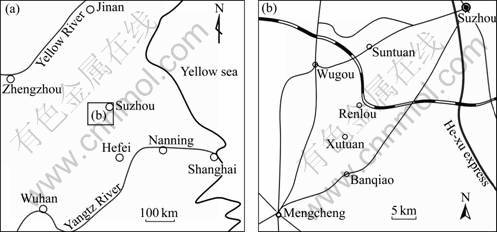
Fig.1 Location map of study area (Renlou Coal Mine) in northern Anhui Province, China
Table 1 Hydrological characteristics of aquifers in Renlou Coal Mine [12]
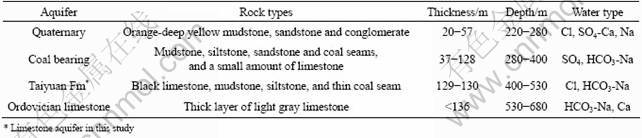
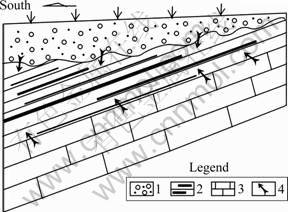
Fig.2 Cross section of Renlou Coal Mine: 1—Quaternary sediments; 2—Coal bearing strata; 3—Carbonatic strata (Taiyuan Fm and Ordovician); 4—Direction of underground water recharge
Major ions were analyzed in the Department of Geology, Renlou Coal Mine. REE concentrations of groundwater were determined after pre-concentration by liquid-liquid extraction, and analyzed by inductively coupled plasma mass spectrometry (ICP-MS, POEMS III) in the State Key Laboratory of Geological Processes and Mineral Resources, China University of Geosciences (Wuhan). Analytical process followed the method reported by SHABANI et al [16]. The isobaric interference of BaO to Eu had not been well corrected, leading to the useless Eu among these REEs [11]. The analytical precision for all REEs except for Eu was 10% (relative standard deviation) or better. REEs of rocks were analyzed with PE Elan 6000 ICP-MS at the Key Laboratory of Isotope Geochronology and Geochemistry, CAS. The relative derivations were less than 5%, and detailed analytical procedures were referred to LIU et al [17].
The previous study demonstrated that REEs exist in solution primarily as complexes with halides, sulphate, phosphate, hydroxides, and carbonate or as the free ions [18]. Phosphate complexes are only important if phosphrous levels are high [19], which is always considered in eutrophic water rather than water in this study. Moreover, organic complexation of REEs in groundwater is likely to be substantially less significant than for surface water [11]. In this study, only inorganic complexation of REEs modeled by Visual Minteq (Version 2.61) is reported. The method of modelling is similar to that used by ZHU et al [19] and RONNBACK et al [20].
4 Results
4.1 Major solute compositions of groundwater
Concentrations of the major ions of the LW are presented in Table 2, along with pH, water temperatures and TDS. The groundwater can be classified as Cl-Ca, Na water based on the relative distribution of the major solutes (Fig.3), which is slightly different from the previous study [12]. Additionally, the groundwater is of circum-neutral pH, with values ranging from 7.35 to 8.28 and an average of 7.62, and is relatively warm with temperatures ranging from 34.0 °C to 37.2 °C and an average of 35.7 °C. The groundwater is also high in TDS ranging from 1 746 to 2 849 mg/L with an average of 2 241 mg/L.
4.2 REE of groundwater
The REE concentrations are slightly higher than those reported for other groundwater of circum-neutral pH (see Table 3). For example, Nd concentrations of the LW range from 0.006 to 0.010 μg/L with average of 0.008 μg/L (equivalent to 55.5 pmol/kg). In comparison, Nd concentration of the LW is higher than the mean Nd concentration of groundwater collected from the Battleford aquitard (pH=7.54 with mean Nd concentration of 22 pmol/kg) [21]. On the other hand, groundwater from the Carnmenellis region of England (pH=5.82) has remarkably high Nd concentrations ranging from 3 500 to as high as 478 000 pmol/kg [7].
Table 2 Major solute compositions, pH, temperature and total dissolved solids (TDS) of groundwater from limestone aquifer
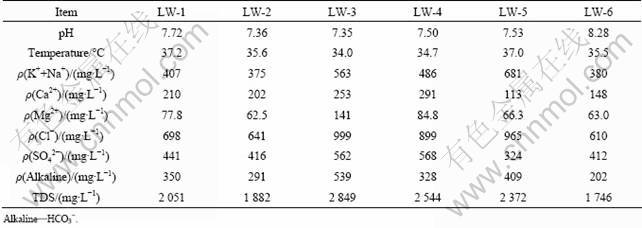
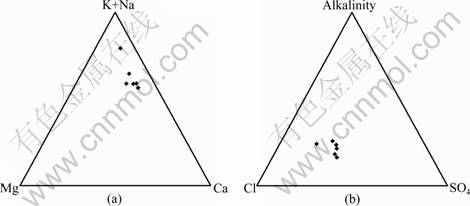
Fig.3 Ternary plots of major ion concentrations of groundwater from limestone aquifer
Table 3 Rare earth element concentrations of groundwater from limestone aquifer (μg/L)
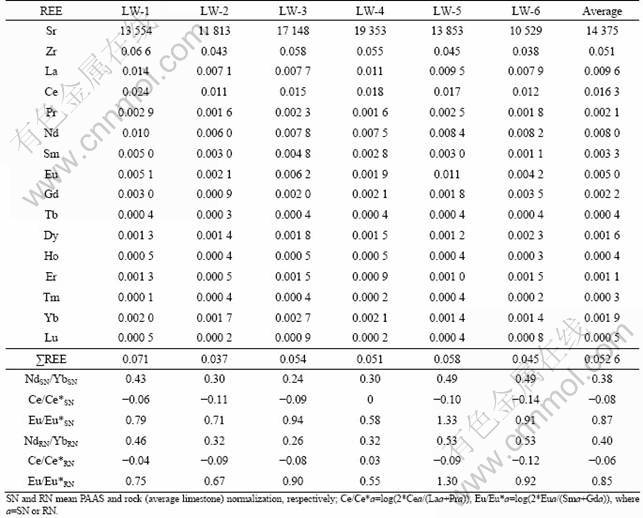
PAAS (Post Archean Average Shale [3])-normalized REE patterns of LW are presented in Fig.4. All kinds of the groundwater are characterized by enrichment of HREEs compared with LREEs when normalized to PAAS, and the normalized NdSN/YbSN ratios (SN means PAAS normalization) range from 0.24 to 0.49 with average of 0.38 (Table 3). In addition, all kinds of the groundwater samples show weak or no negative Ce anomalies (Fig.4). The PAAS-normalized Ce anomalies range from -0.14 to 0 with average of -0.08. Another remarkable characteristic is their positive Eu anomalies, Eu/Eu*SN=0.58-1.33. Moreover, correlation analysis indicates that the Zr concentrations of groundwater are well correlated with ∑REE (R=0.76), whereas the R value between Sr and ∑REE is 0.22.
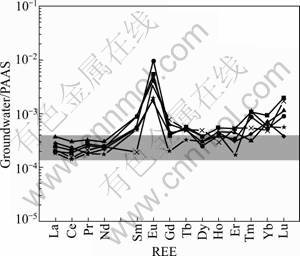
Fig.4 PAAS normalized REE patterns of groundwater from limestone aquifer
4.3 REE of limestones and limestone-normalized patterns of groundwater
The REE concentrations of limestones are listed in Table 4, and the PAAS-normalized REE patterns are presented in Fig.5. Some of these limestones exhibit PAAS-normalized REE patterns similar to the oceanic limestones [22-23] with HREEs enriched relative to LREEs (NdSN/YbSN=0.60-0.88). However, other two samples (LR-4 and 6) have NdSN/YbSN=1.03 and 1.11, respectively. Moreover, these limestones show almost no negative Ce anomalies, with Ce/Ce*SN ranging from -0.07 to -0.01 with an average of -0.02. Such characteristics suggest that these limestones are not “pure” limestones, but have been affected by terrigenous materials (“pure” marine derived limestones are characterized by significant negative Ce anomalies and low NdSN/YbSN ratios [22-23]).
Zr and Sr are always considered to represent terrigenous and marine materials, respectively. The Zr concentrations of limestones are well correlated with ∑REE (R=0.96), whereas the R value between Sr and ∑REE is 0.
Although it is instructive to normalize REE concentrations of the groundwater in this study to PAAS for purposes of comparison with earlier studies, it is more appropriate to normalize these groundwater to the REE concentrations of the limestones in this study because aquifer rocks are thought to be the chief source of REEs to groundwater [7]. Figure 6 shows a plot of REE concentrations of LW-normalized to the average limestones from Table 4. The average limestone normalized groundwater patterns are similar to the PAAS normalized patterns with little differences. For example, the groundwater also exhibits HREEs enrichment relative to LREEs with NdRN/YbRN=0.26-0.53 (average of 0.40, RN means rock normalized), and shows weak to no Ce anomalies (Ce/Ce*RN=-0.12-0.03 with average of -0.06). Additionally, the Eu/Eu*RN ranges from 0.55 to 1.30 with average of 0.85. It is worth noting that the fractionation of REEs of groundwater normalized to PAAS and average limestone are different (e.g. the fractionation of La-Nd relative to Gd-Er is more obvious in PAAS normalized REE patterns than average limestone normalized, with average NdSN/GdSN of 0.56 whereas NdRN/GdRN of 0.78).
Table 4 Rare earth element concentrations (10-6) of limestones
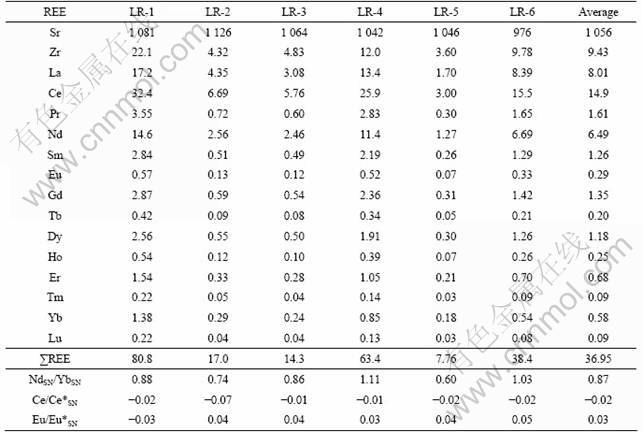
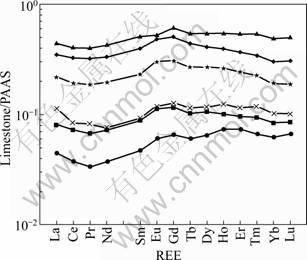
Fig.5 PAAS-normalized REE patterns of limestones
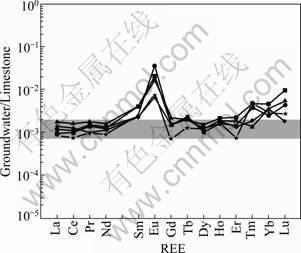
Fig.6 Rock (average limestone) normalized groundwater from limestone aquifer
4.4 Solution complexation
The results of inorganic solution complexation modelling for LW are shown in Fig.7. Only Ln(CO3)2- and LnCO3+ (Ln means any lanthanide) had been collected because they were predicted to dominate in groundwater in this study (64.5%-99.8% of Ln can be explained by these two species). It is observed that the proportions of Ln(CO3)2- increase from La to Lu, whereas the proportions of LnCO3+ decrease, for example, the proportions of La(CO3)2- and Lu(CO3)2- in LW-1 are 24.5% and 81.4%, respectively. In addition, the relative proportions of Ln(CO3)2- are positively correlated with pH values, whereas the proportions of LnCO3+ are negatively correlated with pH values (Fig.7). For example, La(CO3)2- accounts for only 8.02% of LW-2 (pH=7.36) and 43.6% for LW-6 (pH=8.28), whereas Lu(CO3)2- accounts for 9.42% of LW-6 and 37.6% of LW-2.
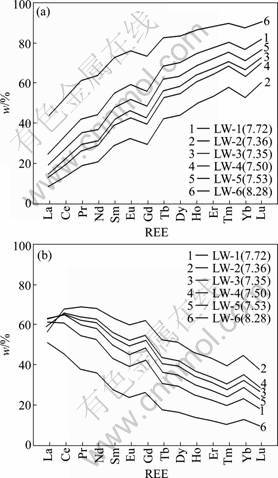
Fig.7 Results of speciation model for groundwater from limestone aquifer: (a) Ln(CO3)2-; (b) LnCO3+
5 Discussion
5.1 REE enrichment and fractionation of groundwater
Figure 6 demonstrates that LW samples show similar REE patterns with their aquifer rocks (limestones) among La-Nd and Gd-Er. However, Fig.4 indicates that REE distribution patterns of the groundwater differ from PAAS. Inspection of Fig.4 reveals that the groundwater samples have PAAS normalized REE patterns that are grossly similar to those of seawater [22-23] except for Ce and Eu anomalies.
Many previous investigations cite aquifer material as the primary source of REEs in groundwater [7-8]. Because groundwater in this study is in intimate contact with limestones, the weathering or solid-liquid exchange reactions between groundwater and limestones represent the most likely source of REEs to the groundwater. It can be seen in Fig.6 that the REE patterns of groundwater and limestones are similar between La-Nd and Gd-Er, indicating that the limestones play important roles in controlling the REE patterns of groundwater.
In addition, the dramatic fractionation of some heavy REEs (Tm-Lu) indicates that other processes must be engaged (e.g. chemical reactions between groundwater and HREE-enriched minerals). The correlation analysis shows that Zr concentrations of groundwater are well correlated with ∑REE (R=0.76) than Sr concentrations (R=0.22), indicating that terrigenous materials such as zircon play more important roles in controlling the REE concentrations of groundwater. However, for the correlation analysis of Sr, Zr versus NdSN/YbSN, they have R=0.60 and 0.48, respectively. Such results indicate that although the REE concentrations of groundwater can be controlled by terrigenous materials in the limestones, the Sr enriched minerals (e.g. calcite in limestone) play the most important role in controlling the REE fractionation of groundwater.
The fractionation of REEs of groundwater can also be affected by solution and surface complexation reactions [9, 24]. Speciation modeling shows that the HREEs of groundwater are predominantly complexed as Ln(CO3)2-, whereas LREEs are LnCO3+ dominant, and the proportions of Ln(CO3)2- increase with increasing the relative atomic mass (Fig.7). Because in neutral to slightly alkaline conditions, the particle or aquifer rock surface is negatively charged, the positively charged LnCO3+ species will be removed from the groundwater [9]. Because HREEs are predominantly complexed as Ln(CO3)2-, they will be stabilized in groundwater, leading to the fractionation of L- and HREEs. In this study, the highest concentration of Ln(CO3)2- sample (LW-6 with pH=8.28) having the highest NdSN/YbSN ratio might be an expression.
5.2 Ce anomalies
The LW exhibits weak negative Ce anomalies when normalized to PAAS as well as average limestone (Table 3, Figs.4 and 6). These negative Ce anomalies may reflect oxidative conditions of the aquifer, whereby Ce3+ is oxidized to less soluble Ce4+ [6]. However, field measurement indicates that the groundwater in the Taiyuan Fm limestone aquifer is in reducing condition (Eh from -60 to -300 mV), which will lead Ce to be Ce3+ in groundwater, rather than leaving.
Another possible explanation is that the Ce anomalies of groundwater reflect solubility differences of Ce redox species related to pH conditions in the aquifer system. The previous study revealed that Ce3+ is more stable in low pH conditions [7, 11], and it will lead to more negative Ce anomaly with pH increasing. In fact, although the highest pH groundwater (LW-6) has the lowest Ce/Ce*SN value, the correlation between pH and Ce/Ce*SN is not obvious (R=-0.38).
Alternatively, they may reflect a signature inherited from the aquifer rock [7]. For example, groundwater samples from aquifers composed of marine derived carbonate rocks tend to obtain negative Ce anomalies [22-23]. We had demonstrated that calcite plays important roles in controlling the fractionation of REEs of groundwater, and then they might be also important in controlling the Ce anomalies. The correlation coefficients of Sr and Zr versus Ce/Ce*SN are 0.82 and 0.63, respectively, indicating that either marine derived materials or terrigenous materials are important in controlling the Ce anomalies, although the former plays more important roles.
5.3 Application to water source discrimination
This study demonstrates that aquifer rocks, as well as their mineral compositions, play important roles in controlling the REE characteristics of groundwater. Because of the differences between aquifers (Table 1), the groundwater in each aquifer should be different.
Take Renlou Coal Mine in this study as an example. The Quaternary aquifer is composed of mixed rocks (including limestones, sandstones, etc), the coal-bearing aquifer is mainly composed of sandstones (with minor limestones), whereas the Taiyuan Fm and Ordovician limestone aquifers are mainly composed of limestones. Considering the similarity of REE characteristics between aquifer rocks and groundwater, the groundwater from Taiyuan Fm and Ordovician aquifers tend to inherit the marine derived signatures, and they will have the lowest NdSN/YbSN (<0.5) and highest Y/Ho ratios (>35) [23]. Comparatively, the coal-bearing groundwater will show the highest positive Eu anomalies (Eu/Eu*SN>1) because of the enrichment of plagioclase [5]. Moreover, they will be expected to have chondritic Y/Ho ratios (~27) [23]. Although groundwater from the Quaternary aquifer probably shows chondritic Y/Ho ratios (27-35), the lack of obvious positive Eu anomalies (Eu/Eu*SN<1) can be discriminated from the coal-bearing groundwater. However, groundwater from the lowest Taiyuan Fm and Ordovician aquifers are hard to be distinguished because of their similarity of aquifer rocks and redox conditions; so, other methods (e.g. major ions, isotopic and trace elements) are needed.
6 Conclusions
1) The groundwater from limestone aquifer in Renlou Coal Mine are Cl-Ca, Na water, with warm temperature (34.0-37.2 °C), circum-neutral pH (7.35- 8.28) and high TDS (1 746-2 849 mg/L);
2) The groundwater has higher REE concentrations (ρ(Nd)=0.006-0.010 μg/L) relative to groundwater with circum-neutral pH, and shows HREEs enrichment relative to LREEs and weak or no negative Ce anomalies when normalized to PAAS. It has similar REE patterns with the aquifer rocks (limestones) except for some HREEs (Tm-Lu).
3) The similarities of REE patterns (including fractionation and anomalies) between groundwater and aquifer rocks imply that aquifer rocks, as well as their minerals, play important roles in controlling the REE characteristics of groundwater.
4) The controlling of aquifer rocks to the REE characteristics of groundwater, in combination with the differences between aquifer rocks in Renlou Coal Mine, provides probability for discrimination of groundwater from different aquifers by using REEs.
References
[1] HENDERSON P. Rare earth element geochemistry [M]. New York: Elsevier, 1984: 466.
[2] HANSON G N. Rare earth elements in petrogenetic studies of igneous systems [J]. Earth Planet Science Letters, 1980, 8: 371-406.
[3] TAYLOR S R, MCLENNAN S M. The continental crust: Its composition and evolution [M]. Oxford: Blackwell Scientific Publications, 1985: 312.
[4] BRAUN J J, PAGEL M, MULLER J P, BILONG P, MICHARD A, GUILLET B. Cerium anomalies in lateritic profiles [J]. Geochimica et Cosmochimica Acta, 1990, 54: 781-795.
[5] BAU M. Rare-earth element mobility during hydrothermal and metarmorphic fluid-rock interaction and the significance of the oxidation state of europium [J]. Chemical Geology, 1991, 93: 219-230.
[6] LEYBOURNE M I, GOODFELLOW W D, BOYLE D R, HALL G M. Rapid development of negative Ce anomalies in surface waters and contrasting REE patterns in groundwaters associated with Zn-Pb massive sulphide deposits [J]. Applied Geochemistry, 2000, 15: 695-723.
[7] SMEDLEY P L. The geochemistry of rare earth elements in groundwater from the Carnmenellis area, southwest England [J]. Geochimica et Cosmochimica Acta, 1991, 55: 2767-2779.
[8] JOHANNESSON K H, STETZENBACH K J, HODGE V F, KREAMER D K, ZHOU X P. Delineation of groundwater flow system in the southern Great Basin using aqueous rare earth element distributions [J]. Ground Water, 1997, 35: 807-819.
[9] TANG J W, JOHANNESSON K J. Controls on the geochemistry of rare earth elements along a groundwater flow path in the Carrizo Sand aquifer, Texas, USA [J]. Chemical Geology, 2006, 225: 156-171.
[10] BIDDAU R, BENSIMON M, CIDU R, PARRIAUX A. Rare earth elements in groundwater from different Alpine aquifers [J]. Chemie der Erde Geochemistry, 2009, 69(4): 327-339.
[11] JOHANNESSON K H. Rare earth elements in groundwater flow systems [M]. Netherlands: Springer, 2005: 187-222.
[12] GUI He-rong, CHEN Lu-wang. Hydrogeochemistric evolution and discrimination of groundwater in mining district [M]. Beijing: Geological Publishing House, 2007: 32-44. (in Chinese)
[13] CHEN Lu-wang, GUI He-rong, Yin Xiao-xi. Composing characteristic and evolution law of carbon and oxygen stable isotopes in groundwater dissolved carbonate [J]. Journal of China Coal Society, 2008, 33(5): 537-542. (in Chinese)
[14] CHEN Lu-wang, GUI He-rong, YIN Xiao-xi. Composing characteristic of hydrogen and oxygen stable isotopes and tracing of hydrological cycle [J]. Journal of China Coal Society, 2008, 33(10): 1107-1111. (in Chinese)
[15] CHEN Hong-jiang, LI Xi-bing, LIU Ai-hua, PENG Shu-quan. Identifying of mine water inrush sources by fisher discriminant analysis method [J]. Journal or Central South University: Science and technology, 2009, 40(4): 1114-1120. (in Chinese)
[16] SHABANI M B, AKAGI T, SHIMIZU H, MASUDA A. Determination of trace lanthanides and yttrium in seawater by inductively coupled plasma mass spectrometry after preconcentration with solwent extraction and back-extraction [J]. Analytical Chemistry, 1990, 62: 2709-2714.
[17] LIU Ying, LIU Hai-chen, LI Xian-hua. Simultaneous and precise determination of 40 trace elements using ICP-MS [J]. Geochemica, 1996, 25(6): 552-558. (in Chinese)
[18] LEWIS A J, KOMNINOU A, YARDLEY B W D, PALMER M R. Rare earth element speciation in geothermal fluids from Yellowstone National Park, Wyoming, USA [J]. Geochimica et Cosmochimica Acta, 1998, 62(4): 657-663.
[19] ZHU Zhao-zhou, LIU Cong-qiang, WANG Zhong-liang, LI Jun, ZHOU Zhi-hua. Inorganic speciation of rare earth elements in Chaohu Lake and Longganhu Lake, East China [J]. Journal of the Chinese Rare Earth Society, 2006, 24(1): 110-115. (in Chinese)
[20] RONNBACK P, ASTROM M, GUSTAFSSON J P. Comparison of the behavior of rare earth elements in surface waters, overburden groundwaters and bedrock groundwaters in two granitoidic settings, Eastern Sweden [J]. Applied Geochemistry, 2008, 23(7): 1862-1880.
[21] JOHANNESSON K H, HENDRY M J. Rare earth element geochemistry of groundwaters from a thick till and clay-rich aquitard sequence, Saskatchewan, Canada [J]. Geochemica et Cosmochimica Acta, 2000, 64: 1493-1509.
[22] FRIMMEL H E. Trace element distribution in Neoproterozoic carbonates as palaeo environmental indicator [J]. Chemical Geology, 2009, 258: 338-353.
[23] WEBB G E, KAMBER B S. Rare earth elements in Holocene reefal microbialites: A new shallow water proxy [J]. Geochimica et Cosmochimica Acta, 2000, 64: 1557-1565.
[24] JOHANNESSON K H, LYONS W B. The rare earth element geochemistry of Mono Lake water and the importance of carbonate complexing [J]. Limnology and Oceanography, 1994, 39: 1141- 1154.
(Edited by YANG Bing)
Foundation item: Project(40873015) supported by the National Natural Science Foundation of China; Project(08010302062) supported by the Eleventh Five- year Scientific and Technological Program of Anhui Province, China
Received date: 2010-07-28; Accepted date: 2010-11-26
Corresponding author: SUN Lin-hua, PhD; Tel: +86-557-2871038; E-mail: sunlinh@126.com