J. Cent. South Univ. (2017) 24: 30-38
DOI: 10.1007/s11771-017-3405-0

Adsorption of residual amine collector HAY from aqueous solution by refined carbon from coal fly ash and activated carbon
LI Xian-bo(李显波)1, 2, 3, YE Jun-jian(叶军建)2, 3, 4, QIU Yue-qin(邱跃琴)2, 3, 4, LI Long-jiang(李龙江)2, 3, 4,
MAO Song(卯松)2, 3, 4, LIU Zhi-hong(刘志红)2, 3, 4, ZHANG Qin(张覃)2, 3, 4
1. College of Materials and Metallurgy, Guizhou University, Guiyang 550025, China;
2. Mining College, Guizhou University, Guiyang 550025, China;
3. National & Local Joint Laboratory of Engineering for Effective Utilization of
Regional Mineral Resources from Karst Areas, Guizhou University, Guiyang 550025, China;
4. Guizhou Key Lab of Comprehensive Utilization of Non-metallic Mineral Resources,
Guizhou University, Guiyang 550025, China
Central South University Press and Springer-Verlag Berlin Heidelberg 2017
Abstract: Refined carbon (RC) derived from coal fly ash (CFA) as well as powdered activated carbon (PAC) was investigated as adsorbent to remove residual amine collector HAY from aqueous solution. The RC and PAC were characterized by scanning electron microscopy (SEM), surface area measurement, Zeta potential measurement and Fourier transform infrared (FTIR) spectroscopy. The effect factors and mechanisms of HAY adsorption onto RC and PAC were studied in detail. The results show that the experimental kinetic data agree well with the pseudo second-order equation, and the Langmuir isotherm model is found to be more appropriate to explicate the experimental equilibrium isotherm results than the Freundlich model. The adsorption capacities of PAC and RC increase with pH. It is found that alkaline condition is conducive to the adsorption of HAY onto PAC and RC and the adsorption efficiency of RC is close to PAC at pH near 11. Zeta potential variation of adsorbents suggests that HAY generates electrostatic adsorption onto RC and PAC. FTIR analysis shows that the adsorption is dominantly of a physical process. The Box-Behnken design optimization conditions of process are RC 1 g/L, pH 11, temperature 302 K and initial HAY concentration 100 mg/L. Under these conditions, the measured adsorption ratio and adsorption capacity are 87.91% and 87.91 mg/g, respectively. Thus, the RC is considered to be a potential adsorbent for the removal of residual amine from aqueous solution.
Key words: powdered activated carbon; coal fly ash; refined carbon; adsorption; amine collector HAY
1 Introduction
As a series of effective flotation collectors for oxidized ore, amine and amine salts have been widely used in reverse flotation to remove gangue minerals, i.e., quartz and silicate from siliceous phosphate ore [1, 2]. Residual amine collector in flotation wastewater of phosphate ore is characterized by poor selectivity and high foam stability, which will have detrimental influence on flotation performance before purification. Besides, direct emission of flotation wastewater without treatment will result in the eutrophication of water body and also it is waste of water resources [3]. So, appropriate technologies are needed for dealing with the wastewater from phosphate ore flotation.
Various treatment techniques for removing residual reagents are suggested, such as chemical precipitation [4], electrochemistry [5], ion exchange and biodegradation [6], etc. Chemical precipitation can remove the organic matter effectively, but it will produce a large amount of precipitated sludge and cause secondary pollution [7]. Restricted by drawbacks like high cost, specialized equipment and high energy-consumption [8], techniques such as electrochemistry, ion exchange and biodegradation have not been applied in production yet. While being considered to be of high treatment efficiency and non-pollution, adsorption has become the most common and widely used technique for the treatment of organics-containing wastewater [9, 10]. However, the adsorption is subject to the performance of adsorption material [11]. Activated carbon has become one of the most promising adsorbing materials for removal of organic pollutants from wastewater [12, 13], this is primarily due to their high surface area, micro porous character and relatively large adsorption capacity.
Unfortunately, activated carbon has not been applied in large-scale industry because of its high manufacture and regeneration costs [14, 15]. So, it is essential to develop a low-cost and readily available material to replace activated carbon to remove pollutants from flotation wastewater.
Coal fly ash (CFA) is a kind of industrial waste emitted from coal-fired power stations during pulverized coal combustion. It turns out to be an industrial by-product if not used effectively [16]. Annual generation of CFA is still increasing and the total amount of which is anticipated to reach 580 million tones in China by 2015 [17]. Greenpeace reported only 30% of CFA in China has been reused [18]. Irregular accumulation and inappropriate disposal of CFA increase occupation of vast land and aggregates danger to human and environment.
At present, CFA has found a wide application in construction materials, environmental protection and other fields. However, because CFA contains high content of unburned carbon, it can be utilized only after decarburization [19-21]. Since the carbon experiences a devolatilization during the combustion in the furnace of the power station [22], it has a larger and more intensive porous and thus higher surface area relative to the inorganic matter in the CFA [23], so it is considered as a potential low-cost adsorbent to remove organics from flotation wastewater.
The main objective of the present work is to investigate the adsorption of residual amine collector HAY by using refined carbon (RC) from CFA as an adsorbent, and powdered activated carbon (PAC) was also investigated for comparison. The absorption dynamics and adsorption isotherms of HAY onto RC and PAC were studied. The effects of adsorbent dosage, pH, temperature and initial concentration of HAY on the adsorption of RC were also investigated. The results of this research are expected to explore a low-cost adsorbent for wastewater treatment, and the aim of “waste treats waste” is hopefully achieved.
2 Materials and methods
2.1 Materials
The CFA used in this work was collected from Guizhou Province, China, and the loss on ignition (LoI) is 16.90%. HAY is a kind of amine cationic collector for phosphate ore flotation. H2SO4, HCl and NaOH were purchased from Chongqing Chuandong Chemical (Group) Co., Ltd. (Chongqing, China), while PAC was obtained from Tianjin Guangfu Technology Development Co., Ltd. (Tianjin, China). The HAY solutions of various concentrations (from 100 to 450 mg/L) were prepared by using deionized water from a stock solution of 10 g/L; these solutions were used as simulated flotation wastewater containing HAY. All chemicals are of analytical grade except HAY, which is of industrial grade.
2.2 Preparation of refined carbon
RC was concentrated from CFA by flotation in a 1.5 L flotation cell. Five hundred grams of CFA and proper amount of tap water were added to the flotation cell. After 1 min of mixing 0.5 g of collector (diesel oil) was added and conditioned for 1 min. Then, 0.5 g of pine oil was added as a frother. The air was introduced into the cell 1 min after frother addition. The flotation was conducted for 6 min, and then the floats was concentrated three times without adding reagent and the sinkers scavenging one time with 0.2 g of diesel oil and pine oil respectively. At last, the flotation products were collected, filtered, washed, dried, weighed and analyzed. The LoI of tail ash is 4.87%. The yield and LoI of RC are found to be 7.08% and 69.15%, respectively. Finally, the RC was ground and sieved into a uniform size of 75 μm and stored in a desiccator for further use.
2.3 Characterization methods
The specific surface area of adsorbents was measured using N2 adsorption isotherm at 77 K with a surface area analyzer (ASAP2020M). The total pore volume (Vt) was calculated at P/P0=0.99. The specific surface area (SBET) was calculated by Brunauer-Emmett- Teller method, applied to adsorption branch within the relative pressure P/P0 range of 0.05-0.30. The average pore diameter (Da) was determined by the Barrette- Joyner-Halenda method.
Surface morphology was investigated by using a field emission scanning electron microscopy (SEM, ZEISS). The accelerated voltage was 5 kV, at which samples need coating with gold.
Surface functional groups were examined with a Fourier transform infrared spectroscopy analyzer (FTIR, Nicolet iS50). All samples were mixed with KBr and determined within the range of 4000-400 cm-1 wavenumber.
Zeta potentials were measured by Zeta potential and particle size analyzer (DelsaNanoC). 0.1 g of sample was added to a beaker with 100 mL deionized water or 150 mg/L HAY solutions, and the pH value of the solutions was adjusted by using HCl and NaOH. The Zeta potentials of PAC and RC were determined after ultrasonic oscillation for10 min.
2.4 Absorption kinetics and adsorption isotherms
Adsorption kinetics was studied from batch techniques. Batch experiments were carried out in conical flasks (100 mL) at 298 K and 200 r/min. A weighed amount (0.06 g) of adsorbents was added to HAY solutions (150 mg/L, 100 mL) each time and shaken continuously for different time, and then the suspensions were filtrated and the concentrations of residual reagent were determined according to the standard GB/T 15456—2008. The adsorption capacity at time t (Qt (mg/g)) was calculated using the following equation:
(1)
where Ci (mg/L) is the initial concentration; Ct (mg/L) is the residual concentration of adsorption time t; V (L) is the solution volume; m (g) is mass of adsorbent.
Adsorption isotherms: different concentrations of HAY solutions (100, 150, 200, 250, 300, 350, 400 mg/L) 100 mL and 0.06 g adsorbents were added to different conical flasks at 303 K and 318 K and shaken continuously for 60 min to reach the equilibrium. The suspensions were filtrated and analyzed. The equilibrium adsorption capacity Qe (mg/g) was calculated using the following equation:
(2)
where Ce (mg/L) is the equilibrium residual concentration of HAY in solutions.
3 Results and discussion
3.1 Characterizations of adsorbents
The SEM photographs of PAC and RC are shown in Fig. 1. A significant difference can be observed between the surface topography of PAC and RC that the external surface of PAC has a rough texture with a large number of cavities, while RC presents a porous structure with a large number of pores in different sizes and shapes. These cavities and pores on the surface increase the contact area and facilitate pore diffusion during adsorption [24].
The porous structure parameters of PAC and RC are listed in Table 1. SBET value of RC is relatively low compared to that of PAC. This may be attributed to the fact that RC has higher content of ash than PAC. The average pore diameters of PAC and RC are 10.36 nm and 30.10 nm, respectively. They both lie between 2 to 50 nm, indicating that PAC and RC are typical mesoporous materials. The relatively large pore diameter may improve the diffusion of large adsorbate molecules into pores of the adsorbents [15].
3.2 Adsorption condition experiments
3.2.1 Effect of adsorbent dosage
The effect of dosage of PAC and RC on the adsorption of HAY was investigated by conducting batch experiments at dosage ranges from 0.2 to 0.9 g/L. Figure 2 shows that with increasing adsorbents dosage the adsorption capacities decrease from 447.53 to 125 mg/g for PAC and from 350.64 to 102.21 mg/g for RC. Contrary to the adsorption capacity, increasing adsorbent dosage is conducive to improving the adsorption ratio.
3.2.2 Effect of pH
The effect of pH on the adsorption of HAY was studied at a pH range of 2 to 12. Figure 3 shows the adsorption capacity and adsorption ratio of PAC and RC as a function of pH. For both PAC and RC, the adsorption capacity and adsorption ratio are found to increase gradually with pH. PAC reaches an optimum adsorption capacity and adsorption ratio at pH 10.5, while for RC the appropriate pH is 11.0, the adsorption capacities of PAC and RC are 194.09 mg/g and 197.60 mg/g, respectively. Therefore, the adsorption efficiency of RC is close to that of PAC. Moreover, it is more conducive to the adsorption of HAY onto PAC and RC under alkaline conditions. The difference between the effects of pH on the adsorption of HAY may be largely related to the change of net charge on the surface of PAC and RC during adsorption process, which is caused by pH variation in solution, and the characteristics of HAY.
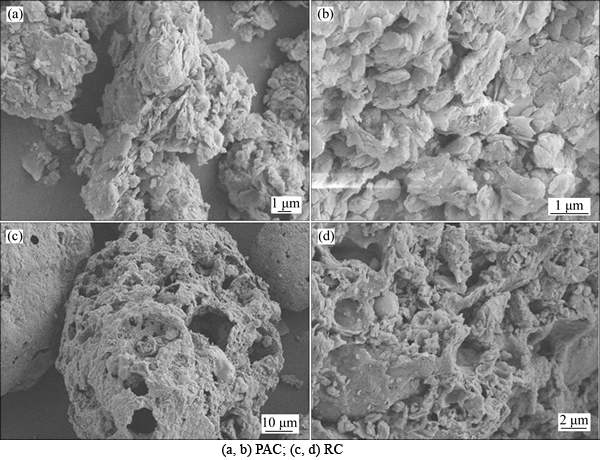
Fig. 1 SEM photographs:
Table 1 Characteristics of porous structure of PAC and RC
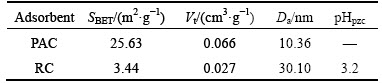
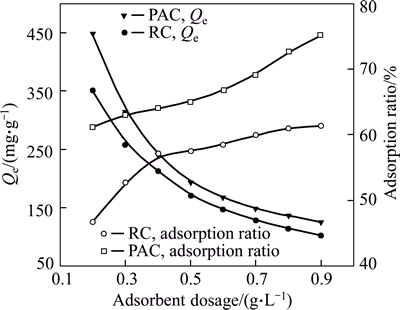
Fig. 2 Effect of adsorbent dosage on adsorption of HAY (Initial concentration: 150 mg/L; initial pH: 5; temperature: 293 K; adsorption time: 60 min)
The solution pH affects the surface charge of the adsorbent, while Zeta potential influences the adsorption of HAY. Zeta potentials of PAC and RC as a function of pH in the absence and presence of HAY (150 mg/L) were measured and the results are shown in Fig. 4. The Zeta potentials of both PAC and RC decrease with increasing pH and show a similar trend. The Zeta potential of PAC is negative between pH 2 and 12, and the pHzpc of RC has been found to be 3.2. In the presence of collector HAY, The Zeta potentials of PAC and RC are significantly shifted to more positive at pH<11, and this indicates a strong electrostatic absorption of HAY onto PAC and RC. However, Zeta potentials of PAC and RC remain negative at pH above 11. There exists competitive adsorption between HAY and OH- on the adsorbent surface and the adsorption effect becomes worse. This observation is consistent with the results shown in Fig. 3.
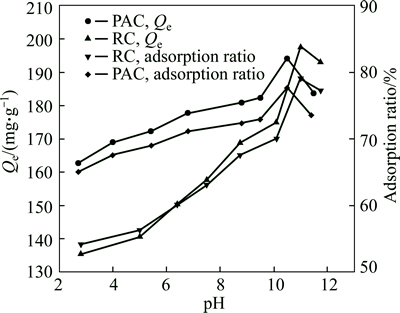
Fig. 3 Effect of pH on adsorption of HAY onto PAC and RC (Initial concentration: 150 mg/L; adsorbent dosage: 0.6 g/L; temperature: 293 K; adsorption time: 60 min)
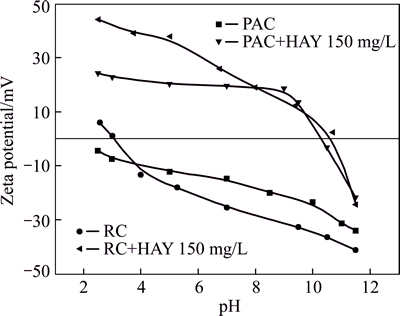
Fig. 4 Zeta potentials of PAC and RC as function of pH in absence and presence of HAY
3.2.3 Box-Behnken response surface optimization analysis
In order to further optimize the adsorption performance, the parameters such as RC dosage, pH, temperature and initial concentration were evaluated individually by means of single-factor experiments. Box- Behnken design in Design-expert 8.06 software with 4 factors in three levels was selected. Twenty-nine tests were carried out, and the results are presented in Table 2 and Table 3. The experimental data were subjected to a multiple regression analysis to quantify the main effects of the variables, as well as the interactions and the quadratic contributions to the responses of adsorption ratio. In Table 3, all statistically significant effects are identified using an asterisk. The optimized regression equation is obtained as follows:
y=889.06-63.69x1-183.90x2+6.81x3-0.65x4+7.40x1x2-0.25x2x3+0.04x2x4+
(3)
The analysis of variance (ANOVA) indicates that the R2 is 0.9986 and the standard deviation is 0.56. The model is significant (p<0.0001) and the lack of fit is ignorable. The analysis shows that the main factors (x1, x2, x3 and x4) and their interaction x1x2, x2x3 and x2x4 are significant model terms for adsorption ratio.
Table 2 Design and response values of Box-Behnken test
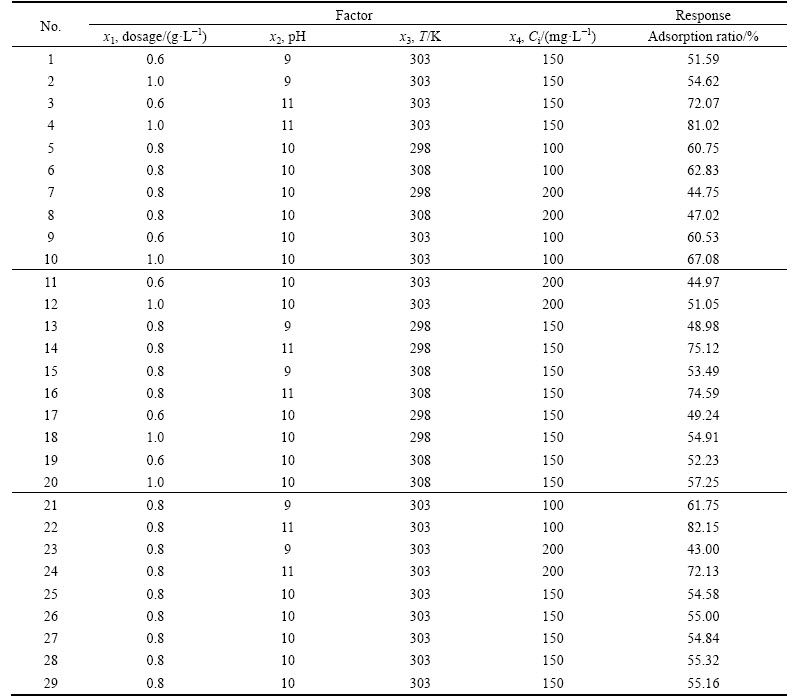
Table 3 Regression model analysis of variance of Box-Behnken design
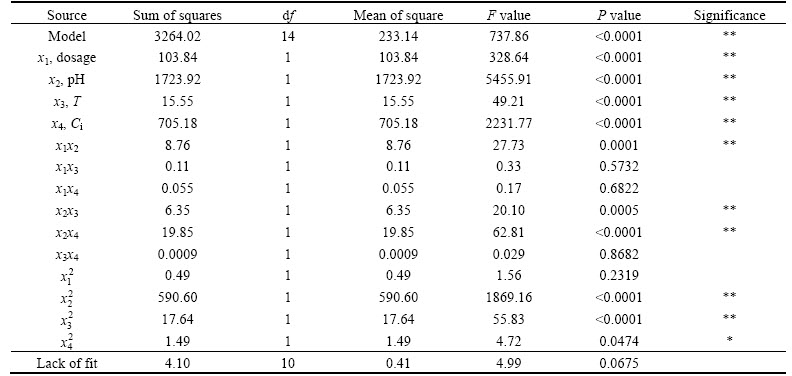
To facilitate visualization of the effects of the variables, Figs. 5, 6 and 7 illustrate the response surface obtained from Eq. (3). Figures 5, 6 and 7 show that interactions of RC dosage and pH, pH and temperature, pH and initial concentration affect significantly the adsorption ratio. Increase in both pH and RC dosage has a positive effect on the adsorption ratio. Conversely, initial concentration negatively affects the adsorption ratio. Therefore, the optimized process conditions are RC dosage 1 g/L, pH 11, T 302 K, initial HAY concentration 100 mg/L. The verification experiments were carried out under these conditions. The measured adsorption ratio and adsorption capacity are 87.91% and 87.91 mg/g, respectively.
3.3 Adsorption kinetics
Kinetic study is usually conducted to analyze the time for reaching the adsorption equilibrium. The classic Lagergren model [25] was used to examine the adsorption. The pseudo first-order (Eq. (4)) and pseudosecond-order (Eq. (5)) kinetic equations are expressed as
(4)
(5)
where K1 (min-1) and K2 (g/(mg·min)) are the adsorption rate constants of corresponding kinetic equations. The correlation coefficient (R2) was evaluated by plotting the graph for models.
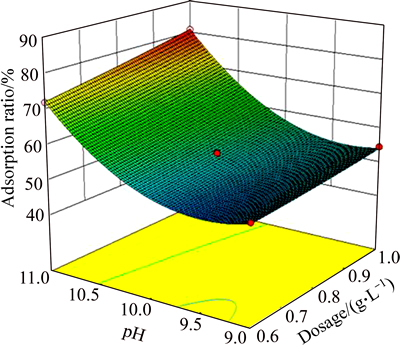
Fig. 5 Adsorption ratio response surface as a function of pH and adsorbent dosage
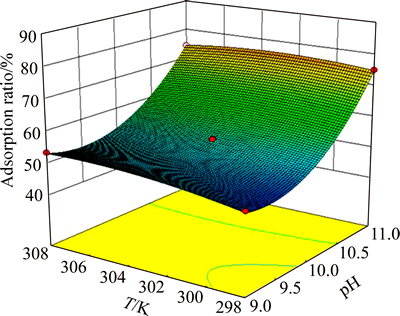
Fig. 6 Adsorption ratio response surface as a function of pH and temperature
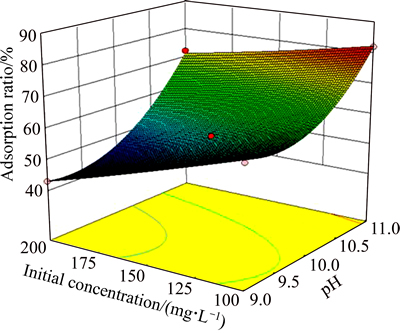
Fig. 7 Adsorption ratio response surface as a function of pH and initial concentration
The kinetic plots for 150 mg/L of HAY adsorption onto PAC and RC are shown in Fig. 8. It is shown that the adsorption capacities of PAC and RC increase rapidly at the initial stage, thereafter proceed at a slower rate and finally reach saturation. The PAC and RC reach their absorption equilibrium after 10 and 15 min, respectively. The higher adsorption capacities at the initial stage are due to the larger concentration gradients between adsorbate in solution and on the adsorbent surface [26]. Besides, the adsorption capacity of PAC is higher than RC.
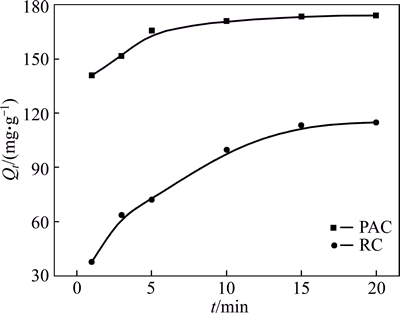
Fig. 8 Adsorption of HAY onto PAC and RC as a function of time (Initial concentration: 150 mg/L; initial pH: 5; adsorbent dosage: 0.6 g/L; temperature: 293 K)
The adsorption capacities of PAC and RC were fitted with the pseudo first-order and pseudo second- order kinetic equations and the results are shown in Figs. 9 and 10, respectively. The fitting parameters are presented in Table 4. The values of R2 for the pseudo second-order model are all higher than 0.99, so the pseudo second-order model describes the adsorption process better than the pseudo first-order model. This finding is also in good agreement with previous studies [25, 26]. The equilibrium adsorption capacity and the adsorption rate constant of PAC are higher than RC.
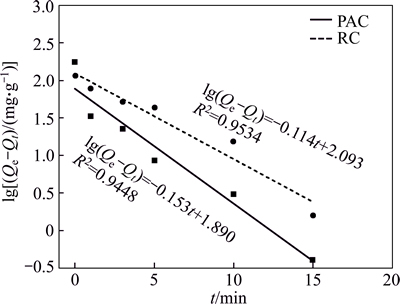
Fig. 9 First-order kinetics fitting of HAY adsorption onto PAC and RC
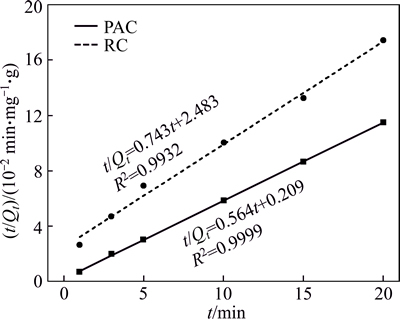
Fig. 10 Second-order kinetics fitting of HAY adsorption onto PAC and RC
Table 4 Kinetics parameters for adsorption of HAY onto PAC and RC
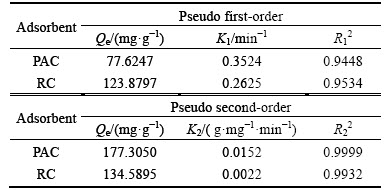
3.4 Adsorption isotherms
Adsorption isotherms are helpful in understanding the nature of adsorption phenomenon, and the adsorption capacity of adsorbent can be obtained by adsorption isotherms under certain concentration of adsorbate. In current study, the adsorption of organic matter onto PAC was studied by two adsorption isotherm models, Langmuir and Freundlich isotherms:
Langmuir isotherm:
(6)
Freundlich isotherm:
(7)
where Qm (mg/g) and KL (L/mg) are the Langmuir constants in relation to the maximum monolayer capacity and energy of adsorption, respectively. The KF and 1/n are Freundlich constants related to adsorption capacity and surface heterogeneity, respectively. Langmuir isotherm assumes a monolayer adsorption surface without any lateral interaction between adsorbed molecules. Freundlich model is for describing multilayer adsorption with interaction between adsorbed molecules [14].
The adsorption isotherms of HAY onto PAC and RC at 303 K and 318 K are shown in Fig. 11. The equilibrium adsorption capacities increase with increasing equilibrium concentration at the same temperature. Higher temperature is conducive to the adsorption under the same equilibrium concentration, which means that the adsorption process is endothermic. The results of Langmuir fitting and Freundlich fitting of HAY onto adsorbents are shown in Figs. 12 and 13 respectively, and the parameters of isotherms are given in Table 5. Based on the correlation coefficients (R2), it is obvious that the adsorption of HAY onto PAC and RC are best fitted to the Langmuir adsorption isotherm for the entire range of concentrations. The results are in close agreement with previous reports [25, 27].
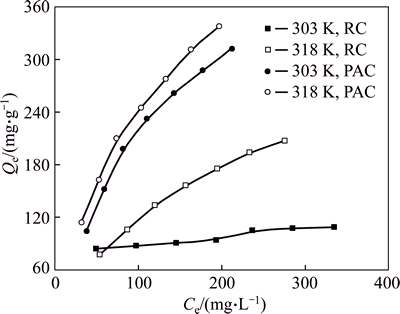
Fig. 11 Adsorption isotherm of HAY adsorption onto PAC and RC (Initial pH: 5; adsorbent dosage: 0.6 g/L; adsorption time: 60 min)
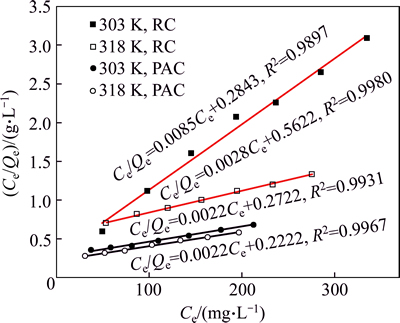
Fig. 12 Langmuir fitting of HAY adsorption onto PAC and RC
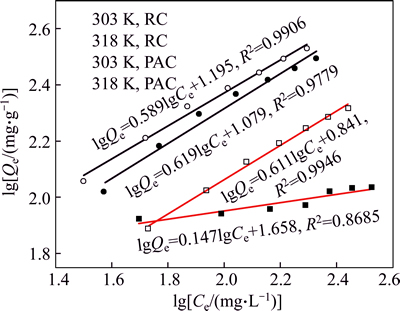
Fig. 13 Freundlich fitting of HAY adsorption onto PAC and RC
Table 5 Isotherm parameters for HAY adsorption onto PAC and RC
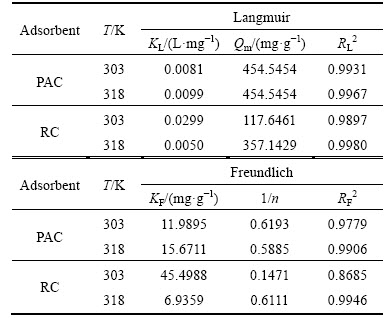
3.5 FTIR analysis
The FTIR spectra of RC, RC+HAY, PAC+HAY, and PAC are shown in Fig. 14. The significant bands located at about 3448.58 cm-1, 3450.51 cm-1 and 3440.39 cm-1 are assigned to O—H stretching vibration of hydroxyl groups. The absorption bands at 1637.75 cm-1, 1636.30 cm-1 and 1627.63 cm-1 are assigned to the stretching vibration of C=O. The peak near 1384.64 cm-1 is assigned to the bending vibration of C—H. The absorption bands at 1033.18 cm-1, 1052.94 cm-1 and 1060.18 cm-1 belong to the stretching vibration of C—O. The surface of PAC and RC has the same functional groups, and the FTIR spectra have no obvious change after adsorption of HAY. This shows that the adsorption of HAY onto PAC and RC is mainly physical adsorption.
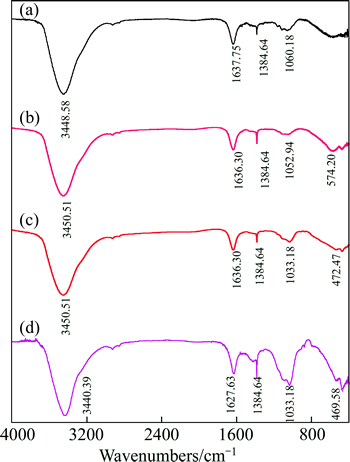
Fig. 14 FTIR spectra of RC (a), RC+HAY (b), PAC+HAY (c), and PAC (d)
4 Conclusions
1) The adsorption capacity and adsorption ratio increase with pH, it is conducive to the adsorption of HAY onto PAC and RC in alkaline conditions and the adsorption efficiency of RC is close to PAC at pH near 11. Zeta potential variation of adsorbents suggests that HAY generates electrostatic adsorption onto RC and PAC.
2) The Box-Behnken design optimization conditions of process are RC dosage 1 g/L, pH 11, temperature 302 K, and initial HAY concentration 100 mg/L. The measured adsorption ratio and adsorption capacity are 87.91% and 87.91 mg/g, respectively. This suggests that RC can be effectively used as a potential adsorbent.
3) The experimental kinetic data agree well with the pseudo second-order kinetics equation, and the adsorption rate constant of PAC is higher than that of RC. The equilibrium data fit well with the Langmuir isotherm model, and the adsorption process of HAY onto PAC and RC is endothermic.
4) The surface of PAC and RC has the same functional groups, and the FTIR spectra have no obvious change after adsorption of HAY, indicating the adsorption of HAY onto PAC and RC is mainly physical adsorption.
References
[1] GUO Fang, LI Jun. Selective separation of silica from a siliceous- calcareous phosphate rock [J]. Mining Science and Technology, 2011, 21(1): 135-139.
[2] MOHAMMADKHANI M, NOAPARAST M, SHAFAEI S Z, AMINI A, AMINI E, ABDOLLAHI H. Double reverse flotation of a very low grade sedimentary phosphate rock, rich in carbonate and silicate [J]. International Journal of Mineral Processing, 2011, 100(3,4): 157-169.
[3] AL-THYABAT S, AL-ZOUBI H. Purification of phosphate beneficiation wastewater: Separation of phosphate from Eshydia Mine (Jordan) by column-DAF flotation process [J]. International Journal of Mineral Processing, 2012, (100,111): 157-169.
[4] LI Xian-bo, ZHANG Yu-biao, LIU Zhi-hong, ZHANG Qin. Experimental study on removal of SO42- and total hardness in circulating water of phosphate rock flotation [J]. Mining and Metallurgical Engineering, 2015, 35(2): 59-62. (in Chinese)
[5] YU Shi-xin, SUN Wen, WANG Yu-lin. Electrochemical treatment of wastewater from flotation of phosphate ores and its recycling for processing uses [J]. Journal Wuhan Institute Chemical Technology, 2002, 25(6): 599-602. (in Chinese)
[6] YAN Heng-zhen. Study on the biodegradability of amine collectors [D]. Wuhan: Wuhan University of Technology, 2010. (in Chinese)
[7] LIU Yue. Research on treatment and reuse technic of phosphorite processing wastewater [D]. Wuhan: Wuhan University of Technology, 2010. (in Chinese)
[8] PADMESH T V N, VIJAYARAGHAVAN K, SEKARAN G, VELAN M. Biosorption of acid blue 15 using fresh water macroalga azolla filiculoides: Batch and column studies [J]. Dyes and Pigments, 2006, 71(2): 77-82.
[9] LIU Fei-fei, Fan Jin-lin, WANG Shu-guang, MA Guang-hui. Adsorption of natural organic matter analogues by multi-walled carbonnanotubes: Comparison with powdered activated carbon [J]. Chemical Engineering Journal, 2013, 219: 450-458.
[10] ZHANG Shu-juan, SHAO Ting, KARANFIL T. The effects of dissolved natural organic matter on the adsorption of synthetic organic chemicals by activated carbons and carbon nanotubes [J]. Water Research, 2011, 45(3): 1378-1386.
[11] ZHONG Xuan-bin, GUO Jian-wei, FU Bao-lin, PENG Jin-ping, YANG Yu-cheng. Adsorption behavior of Fe3+ from condensed water on activated carbon fiber and ACF’s regeneration [J]. Journal of Central South University: Science and Technology, 2014, 45(6): 1778-1783. (in Chinese)
[12] BAUTISTA-TOLEDO I, RIVERA-UTRILLA J, FERRO-GARCIA M A, MORENO-CASTILLA C. Influence of the oxygen surface complexes of activated carbons on the adsorption of chromium ions from aqueous solutions: Effect of sodium chloride and humic acid [J]. Carbon, 1994, 32(1): 93-100.
[13] NAMASIVAYAM C, SANGEETHA D. Recycling of agricultural solid waste, coir pith: Removal of anions, heavy metals, organics and dyes from water by adsorption onto ZnCl2 activated coir pith carbon [J]. Journal of Hazardous Materials, 2006, 135(1-3): 449-452.
[14] ANIRUDHAN T S, SREEKUMARI S S. Adsorptive removal of heavy metal ions from industrial effluents using activated carbon derived from waste coconut buttons [J]. Journal of Environmental Sciences, 2011, 23(12): 1989-1998.
[15] LI Xin, WANG Guang-zhi, LI Wei-guang, WANG Ping, SU Cheng-yuan. Adsorption of acid and basic dyes by sludge-based activated carbon: Isotherm and kinetic studies [J]. Journal of Central South University, 2015, 22(1): 102-113.
[16] SUN Xiu-yun, MA Fang-bian, SHI Xiao-kun, YAN Hui, WANG Lian-jun. Adsorption of Pb(II) on SBA-15 synthesized from fly ash [J]. Journal of Central South University: ScienceandTechnology, 2014, 45(11): 4093-4099. (in Chinese)
[17] YAO Z T, XIA M S, SARKER P K, CHEN T. A review of the alumina recovery from coal fly ash with a focus in China [J]. Fuel, 2014, 120: 74-85.
[18] Greenpeace. The true cost of coal-an investigation into coal ash in China [EB/OL]. [2015-10-17]. http:// www. greenpeace.org/usa/en/ media-center/reports/The-True-Cost-of-Coal-Coal-Ash-in-China/.
[19] PEDERSEN K H, JENSEN A D, SKJOTH-RASMUSSEN M S. A review of the interference of carbon containing fly ash with air entrainment in concrete [J]. Progress in Energy and Combustion Science, 2008, 34 (2): 135-154.
[20] LUCIE B. Unburned carbon from coal combustion ash: An overview [J]. Fuel Processing Technology, 2015, 134: 136-158.
[21] ALTUN N E, XIAO Chuang-fu, HWANG J Y. Separation of unburned carbon from fly ash using a concurrent flotation column [J]. Fuel Processing Technology, 2009, 90(12): 1464-1470.
[22] RUBIO B, IZQUIERDO M T, MAYORAL M C, BONA M T, ANDRES J M. Unburnt carbon from coal fly ashes as a precursor of activated carbon for nitric oxide removal [J]. Journal of Hazardous Materials, 2007, 143(1-2): 561-566.
[23] BLISSETT R S, ROWSON N A. A review of the multi-component utilization of coal fly ash [J]. Fuel, 2012, 97: 1-23.
[24] TAY T, UCAR S, KARAGOZ S. Preparation and characterization of activated carbon from waste biomass [J]. Journal of Hazardous Materials, 2009, 165(1-3): 481-485.
[25] SONG Xiao-lan, ZHANG Ying, YAN Cheng-yin, JIANG Wen-juan, CHANG Cai-min. The Langmuir monolayer adsorption model of organic matter into effective pores in activated carbon [J]. Journal of Colloid and Interface Science, 2013, 389(1): 213-219.
[26] YU Jing, LU Lv, PEI Lan, ZHANG Shu-juan, PAN Bing-cai, ZHANG Wei-ming. Effect of effluent organic matter on the adsorption of perfluorinated compounds onto activated carbon [J]. Journal of Hazardous Materials, 2012, 225-226: 99-106.
[27] MAHAMAD M N, ZAINI M A A, ZAKARIA Z A. Preparation and characterization of activated carbon from pineapple waste biomass for dye removal [J]. International Biodeterioration & Biodegradation, 2015, 102: 274-280.
(Edited by YANG Bing)
Cite this article as: LI Xian-bo, YE Jun-jian, QIU Yue-qin, LI Long-jiang, MAO Song, LIU Zhi-hong, ZHANG Qin. Adsorption of residual amine collector HAY from aqueous solution by refined carbon from coal fly ash and activated carbon [J]. Journal of Central South University, 2017, 24(1): 30-38. DOI: 10.1007/s11771-017-3405-0.
Foundation item: Projects(2013BAB07B03, 2013BAC15B01) supported by the National Key Technology Research and Development Program of the Ministry of Science and Technology of China; Project(51264005) supported by the National Natural Science Foundation of China; Project(Qiankehejz [2014]2009) supported by the Key Foundation of Science and Technology of Guizhou Province, China; Project([2013]019) supported by “125” Major Special Project of Guizhou Province, China
Received date: 2015-11-25; Accepted date: 2016-03-17
Corresponding author: ZHANG Qin, Professor, PhD; Tel: +86-851-88292081; E-mail: zq6736@163.com