
Effects of tourmaline on microstructures and photocatalytic activity of TiO2/SiO2 composite powders
MENG Jun-ping(孟军平), LIANG Jin-sheng(梁金生), LIANG Guang-chuan(梁广川),
YU Jun-mei(于俊梅), PAN Yan-fen (潘艳芬)
Institute of Power Source and Ecomaterials Science, Hebei University of Technology, Tianjin 300130, China
Received 17 August 2005; accepted 22 November 2005
Abstract: The SiO2/TiO2 composite powders including mineral tourmaline powders (T/SiO2/TiO2) were prepared from a sol made by a two-step hydrolysis method, using metasilicate ester as precursor. The powders were characterized by scanning electron microscopy (SEM). The photocatalytic activity of the sample was evaluated by the photocatalytic degradation of methyl orange. The effects of heat-treatment on the photocatalytic activity were discussed. It is found that the T/SiO2/TiO2 composite powders show higher photocatalytic activity when including 10% SiO2 and 4% tourmaline. Moreover, the photocatalytic mechanism of tourmaline on the powders was proposed.
Key words: TiO2/SiO2 powders; composite materials; titania; photocatalytic activity; tourmaline
1 Introduction
Ever since the discovery of the photocatalytic property of titania by FUJISHIMA and HONDA[1] in 1972, the photocatalytic technique has now become one of the popular research subjects in the fields of photochemistry and environment protection due to its lower cost, non-toxicity, stronger antioxidation and so on[2,3]. The photocatalytic properties should be enhanced in order to improve the practicability of such photocatalytic materials as TiO2. Many researchers reported that titania particles loaded with Pt, Ag, and Fe show improved photocatalytic activity[4-6]. Some multicomponent or mixed oxides such as SrTiO3, titania/zirconia, and silica-titania have good photoactivity [7,8]. Especially, titania-silica mixed oxide was used as not only a good photocatalyst itself but also a support material for other chemical reaction catalyst [7]. ANDERSON et al [9] reported the effects of incorporation silica on the behavior of the titania-based photocatalyst prepared by a sol–gel technique. According to the result for the decomposition of rhodamine-6G(R-6G), the titania/silica mixed oxide with a mass ratio of 30∶70 produced the highest activity about three times higher than Degussa P25 titania. KYEONG et al [10] reported that 30% (mass fraction, the same below if not mentioned) silica in titania was an optimum mixture concentration for the oxidation of trichloroethylene and the increase of photocatalytic activity due to the large pore size and pore volume of mixed oxide particles by the addition of silica.
Tourmaline is a kind of complex borosilicate mineral belonging to the trigonal space group[11]. The general chemical formula can be written as XY3 Z6Si6-
O18 (BO3) 3 W4, where X is Na, Ca, K or vacancies; Y is Mg2+, Fe2+, Mn2+, Al, Fe3+, Mn3+, Li; Z is Al, Fe3+, Cr3+, Mg; W is OH, F, O. One of the most important features among the electric properties of tourmaline is the possession of spontaneous and permanent poles, which can produce an electric dipole, especially in a small granule with a diameter of several microns or less[12,13]. Therefore, a strong electric field exists on the surface of a tourmaline granule[13,14]. Also, there exists a high radiotechnology of far infrared. Moreover, tourmaline has many other functions such as shielding of electromagnetic wave and releasing of negative ions. Therefore, tourmaline minerals are a kind of ecomaterials to purify environment[13,15,16].
Till now there is little report about the preparation of SiO2/TiO2 composite powders containing tourmaline powders so far. So, in our study, T/SiO2/TiO2 composite powders were prepared by a sol-gel method. The microstructures of T/SiO2/TiO2powders were studied by SEM. Finally, the effects of heat treatment on the photocatalytic activity of TiO2 were determined by photocatalytic degradation of methyl orange.
2 Experimental
2.1 Preparation of composite sol
The Ti-Si composite sol was prepared by a two-step hydrolysis method. Teraethylorthosilicate (TEOS), ethanol, silane coupling agent of SC-Si 900, water and HCl were blended uniformly. The homogeneous liquid was heat-treated at 70 ℃ for 2 h. After blended solution including acetic acid, tetrabutyl titanate and ethanol was slowly dropped, the resultant solution was obtained by stirring vigorously for 30 min. After adding the water, ethanol and HCl dropwise with a burette, the transparent Ti-Si composite sol was produced under stirring vigorously.
2.2 Preparation of TiO2 composite powders
Tourmaline used here was from Inner Mongolia, China, and its main components are as follows: B2O3 7.73%; Al2O3 32.00%;B2O3 7.73%;K2O 0.32%;Na2O 1.48%;CaO 0.46%; MgO 5.18%;SiO2 36.79%;FeO 8.97%;Fe2O3 2.54%; H2O 2.73%; TiO2 0.68%; P2O5 0.16%. Its average particle size was 1.2 μm. The tourmaline was firstly dipped into the above sol under stirring. The mixture was separated by the process of filtration after aged in ambient temperature for 24 h. The resultant powders were frozen below zero for 24 h, and subsequently heat-treated at 400-700 ℃ for 3 h in air using an electric oven. The following TiO2 composite powders were thus prepared.
2.3 Microstructures and photocatalytic activity of TiO2 composite powders
The microstructures of T/SiO2/TiO2 composite powders were determined by SEM (Philips XL30-TMP). A mono-energetic electron beam was employed, whose cross section and driving voltage were 3 nm and 25 kV, respectively. The current density of the incident electron beam was 33 μA. The composite powders including 10% SiO2 and 4% tourmaline were treated at 600 ℃ for 3 h.
The photocatalytic activity of T/SiO2/TiO2 composite powders was evaluated by methyl orange degradation. A UV lamp (25 W, 253.7 nm) was used as a light source. The averaged intensity of UV irradiance was 49 mW/cm2 by measuring with a UV irradiance meter (UVZDZ-1). The concentration of methyl orange was determined by a 756MC U-V spectrometer.
2.4 Effects of tourmaline on oxygen solved in water
0.48 g tourmaline powders was used to study the effects of its polarity and far infrared on oxygen solved in the water. A plastic cup, 62.2 mm in diameter, was surrounded by tourmaline, poured into 94 mL water. The oxygen concentrations of water in the experimental cup were tested at the intervals of 20 min at room temperature and 0.101 3 MPa. The testing instrument was JPB-607 portable instrument for analyzing dissolved oxygen.
3 Results and discussion
3.1 Microstructures of composite powders
Fig.1 shows the microstructures of composite photocatalysts powders heat-treated at 600 ℃ for 3 h.
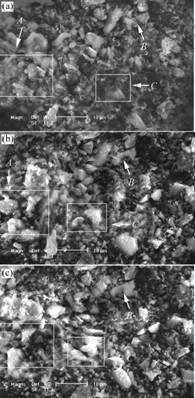
Fig.1 SEM images of composite photocatalysts powders: (a) Before bombardment by electron beam; (b) Under bombardment by electron beam for 10 s; (c) Under bombardment by electron beam for 20 s
It can be seen from Fig.1 that the composite powders, such as particles in zones A, B and C, migrated evidently after electron-beam bombardment of secondary electron. The composite powders captured lots of electrons so that it was more probable for the migration. When the repulsive force exceeded the biggest force that the composite needed to overcome, the migration appeared which confirmed there was polarization among
the composite particles. Fig.1(a) shows the micro- structures before the bombardment by electron beam. Figs.1(b) and (c) show the microstructures under bombardment by electron beam for 10 s and 20 s, respectively. From zones A, B and C in Figs.1(a), (b) and (c), during the electron-beam bombardment of secondary electron, the distance between the powders of A and C zones change. Especially, the particle of B was disappeared.
3.2 Photocatalytic activity of composite powders
Fig.2 shows the curves of photocatalytic degradation of methyl orange by T/TiO2/SiO2 composite powders with different tourmaline contents.
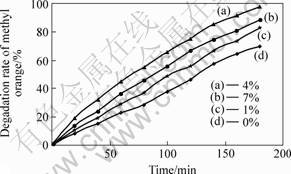
Fig.2 Curves of photocatalytic degradation of methyl orange by T/TiO2/SiO2composite powders with different tourmaline contents
According to Fig.2, the photocatalysis of TiO2/SiO2 composite powders doping with tourmaline particles is higher than that of without tourmaline particles. At the same time, the degradation rate of methyl orange increases as the increasing tourmaline contents from 1% to 4%, especially, achieving the highest value when including 4% tourmaline. However, when the content of tourmaline is over 4%, as the increasing content, the degradation rate, such as curve (d), decreases gradually.
3.3 Effects of tourmaline on oxygen solved in water
Fig.3 shows the effects of tourmaline on the concentrations of oxygen solved in the water.
It can be seen that, the oxygen value is the highest when threw tourmaline into water; remains relatively high when the water in the cup surrounded by tourmaline; the lowest when there is no tourmaline.
3.4 Enhancement of tourmaline on photocatalysis of composite powders
The chief reactions of titania photocatalysis in aqueous solution are as follows[3]:
TiO2
e-+ h+ (1)
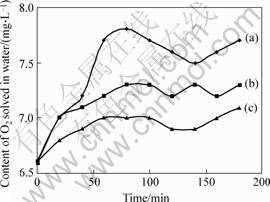
Fig.3 Effects of tourmaline on O2 solved in water: (a) Tourmaline in water; (b) Tourmaline outside cup;(c) Without tourmaline
(2)
(3)
h++ H2O
·OH (4)
[H2O]m=[H2O]n (m>n) (5)
[H2O]n
nH2O (6)
H2O=H++ OH- (7)
OH-+ h+
·OH (8)
·OH+ organic + O2
CO2+H2O (9)
where formula (4) is the simplifier of formulas (5), (6), (7) and (8). The above formulas show the possible reactions that can occur when a solution containing a semiconductor adsorbs a photon (hv) of a suitable wavelength. The electron (e-) and hole (h+) pair may recombine with the generation of thermal. If they are separated they can become involved in electron transfer reactions with other species in the solution, for example oxidation of species ·OH or reduction of species ·
.
When the T/TiO2/SiO2 composite powders are excited by light, the light induces the generation of h+/ e- pairs in the valence band (VB) and conduction band (CB), respectively. At this time, due to the strong electric field of doped tourmaline, the photo-excited electrons are adsorbed tightly on the anode of tourmaline. Thus, the recombination of e- and h+, namely the formula (2) was inhibited and it was more probable that the hydroxylic groups reacted with the holes, generating ·OH radicals. In this case formulas (3) and (4) took place. Hence, the following reactions such as formulas (5) and (6) are succeeding. However, when the content of tourmaline was over 4%, the phocatalytic activity decreased gradually. This is mainly because, the electric field itself adsorbed or disturbed one another when including too much tourmaline. In this case the e-/h+ pair could recombine with the generation of thermal, namely, formula (2) was promoted, which could inhibit the formation of ·OH, as seen from formulas (3) and (9). Besides the amount of photo-excited holes decreased as the decreasing of TiO2/SiO2, the amount of e-/h+ pair was influenced, inhibiting formula (1) and leading to the decreasing of the photocatalysis.
Additional, according to the reaction process of titania photocatalysis, the increase of the oxygen in the system was favorable for the process. In Fig.3, tourmaline was outside of the cup, which separated the action of polarity on the water. When tourmaline was added into the water directly, both the far infrared and polarity of tourmaline had effects on the water. Thus, the oxygen value in Fig. 3 is the highest. It is known that there exists a high electric field of 107 -104 V/m in the range of tens of micron on the tourmaline surface, which can ionize water molecules into OH-(H2O)n and H+. Under the coordinating of far infrared and electric polarity, the water association degree decreased, that is formula (5) was promoted, leading to the increase of O2 solved in the water, which was more favorable for formula (9). So, by increasing the oxygen content in the system, the photocatalysis of the composite powders may be controlled.
4 Conclusions
1) The optimal parameters for preparation of (TiO2, SiO2) composite powders including mineral tourmaline powders are as follows: the silica and tourmaline contents are 10% and 4%, respectively; the heat-treated temperature is 600 ℃; the heat-treated time is 3 h.
2) The composite powders still have polarity and can increase the content of O2 in the system. The as-prepared composite has stronger photocatalysis, which will be helpful to develop new, highly efficient photocatalysts in further studies.
References
[1] FUJISHIMA A, HONDA k. Electrochemical photolysis of water at a semiconductor electrode [J]. Nature, 1972, 328(7): 37-38.
[2] HOFFMAN M R, MARTIN S T, CHOI W, et al. Environmental applications of semiconductor photocatalysis[J]. Chem Rev,1995, 95(1): 69-96.
[3] LINCEBIGLER A L, LU G Q, YATES J T. Photocatalysis on TiO2 surfaces: principle, mechanisms, and selected results [J]. Chem Rev, 1995, 95(3): 735-758.
[4] KOMINAMI H, MURAKAMI S, KATO J, KERA Y. OHTANI B. Correlation between some physical properties of titanium dioxide particles and their photocatalytic actibity for some probe reactions in aqueous systems[J]. J Phys Chem B, 2002, 106: 10501-10507.
[5] NATALIE S, ANNA E, OLGA R. Synthesis and characterization of photocatalytic porous Fe3+/TiO2 layers on glass[J]. J Sol-Gel SciTech, 2001, 21: 109-113.
[6] CHOY J H, LEE H C, JUNG H, KIM H, BOO H. Exfoliation and restacking route to anatase-layered titanate nanohybrid with enhanced photocatalytic activity[J]. Chem Mater, 2002, 14: 2486-2491.
[7] AHUJA S, KUTTY T R N. Nanoparticles of SrTiO3 prepared by gel to crystallite conversion and their photocatalytic activity in the mineralization of phenol [J]. J Photochem Photobiol A: Chem, 1996, 97::99.
[8] FU X, CLARK L A, YANG Q, ANDERSON M A. Enhanced photocatalytic performance of titania-based binary metal oxides: TiO2/SiO2 and TiO2/ZrO2 [J]. Environ Sci Technol, 1996, 30: 647.
[9] ANDERSON C, BARD A J.
Improved photocatalyst of TiO2/SiO2 prepared by a sol-gel synthesis [J]. J Phys Chem, 1995, 99: 9882-9885.
[10] KYEONG Y J, SEUNG B P. Enhanced photoactivity of silica-embedded titania particles prepared by sol-gel process for the decomposition of trichloroethylene[J]. Appl Cata B: Environ, 2000, 25: 249-256.
[11] BARTON R Jr. Refinement of the crystal structure of buergerite and the absolute orientation of tourmalines[J]. Acta Crystallographica, 1969, B25: 1524-1533.
[12] NAKAMURA T, KUBO T. The tourmaline group crystals reaction with water[J]. Ferroelectrics,1992, 137: 13-31.
[13] JI Z J, JIN Z Z, LIANG J S, WANG J, YAN X W. Observation of electric dipole on polar crystalline tourmaline granules[J]. Synth Cryst, 2002, 31(5): 503-508.
[14] YAMAGUCHI S. Surface electric fields of tourmaline[J]. J Appl Phys A, 1983, 31: 183-185.
[15] JI Z J, JIN Z Z, LIANG J S, WANG J, YAN X W. Influence of tourmaline on pH value of water [J]. Chin Environ Sci, 2002, 22(6): 515-519.
[16] YANG R Z, LIAO Z T, CHEN X D. Thermoelectric character of natural blank tourmaline [J]. J Gems Gemmology, 2000, 2(1): 34-38.
(Edited by LONG Huai-zhong)
Foundation item: Project(E2004000033) supported by the Natural Science Foundation of Hebei Province, China
Corresponding author: LIANG Jin-sheng; Tel: +86-22-26582575; Fax: +86-22-26564850; E-mail: liang_jinsheng@sina.com