J. Cent. South Univ. (2012) 19: 2125-2129
DOI: 10.1007/s11771-012-1254-4
Effect of OH- on morphology of Cu2O particles prepared through reduction of Cu(II) by glucose
WANG Yue-jun(王岳俊), ZHOU Kang-gen(周康根)
School of Metallurgical Science and Engineering, Central South University, Changsha 410083, China
? Central South University Press and Springer-Verlag Berlin Heidelberg 2012
Abstract: Cu2O particles with different shapes were prepared via reducing Cu(II) in alkaline system by glucose at 50 ℃. The products were characterized by X-ray diffraction (XRD), scanning electron microscopy (SEM) and transmission electron microscopy (TEM). It is found that the shape of Cu2O particles changes with the change of concentration of NaOH. The different shapes of Cu2O particles are due to the absorption of OH- ions on Cu2O particles, which arise the variety of growth mode of Cu2O, and then influence the morphology of Cu2O particles.
Key words: cuprous oxide; glucose reduction; morphology control; growth mechanism
1 Introduction
Cu2O is widely used as additive of corrosion-proof coating, colorant of glass and bactericide. Cu2O is a p-type semiconductor with a small band gap of about 2 eV [1], which makes it a promising material in the application of solar energy conversion, electronics, magnetic storage and catalysis [2-6]. Recently, some researches were carried out on the copper powder for electronic pastes prepared by H2 reduction of Cu2O as the precursor [7-8]. In this method, the shape and size of copper powder particles were determined by Cu2O. Since the parameters, such as particle size and shape of copper particles are utmost important for conductive pastes, it is essential to investigate the morphology and size control of the Cu2O particle. Many efforts have been devoted to the synthesis of Cu2O with various morphologies such as octahedron [9-12], cube [13-17], sphere [10, 16, 18], star-like, flower-like [19] and tubular like [13].
However, most of the methods utilize organic additive as modifier [10, 13], irradiation of microwave [15, 18], or water-in-oil microemulsions [14]. Those processes are inconvenient and complex. In this work, Cu2O particles were prepared via reducing Cu(II) by glucose in alkaline system. Different shapes and crystallinities of Cu2O particles were obtained through changing the concentration of NaOH without any assistant equipment or reagents. The synthesis can be well repeated in high yield and satisfy the demand of industrialization. Furthermore, mechanism concerning morphology control was analyzed and discussed. The reaction condition of preparing spherical Cu2O particles for producing copper powder used on electronic pastes was discovered.
2 Experimental
2.1 Materials
Copper sulphate (CuSO4·5H2O), sodium hydroxide (NaOH), glucose (C6H12O6·H2O) and solvents were of analytical grade and were used as received without further purification.
2.2 Preparation of Cu2O particles
In different sequences of experiments, the volumes of CuSO4, NaOH and glucose solutions with definite concentration were kept constant at 200 mL, respectively, and the concentrations of CuSO4, NaOH and glucose were listed in Table 1.
Table 1 Reaction reagent concentration for preparation of Cu2O
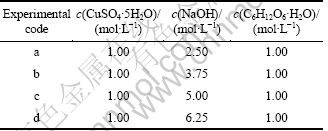
Firstly, the precursor was prepared by dropping
NaOH solution into CuSO4 solution under stirring in flask at room temperature (25 ℃). Secondly, the precursor and glucose solutions were heated up to 50 ℃, respectively. Finally, the glucose solution was dumped into the reaction vessel rapidly to reduce the precursor at 50 ℃. The products were collected by centrifugation, washed for 5 times with distilled water and 2 times with ethanol, and then, dried at 65 ℃ for 24 h. Preparation processes of precursor and Cu2O paste were both under stirring (500 r/min). The pH values were measured timely during the glucose reduction.
In the reaction, the Cu2+ ions are reduced first to Cu+ by glucose and Cu+ can react with OH- to form Cu2O, which is described as follows:
Cu(OH)2=Cu2++2OH- (or CuO+H2O=Cu2++2OH-) (1)
Cu2++3OH-+C6H12O6=Cu++
+2H2O (2)
Cu++OH-=CuOH (3)
2CuOH=Cu2O+H2O (4)
2.3 Measurement of quantities of OH- absorbed on Cu2O particles
Firstly, 4 g Cu2O particles were put into 200 mL NaOH solution. Secondly, the suspension was stirred by ultrasonication for 30 min, and then the suspension was filtrated using 0.45 μm polyether sulfone membrane filter. Finally, the concentration of OH- was measured in the filtrate by acid-base titration.
2.4 Characterization of Cu2O particles
The XRD of the powder was carried out using a Rigaku D/Max 2550 X-ray diffractometer with Cu Kα radiation (λ=1.541 8 ?). The morphology and particle size of Cu2O particles were investigated by SEM (JSEM-6360LV, Japan). TEM images were taken with a Hitachi Model-800 transmission electron microscope using an accelerating voltage of 200 kV.
3 Results and discussion
3.1 Characterization of Cu2O particles
The XRD patterns of products with octahedron and spherical shapes are shown in Fig. 1. All the peaks can be indexed into the cubic symmetry of Cu2O. No impurity peaks are observed, indicating that the products are phase-pure Cu2O.
Cu2O powders obtained from different experiments are characterized by SEM, as shown in Fig. 2. It can be deduced that spherical Cu2O particles are synthesized when the concentration of NaOH is 2.50 mol/L. As the concentration of NaOH increases, quasi-spherical Cu2O particles with angularity appear (Fig. 2(c)). When the NaOH concentration is 6.25 mol/L, the shape of Cu2O particle is octahedron.
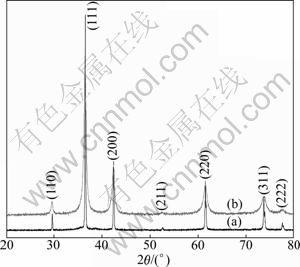
Fig. 1 XRD patterns of Cu2O with different shapes: (a) Octahedron; (b) Sphere
The TEM micrographs and the selected area electron diffraction (SAED) patterns of Cu2O particles with different shapes are provided in Fig. 3. Figure 3(a) shows the TEM and SAED micrographs of the spherical Cu2O particles when the NaOH concentration is 2.50 mol/L. The TEM images clearly indicate that the spherical particles are all polycrystals, as indicated by the ring-like SAED pattern taken on these spherical particles. Figure 3(b) shows the TEM and SAED micrographs of the octahedral Cu2O particles when the NaOH concentration is 6.25 mol/L. The TEM image unambiguously reveals that it is a perfect Cu2O single crystal, and the SAED pattern can be identified as the á111? zone axis projection of the Cu2O reciprocal lattice. Therefore, both single crystal particles and polycrystalline powders can be prepared selectively in similar procedures by simply changing the concentration of NaOH.
3.2 Discussion
Two completely different mechanisms are usually used to explain the formation of single crystals and polycrystals [20]. A diffusion mechanism is generally accepted to explain the formation of monodispersed single crystals. According to this mechanism, the crystal nuclei will make a facet oriented growth via the constituent complexes diffusion to these primary particles, and the morphology of monocrystal is determined by the relatively growth rate of different crystal faces [12, 20-21]. For Cu2O monocrystal, when (111) plane grows relative fast, the shape of Cu2O crystal tends to be cube. When (100) plane grows relatively fast, it tends to be octahedron. On the other hand, an aggregation mechanism is also employed to explain the formation of monodispersed polycrystals [10, 20]. This mechanism considers that the aggregations of uniform single crystalline subunits lead to nuclei growing into the final larger polycrystalline assemblies.
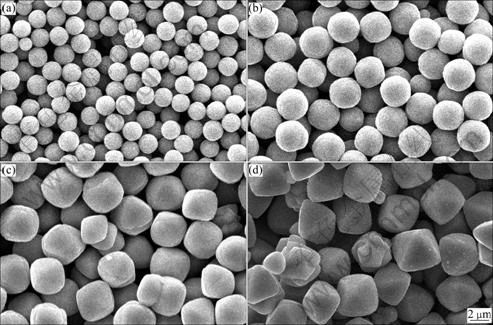
Fig. 2 SEM images of Cu2O particles prepared in presence of different concentrations of NaOH: (a) 2.50 mol/L; (b) 3.75 mol/L; (c) 5.00 mol/L; (d) 6.25 mol/L
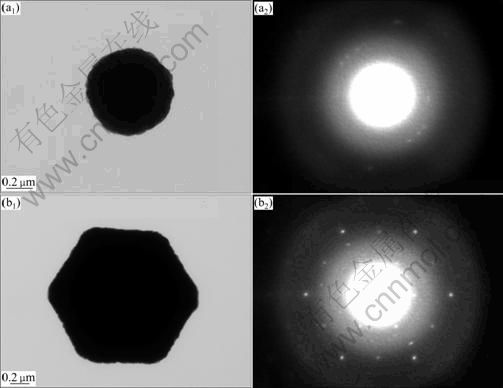
Fig. 3 TEM and SAED images of Cu2O particles with different shapes: (a) sphere; (b) octahedron
It could be concluded that spherical Cu2O polycrystals were obtained in this work because the growth mode of Cu2O nuclei was aggregation, and octahedral Cu2O single crystals were owing to the growth mode of (100) facet oriented growth. In this work, different shapes of Cu2O crystal particles were obtained via changing the concentration of NaOH. The result indicates that the competition exists between aggregation growth and (100) facet oriented growth of crystal nucleus when the concentration of NaOH changes. It could be attributed to having a large number of OH- on Cu2O particles surface in alkaline systems.
In order to study the effect of OH- on growth mode of Cu2O crystal nucleus, the pH values were registered timely during glucose reduction of Cu(II), as shown in Fig. 4. And the absorption quantities of OH- ions on Cu2O particles at different pH values (NaOH solution with different concentrations) are listed in Table 2.
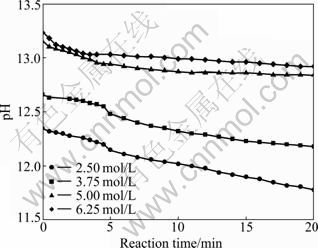
Fig. 4 Change of pH values during reaction in presence of different NaOH concentrations
Table 2 Absorption quantities of OH- on Cu2O in different alkaline systems
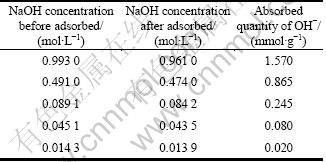
In this work, every reaction finished after 20 min. It can be seen in Fig. 4 that when NaOH concentration is 2.50, 3.75, 5.00 and 6.25 mol/L, the pH values change from 12.35 to 11.80, 12.65 to 12.18, 13.2 to 12.8 and 13.2 to 12.9 in reaction process, respectively. Table 2 shows that there is a strong adsorption between Cu2O particles and OH-, and the adsorption increases with the increase of NaOH concentration (pH value). It could be concluded that when NaOH concentrations are 2.50 mol/L and 3.75 mol/L, the absorbed quantity of OH- ions on Cu2O particles in the reaction process is less than 0.245 mmol/g, but when NaOH concentrations are 5.00 mol/L and 6.25 mol/L, it is more. Large number of OH- adsorbed on surface of Cu2O particles will arouse strong repulsion among them. The repulsion will become stronger while more OH- ions are adsorbed. So, in this work, different shapes of Cu2O crystal particles would be due to the following effects:
1) When the concentration level of NaOH is low, there are less OH- ions adsorbed on surface of Cu2O particles. Thus, repulsion between single-nuclei, molecule clusters, and primary particles is low, aggregation is the leading growth mode, and the crystal nucleus grow into spherical particles finally.
2) When the concentration level of NaOH is high, there are more OH- ions adsorbed on the surface of Cu2O particles. This brings about stronger repulsion among primary particles, which restrains the aggregation growth. Thus, diffusion mechanism is the growth mode of Cu2O crystal nuclei. As to this work, it is accepted that the OH- would be selectively adsorbed on (111) facet of Cu2O crystals, then slow the growth rates of (111) facet [12]. The increase of NaOH concentration will augment the adsorption of OH- on Cu2O particle, in especial, on (111) crystal facet. So, the nuclei tend to preferentially grow along (100) directions and form octahedrons bounded by (111) facets.
4 Conclusions
1) When NaOH concentration is 6.25 mol/L, there are more OH- ions adsorbed on surface of Cu2O particles, and the repulsion among primary particles restrains aggregation growth mode and high density of OH- on (111) facet restrains the growth of (111) facet. As a result, relatively faster growing of (100) facet is eliminated, with morphology of Cu2O forming into octahedron finally.
2) When NaOH concentrations are 2.50 mol/L and 3.75 mol/L, the repulsion is lower, aggregation is the leading growth mode, and Cu2O nuclei grow into sphere finally.
3) When NaOH concentration is 5.00 mol/L, there is a balanced competition between aggregation growth and (100) facet growth. As a result, Cu2O nuclei grow into quasi-spherical particles.
References
[1] SMITH M, GOTOVAC V, ALJNOVIC L J. LUCIC-LAVCEVIC M. An investigation of the electrochemical and photo-electrochemical properties of the cuprous oxide/liquid phase boundary [J]. Surface Science, 1995, 335: 171-176.
[2] JAYEWARDENA C, HEWAPARAMA K P, WIJEWARDENA D L A, GURUGE H. Fabrication of n-Cu2O electrodes with higher energy conversion efficiency in a photo-electrochemical cell [J]. Solar Energy Materials & Solar Cells, 1998, 56(1): 29-33.
[3] WANG Xue-jun, LOU Tao. Photo degradation of dissolved organic matter and its impact on the biologic processes [J]. Photographic Science and Photochemistry, 2004, 22(4): 29-305.
[4] NIAN Jun-nan, HU Che-chia, TENG His-sheng. Electrodeposited p-type Cu2O for H2 evolution from photoelectrolysis of water under visible light illumination [J]. International Journal of Hydrogen Energy, 2008, 33(12): 2897-2903.
[5] KAKUTA S, TOSHIYUKI A. Photocatalytic activity of Cu2O nanoparticles prepared through novel synthesis method of precursor reduction in the presence of thiosulfate [J]. Solid State Sciences, 2009, 11(8): 1465-1469.
[6] WEI Xiao-hao, ZHU Hai-tao, KONG Tian-tian, WANG Li-qiu. Synthesis and thermal conductivity of Cu2O nanofluids [J]. International Journal of Heat and Mass Transfer, 2009, 52(19/20): 4371-4374.
[7] HU Min-yi, WANG Chong-guo, ZHOU Kang-gen, XU Rui. Preparation of spherical ultrafine copper powder [J]. Functional Material, 2007, 38(10): 1577-1579. (inChinese)
[8] ZHOU Kang-gen, WANG Yue-jun, CAO Yan, JIANG Zhi-gang. A novel process for the production of copper powder used on electronic paste: China, ZL 200910043043.7 [P]. 2009-08-26. (inChinese)
[9] ZHANG Xu, XIE Yi, XU Fen, LIU Xiao-hui, XU Di. Shape- controlled synthesis of submicro-sized cuprous oxide octahedral [J]. Inorganic Chemistry Communications, 2003, 6(11): 1390-1392.
[10] DONG Ya-jie, LI Ya-dong, WANG Cheng, CUI Ai-li, DENG Zhao-xiang. Preparation of cuprous oxide particles of different crystallinity [J]. Journal of Colloid and Interface Science, 2001, 243(1): 85-89.
[11] ZHANG Xiao-jun, WANG Guang-feng, WU Hai-bian, ZHANG Xiao-qing, LI Po, WU Hua-qiang. Synthesis and photocatalytic characterization of porous cuprous oxide octahedral [J]. Materials Letters, 2008, 62(28): 4363-4365.
[12] ZHANG Xiao-juan, CUI Zuo-lin. One-pot growth of Cu2O concave octahedron microcrystal in alkaline solution [J]. Materials Science and Engineering B, 2009, 162(2): 82-86.
[13] LUO Fang, WU Di, GAO Lei, LIAN Suo-yuan, WANG En-bo, KANG Zhen-hui, LAN Yang, XU Lin. Shape-controlled synthesis of Cu2O nanocrystals assisted by Triton X-100 [J]. Journal of Crystal Growth, 2005, 285(4): 534-540.
[14] CHEN Qing-de, SHEN Xing-hai, GAO Hong-cheng. Formation of solid and hollow cuprous oxide nanocubes in water-in-oil microemulsions controlled by the yield of hydrated electrons [J]. Journal of Colloid and Interface Science, 2007, 312(2): 272-278.
[15] YANG Shi-guo, CHEN Qing-de, SHEN Xing-hai. The effect of ethylene glycol on the morphology of Cu2O nanoparticles synthesized in W/O microemulsion by γ-irradiation [J]. Spectroscopy and Spectral Analysis, 2007, 27(11): 2155-2159.
[16] ZHAO Hua-tao, WANG Dong, ZHANG Lan-yue, BAI Zhi-ping, WU You-ting. A simple method for the preparation of Cu2O with different morphologies in high reaction concentration [J]. Chinese Journal of Inorganic Chemistry, 2009, 25(1): 142-146. (in Chinese)
[17] SUN Fang, GUO Yu-peng, TIAN Yu-mei, ZHANG Ji-dong, LV Xiao-tang, LI Ming-gang, ZHENG Yun-hui, WANG Zi-chen. The effect of additives on the Cu2O crystal morphology in acetate bath by electrodeposition [J]. Journal of Crystal Growth, 2008, 310(2): 318-323.
[18] WU Zheng-cui, SHAO Ming-wang, ZHANG Wu, NI You-bao. Large-scale synthesis of uniform Cu2O stellar crystals via microwave-assisted route [J]. Journal of Crystal Growth, 2004, 260(3/4): 490-493.
[19] LIANG Zhen-hua, ZHU Ying-jie. Synthesis of uniformly sized Cu2O crystals with star-like and flower-like morphologies [J]. Materials Letters, 2005, 59(19/20): 2423-2425.
[20] SUGIMOTO T. Monodispersed particles [M]. Amsterdam: Elsevier Science B.V., 2001: 86-140.
[21] RAWLINGS J B, MILLER S M, WITKOWSKI W R. Model identification and control solution crystallization processes: A review [J]. Industrial & Engineering Chemistry Research, 1993, 32(7): 1275-1296.
(Edited by HE Yun-bin)
Foundation item: Project(50674100) supported by the National Nature Science Foundation of China
Received date: 2011-05-27; Accepted date: 2012-03-22
Corresponding author: ZHOU Kang-gen, Professor, PhD; Tel: +86-731-88836442; E-mail: zhoukg63@yahoo.com.cn