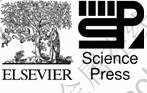
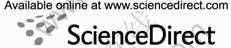
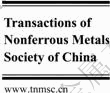
Trans. Nonferrous Met. Soc. China 22(2012) 534-538
Isothermal oxidation behavior of single crystal superalloy DD6
SHI Zhen-xue, LI Jia-rong, LIU Shi-zhong
Science and Technology on Advanced High Temperature Structural Materials Laboratory,
Beijing Institute of Aeronautical Materials, Beijing 100095, China
Received 10 January 2011; accepted 20 December 2011
Abstract: The isothermal oxidation behavior of the second generation single crystal superalloy DD6 was studied at 1050 °C and 1100 °C in ambient atmosphere. Morphology of oxides was examined by SEM and their composition was analyzed by XRD and EDS. The experimental results show that DD6 alloy obeys subparabolic rate law during oxidation of 100 h at 1050 °C and 1100 °C. The oxide scale exposed at 1050 °C is made up of an outer NiO layer with a small amount of Al2O3 and an inner Al2O3 layer. The oxide scale exposed at 1100 °C is made up of an outer Al2O3 layer with a small amount of NiO, an intermediate layer, mainly composed of Cr2O3 and TaO2, and an inner Al2O3 layer. The γ′-free layer was formed under the oxide scale at two temperatures.
Key words: single crystal superalloy; DD6 alloy; high temperature oxidation
1 Introduction
Nickel-base single crystal superalloys have been widely used as turbine blade materials for advanced aircraft engines, owing to their superior performance which includes both mechanical strength and oxidation resistance. At high temperature, the oxidation resistance behavior of the materials becomes one of the major life-limiting factors [1]. It is usual to protect the alloy using coating technology. However, for various reasons, it is not always possible to apply coating to the superalloys [2]. And it is necessary to know the intrinsic behavior of the substrate in oxidizing atmosphere. Therefore study on the oxidation behavior of single crystal superalloys becomes important. There have been several studies on the oxidation behavior of single crystal superalloys in recent years [3-7].
DD6 alloy was developed for aeroengine turbine blade applications by Beijing Institute of Aeronautical Materials, China. The properties of the alloy are equivalent to and more than those of other second generation single crystal superalloys widely used in the world [8]. DD6 alloy has an approximate 40 °C improvement of creep strength relative to the first generation single crystal superalloy DD3 and can be made into complex shaped hollow turbine blades which work under 1100 °C [9]. In this study, the high temperature oxidation behavior of DD6 alloy was investigated at 1050 °C and 1100 °C. Post-oxidation investigations were carried out to characterize the microstructures and compositions in the oxide scales.
2 Experimental
The nominal chemical compositions of DD6 alloy are shown in Table 1. The single crystal superalloy rods with [001] orientation were cast by means of crystal selection method in a directionally solidified furnace with high temperature gradient. The heat treatment of the specimens was carried out according to following heat treatment regime: (1290 °C, 1 h)+(1300 °C, 2 h)+(1315 °C, 4 h, AC)+(1120 °C, 4 h, AC)+(870 °C, 32 h, AC). The square specimens with the dimensions of 30 mm× 10 mm×1.5 mm were machined from the alloy rod by an electro-spark machine. The surface of the specimens was ground with SiC abrasive paper (1000 grid), and ultrasonically cleaned in acetone and ethanol, respectively.
The isothermal oxidation tests were conducted at 1050 °C and 1100 °C in static air. During the oxidation the specimens were cooled to room temperature at an interval of 25 h for mass measurement by an electrobalance with the precision of 0.1 mg. Three specimens were used to obtain the average of the mass gain. The surface and cross sectional morphology of oxides were examined by scanning electron microscopy with energy dispersive X-ray analysis (SEM/EDX) and their phase composition was analyzed by X-ray diffraction (XRD).
Table 1 Nominal chemical compositions of DD6 alloy (mass fraction, %)

3 Results and discussion
3.1 Oxidation kinetics
The isothermal oxidation kinetics of DD6 alloy at 1050 °C and 1100 °C is shown in Fig.1. The results show that oxidation resistance of the alloy at 1050 °C is better than at 1100 °C. It can be seen that the oxidation speeds up in initial period of 25 h. This is the formation period of oxide film. Then, the oxidation speeds down, and enters the oxide film growth period. During the oxidation process, a surface reaction changes into diffusion gradually. The kinetics can be described by examining the growth rate—time constant, which is found as the exponent in the following rate equation [10]:
(Δm/A)=Ktn+C (1)
where Δm is the mass change, A is the specimen surface area, K is the oxidation rate constant, t is the exposure time, and C is a constant. The slope of the lg—lg plot of the above equation gives the n value, which provides an indication of the oxidation mechanism. The n values of about 0.21 and 0.26 were obtained for the alloy oxidation at 1050 °C and 1100 °C, respectively, indicating a subparabolic time dependence of the growth rate. Similar oxidation kinetics has been reported for other single crystal superalloys [1, 10].
3.2 Phase constitution of oxidation layer
Figure 2 presents the XRD patterns of the surface of DD6 alloy after 100 h oxidation at 1050 °C and 1100 °C, respectively. After oxidation for 100 h at 1050 °C, the oxidation products formed on the alloy are mainly NiO, Al2O3, CoO and TaO. After oxidation for 100 h at 1100 °C, the oxidation products formed on the alloy are mainly NiO, Al2O3, Cr2O3, Co3O4 and TaO2. Compared with the oxidation products at 1050 °C, the Cr2O3 is detected and the TaO2 and Co3O4 are in place of TaO and CoO in the oxide layer at 1100 °C.
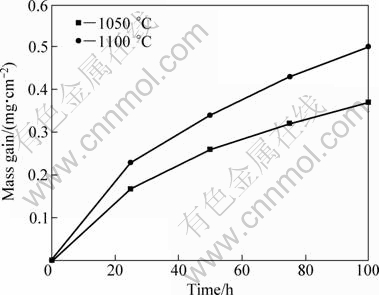
Fig. 1 Isothermal oxidation kinetics curves of DD6 alloy samples at 1050 °C and 1100 °C in air
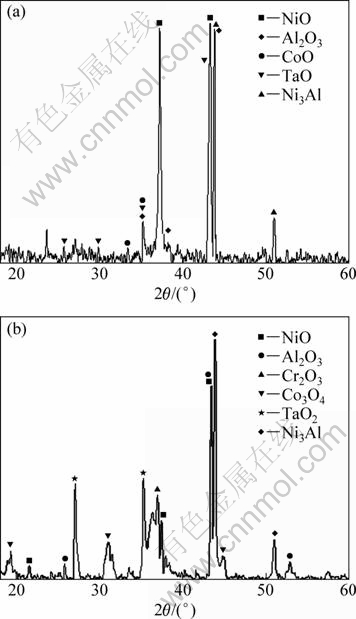
Fig. 2 XRD patterns of DD6 alloy samples after oxidation for 100 h at different temperatures: (a) 1050 °C; (b) 1100 °C
3.3 Morphologies of oxide scale
Figure 3 shows SEM images of surface of DD6 alloy samples oxidized for 100 h at 1050 °C and 1100 °C. It can be seen that the surface morphology of the scale shows considerable difference at different temperatures. The blocky morphology of oxides at 1050 °C tightly piles each other on the surface. No spallation of the scale is observed on the surface. The fine flaky oxides at 1100 °C loosely pile each other. And several cavities are clearly visible. Locally, a little spallation is found on the oxide scale. The compositions of the oxide layer measured by EDS are shown in Fig. 4. According to EDX analysis, O, Ni, Al, Co and Ta are the major constituents of oxide layers at 1050 °C and 1100 °C. This is in accordance with the XRD results.
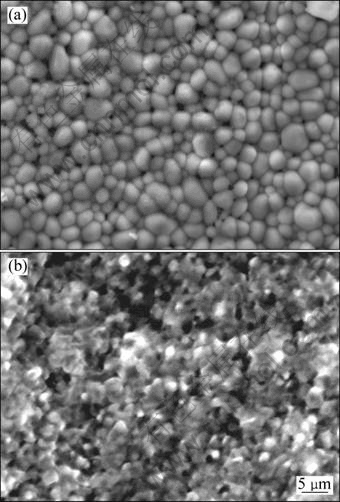
Fig. 3 SEM images of surface of DD6 alloy samples oxidized for 100 h at different temperatures: (a) 1050 °C; (b) 1100 °C
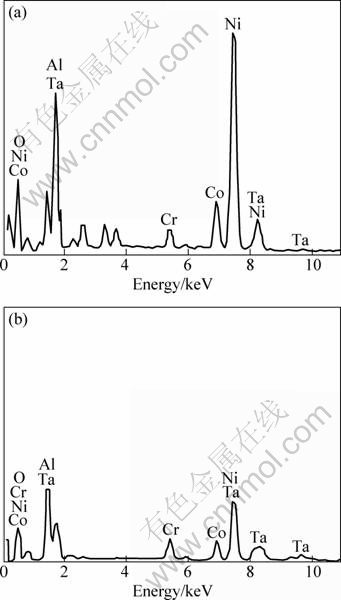
Fig. 4 EDX analysis of oxide layer at different temperatures: (a) 1050 °C; (b) 1100 °C
Figure 5 displays the cross-sectional morphologies of oxide layers at 1050 °C and 1100 °C. It can be seen that the higher the temperature, the thicker the oxide layer. At 1050 °C, a thin and continuous oxidation layer forms. Its thickness is 5 μm averagely. At 1100 °C, the average thickness of the layer is about 8 μm. Therefore, the oxidation temperature is a key factor to affect the thickness of the oxide scale. With increasing the temperature, the inward diffusion rate of oxygen and outward diffusion rate of alloy elements are increased. The oxide layer at 1050 °C consists of two layers. However, the oxide layer at 1100 °C consists of three layers. Table 2 summarizes the compositions of different layers measured by EDS. This, together with the XRD results, suggests that the oxide scale at 1050 °C is made up of an outer NiO layer with a small amount of Al2O3 and an inner Al2O3 layer. The oxide scale at 1100 °C is made up of an outer Al2O3 layer with a small amount of NiO, an intermediate layer, mainly composed of Cr2O3 and TaO2, and an inner Al2O3 layer. Some pores are seen in the oxide layer at 1100 °C. SINHAROY and NARASIMHAN [11] indicated that the stresses generated by oxide growth and thermal mismatch can be relieved by void formation. Sub-surface voids may also be produced by evaporation of high vapor pressure alloying elements such as Mo [12].
The establishment of a continuous inner Al2O3 layer after the initial stage provides a diffusion barrier to the reactive species. So, the overall oxidation speeds down and a low oxidation rate is obtained, as shown in Fig. 1. The results and observations of the present study are in agreement with other investigation [13,14].
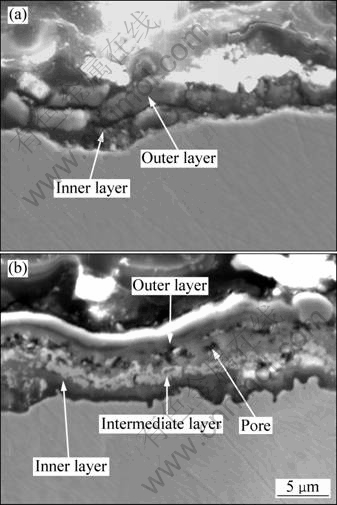
Fig. 5 Cross-sectional SEM images of samples oxidized for 100 h at different temperatures: (a) 1050 °C; (b) 1100 °C
Table 2 Compositions of oxide layers after oxidation for 100 h at 1050 °C and 1100 °C obtained by EDS

Further examination of the cross-sections after chemical etching reveals the development of a γ′-free layer under the oxide scale, as shown in Fig. 6. It is obvious that impoverishment of Al in this layer results from the outward diffusion driven by its own concentration gradient that was induced by the formations of Al2O3. The depletion of Al causes the transformation of γ′ to γ under the oxide scale [15,16]. The width values of this zone, measured after 100 h oxidation at 1050 °C and 1100 °C, are about 5 μm and 12 μm, respectively. At greater depth in the alloy matrix, it is evident that the size of γ′ precipitates increases following exposure to the test temperature.
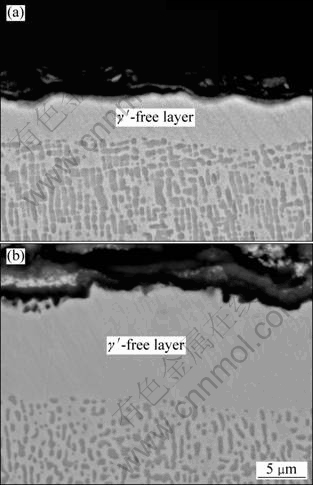
Fig. 6 Cross-sectional SEM images of samples after chemical etching at different temperatures: (a) 1050 °C; (b) 1100 °C
4 Conclusions
1) DD6 alloy obeys subparabolic rate law during oxidation of 100 h at 1050 °C and 1100 °C.
2) The oxide scale exposed at 1050 °C is made up of an outer NiO layer with a small amount of Al2O3, and an inner Al2O3 layer. The oxide scale exposed at 1100 °C is made up of an outer Al2O3 layer with a small amount of NiO, an intermediate layer, mainly composed of Cr2O3 and TaO2, and an inner Al2O3 layer.
3) Oxidation exposure of the alloy causes depletion of Al near the surface and dissolution of γ′ precipitates. Consequently, the γ′-free layer is formed under the oxide scale.
References
[1] HUANG L, SUN X F, GUAN H R, HU Z Q. Effect of rhenium addition on isothermal oxidation behavior of single crystal Ni-based superalloy [J]. Surface & Coating Technology, 2006, 200: 6863-6870.
[2] AKHTAR A, HOOK M S, REED R C. On the oxidation of the third-generation single crystal superalloy CMSX-10 [J]. Metallurgical and Materials Transactions A, 2005, 36: 3001-3017.
[3] NYCHKA J A, CLARKE D R, MEIER G H. Spallation and transient oxide growth on PWA1484 superalloy [J]. Materials Science and Engineering A, 2004, 490: 359-368.
[4] YOUNES C M, ALLEN G C, NICHOLSON J A. High temperature oxidation behaviour of single crystal superalloys RR3000 and CMSX-4 [J]. Corrosion Engineering, Science and Technology, 2007, 42(1): 80-88.
[5] FENG Q, TRYON B, CARROLL L J, POLLOCK T M. Cyclic oxidation of Ru-containing single crystal superalloys at 1100 °C [J]. Materials Science and Engineering A, 2007, 458: 184-194.
[6] KAWAGISHI K, SATO A, HARADA H, YEH A C, KOIZUMI Y, KOBAYASHI T. Oxidation resistant Ru containing Ni base single crystal superalloys [J]. Materials Science and Technology, 2009, 25(2): 271-275.
[7] RAFFAITIN A, MONCEAU D, ANDRIEU E, CRABOS F. Cycle oxidation of coated and coated single crystal nickel based superalloy MC2 analyzed by continuous thermogravimetry analysis [J]. Acta Materialia, 2006, 54: 4473-4487.
[8] LI J R, ZHONG Z G, TANG D Z, LIU S Z, WEI P, WU Z T, HUANG D, HAN M. A low-cost second generation single crystal superalloy DD6 [C]//POLLOCK T M, KISSINGER R D, BOWMAN R R, GREEN K A, MCLEAN M, OLSON S, SCHIRRA J J. Superalloys. Warrendale, PA: TMS, 2000: 777-783.
[9] The Editional Committee of China Aeronautical Materials Handbook. China aeronautical materials handbook (Vol.2) [M]. Beijing: China Standard Press, 2002: 812-818. (in Chinese)
[10] YING W, TOSHIO N. Oxidation behavior of the single crystal Ni-based superalloy at 900 °C in air and water vapor [J]. Surface & Coating Technology, 2007, 202: 140-145.
[11] SINHAROY S, NARASIMHAN S L. Oxidation behavior to two nickel-base superalloys used as elevated temperature valves in spark ignited engines and diesel exhaust recirculation (EGR) applications [C]//GREEN K A, POLLOK T M, HARADA H, HOWSON T W, REED R C, SCHIRRA J J, WALSTON S. Superalloys. Pennsylvania, PA: TMS, 2004: 623-626.
[12] PFENNIG A, FEDELICH B. Oxidation of single crystal PWA1483 at 950 °C in flowing air [J]. Corrosion Science, 2008, 50: 2482-2492.
[13] HUANG L, SUN X F, GUAN H R, HU Z Q. Oxidation behavior of single crystal Ni-based superalloy in air [J]. Oxidation of Metals, 2006, 65: 207-222.
[14] LIU C T, MA J, SUN X F. Oxidation behavior of a single crystal Ni-base superalloy between 900 and 1000 °C in air [J]. Journal of Alloys and Compounds, 2010, 491: 522-526.
[15] BENSCH M, PREUBNER J, HUTTNER R, OBIGODI G, VIRTANEN S, GABEL J, GLATZEL U. Modelling and anysis of the oxidation influence on creep behaviour of thin-walled structures of the single-crystal nickel-base superalloy RenéN5 at 980 °C [J]. Acta Materialia, 2010, 58: 1607-1617.
[16] TIAN Su-gui, ZHANG Lu-ting, YANG Hong-cai, HU Zhuang-qi, LI Tie-fan. Kinetics features of high temperature oxidation for a single crystal Ni-base superalloy [J]. Chinese Journal of Materials Research, 2002, 16(2): 183-187. ( in Chinese).
DD6单晶高温合金的等温氧化行为
史振学,李嘉荣,刘世忠
北京航空材料研究院 先进高温结构材料重点实验室,北京 100095
摘 要:研究第二代单晶高温合金DD6在1050 °C和1100 °C下的等温氧化行为,分别采用SEM、XRD和EDS对氧化产物进行分析。结果表明,在1050 °C和1100 °C温度下,DD6合金的100 h氧化动力学曲线遵循亚抛物线规律。在1050 °C时,氧化产物分为两层,外层为NiO和少量的Al2O3,内层为Al2O3。而在1100 °C时,氧化产物分为三层,外层为Al2O3和少量的NiO,中间层为Cr2O3和TaO2,内层由Al2O3组成。在这2种温度下的氧化物下面都形成了无γ′相的区域。
关键词:单晶高温合金;DD6合金;高温氧化
(Edited by LI Xiang-qun)
Corresponding author: SHI Zhen-xue; Tel: +86-10-62496338; Fax: +86-10-62496371; E-mail: shizhenxue@126.com
DOI: 10.1016/S1003-6326(11)61210-7