
Effect of T6I6 and its modified processes on mechanical properties of novel high strength Al-Li alloy-2A97
YUAN Zhi-shan(袁志山)1, 2, LU Zheng(陆 政)1, XIE You-hua(谢优华)1, WU Xiu-liang(吴秀亮)1,
DAI Sheng-long (戴圣龙)1, LIU Chang-sheng (刘常升)2
1. Beijing Institute of Aeronautical Materials, Beijing 100095, China;
2. School of Materials and Metallurgy, Northeastern University, Shenyang 110004, China
Received 28 July 2006; accepted 15 September 2006
Abstract: Based on a novel high strength Al-Cu-Li-X alloy-2A97, the effect of T6I6 and its modified processes on the properties investigated by SEM and tensile test. The results show that when the alloy is heat treated by triple ageing, with secondary low temperature ageing at 80 ℃ after initial ageing at 155 and 150 ℃, and final re-ageing at 135 and 165 ℃, the tensile properties are close to the peak level of aged alloy in T6 temper. The addition of plastic deformation after and prior to secondary ageing favor the T1(Al2CuLi) and δ′(Al3Li) precipitation during final re-ageing at 135 and 165 ℃ corresponding to triple ageing, so the Al-Li alloy displays higher strength for the modified processes of T6I6. The microstructures consist of δ′, T1 and θ″/θ′ (Al2Cu) phase for single and triple aged alloy, the number density and volume fraction of δ′ phase increase for T6I6 and its modified processes correspond to single ageing.
Key words: Al-Cu-Li-X alloy; 2A97; T6I6; triple ageing; microstructure
1 Introduction
Al-Li alloys were most often selected for aerospace components because of their low density, high strength and high specific modulus[1]. The results showed that highly saturated Al-Cu-Li series alloys yielded better balance of mechanical properties in T8 temper due to the precipitation of main strengthening T1 phase which nucleated on dislocations or dislocation loops introduced by plastic deformation[2-4], it was different from T6 temper which usually yielded low ductility and fracture toughness. Some multi-stage ageing treatments were designed to improve combination of strength and ductility of AF/C458(Al-1.8%Li) alloy and AF/C489(Al-2.1%Li) alloy in T6 temper, especially to increase the S-T tensile elongation, it was contributed to minimize the size of grain boundary precipitates T1 and width of δ′ and/or θ?/θ′ PFZ and to increase matrix precipitation of T1[5-7]. LUMLEY et al[8-9] employed T6I6 temper to improve the fracture toughness in a wide range of aluminum alloys, it was found that the secondary precipitation of GP zones or δ′ precipitated at 65 ℃ after initial elevated temperature ageing, facilitated the increase of tensile strength by 3%-14%(average value is 10%) in the alloys 2014, 7050, 6061, 6013 and 8090 for the T6I6 temper, usually had minimal effect on ductility, compared with equivalent properties obtained by conventional T6 temper. In present work, the T6I6 process was employed firstly to improve the strength and ductility relationship of 2A97 alloy, and the plastic deformation was introduced firstly into the T6I6 process prior to and after secondary ageing at 80 ℃, and the effect of second ageing, final re-ageing, as well as the plastic deformation prior to and after second ageing in modified processes of T6I6, on the properties of a novel high strength Al-Cu-Li-X alloy-2A97.
2 Experimental
The study was carried out on 30 mm hot rolled sheets of alloy-2A97. The samples were solution treated at 520 ℃ for 2 h and quenched into cold water, then some samples were single aged at 155 ℃ for 24, 36, and 48 h for tensile test to determine the under-aged condition, and some specimens were double aged at 150 ℃ for 12 h followed by ageing at 80 ℃ for 24, 60, 100 h to determine the response of tensile properties during secondary ageing.
The specimens for three-stage ageing were final re-aged at 135 and 165 ℃ for 36 h and 24 h, respectively, after initial ageing at 150 and 155 ℃ for 12 h and secondary ageing at 80 ℃ for 24 h. Prior to and after secondary ageing at 80 ℃, 4% or 4.8% cold plastic deformation were introduced into T6I6 temper. The process identifications are listed in Table 1. Cylinder samples for tensile test with strain rate of 1 mm/min were prepared from the sheets in transverse direction. The fracture surfaces were examined by SEM on a JSM 5600LV instrument.
3 Results
3.1 Single ageing treatment and double ageing treatment
Fig.1(a) shows the tensile properties of aged alloy at 155 ℃ for 24, 36 and 48 h. From Fig.1(a), alloy aged for 36 h displays peak strengths σb and σ0.2 are 500 MPa and 413 MPa, respectively. 2195 alloy ageing at 150 ℃ lower than 180 ℃[4], the alloy has lower strength. The results show that under-aged condition can be achieved at 150 and 155 ℃ for 12 h, which is chosen for initial ageing to accelerate the precipitation of matrix strengthening phase. For interrupted aged alloys under double ageing, they display hardness readings 50%-80% of those in the full T6 temper[8], since under-aged condition is technically interesting. Fig.1(b) shows curves of tensile properties of double aged alloy vs secondary ageing time. The peak strength at 80 ℃ for 60 h after initial ageing achieved is greater than under-aged alloy at 155 ℃ for 24 h, σb, σ0.2 and δ5 are 474 MPa, 323 MPa and 22.5%, respectively. Along curve of tensile properties vs secondary ageing time, it finds the increase of tensile strength and elongation is slight, which is different from that of 2090 alloy aged to T6 temper followed by exposure at 70 ℃, suffering a loss of ductility and fracture toughness, but similar to the case in 1460 after lower temperature ageing following peak ageing[10].
Table 1 Values of σb and σ0.2 for triple ageing and modified processes to single aged at 155 ℃ for 24 h in last two columns
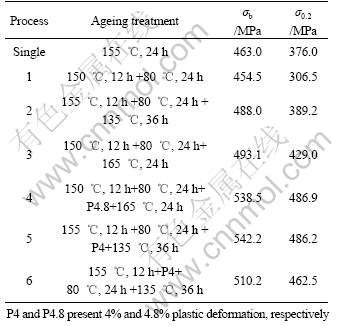
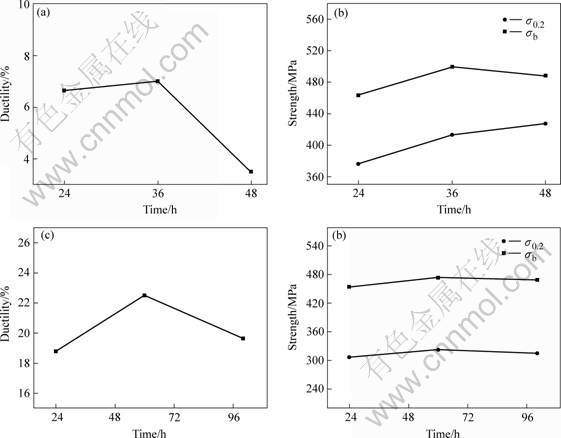
Fig.1 Relationship between tensile properties and time of alloys single aged at 155 ℃(a), (b) and double aged at 155 ℃(c), (d) for different time
3.2 Final re-ageing treatment and plastic deformation
Final re-ageing at 135 and 165 ℃ was employed to achieve the peak strength obtained for the T6 temper at 155 ℃, the results show in Fig.2. The strengths of 2A97 alloy treated by processes 2 and 3 in Table 1 increase significantly compared with the process 1, and process 3 produces higher strength than that of process 2. When plastic deformation was introduced into process 4 after secondary ageing, the increased value of σb and σ0.2 in strength are 54 and 97 MPa, respectively, corresponding to process 2. For process 6, σb and σ0.2 are 510 and 463 MPa, respectively, which is higher than process 2, but they are lower than that of process 5. The results indicate secondary ageing and plastic deformation prior to and after secondary ageing can lead to further strengthening in T6I6 and their modified processes. As shown in Table 1, the growth of σb and σ0.2 for triple ageing and their modified processes corresponding to single ageing at 155 ℃ are recorded. It is noted that the mechanical properties for alloys treated by triple ageing and their modified processes are close to or higher than the strength of peak aged alloy at 155 ℃ for 36 h.
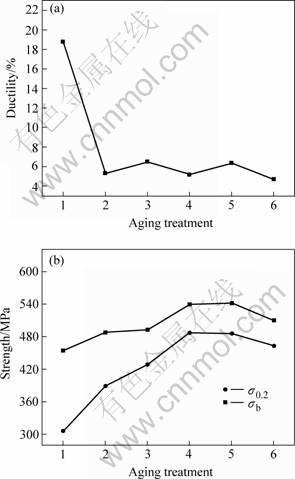
Fig.2 Relationship between tensile properties and aging treatment
3.3 SEM micrographs
Fig.3 shows SEM images of the tensile samples of processes 1 and 3 and its modified process 4, which indicate the dominance of intergranular failure and subintergranular failure. The SEM image in Fig.3(a) reveals some small areas of dimples for double aged alloy in under-aged temper are different from that treated by process 3 and its modified process 4, and the strength is close to the peak-aged alloy in T6 temper.
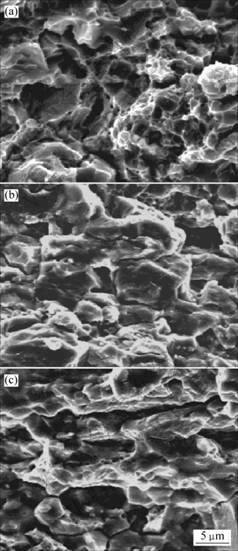
Fig.3 SEM images of fracture surfaces for alloys treated by double-ageing in process 1(a), triple-ageing in process 2(b) and its modified process in process 4(c)
4 Discussion
The investigation on ageing behavior and microstructure-property relationship during ageing at 155 ℃ for 2A97 alloy shows that the main strengthening phases include α-Al, δ′, θ″/θ′, T1 and β′(Al3Zr) phase of over-aged samples for 48 h at 155 ℃. For the under- aged condition at 155 ℃ for 12 h, the decomposition of supersaturated solid solution occurs partly accompanying by the homogeneous precipitation of δ′ and GP zones, θ″/θ′ at the matrix, and by the heterogeneous precipitation of T1 phase at the interface between α-Al and β′ or δ′/β′, the residual dislocations resulting from quenching and subgrain boundaries. TEM, X-ray and DSC results indicate that the volume fractions of δ′ and/or θ″/θ′ increase during secondary ageing[10-11] due to the formation of GP zones and/or δ′ phase when exposed at low temperature[12]. It can be concluded that, during secondary ageing at 80 ℃ for 24, 60 and 100 h, the ultrafine nucleus of δ′ and GP zones may continue to grow due to high driving force of phase transformation and high chemical driving force[3, 13] resulting from partial decomposition of supersaturated solid solution, for the same reason, a great number of clusters of solute atom nucleate during secondary ageing time. It points out that vacancies served as transformation media of diffusion of Li or Cu atoms for the growth of δ′ and GP zones at the early stage of their precipitation[4], and they aggregate and collapse to form clusters and dislocation voids or loops, which are considered to be nucleation sites for T1, and these vacancies accelerated the coarsening of δ′ and GP zones by speeding up the diffusion of Cu and Li atoms[4, 14]. The clusters of solute atom, fine δ′ and GP zones, and the dislocation loops form during secondary ageing strengthen the double aged alloy with secondary ageing at 80 ℃ for 100 h, the strength is higher than that of the under-aged alloy at 155 ℃ for 24 h.
When plastic deformation was introduced prior to and after secondary ageing, the dislocations are induced to form at the matrix. For the former, some dislocations interacted with fine particles transform to dislocations loops during secondary ageing. These dislocations and dislocation loops relate to plastic deformation, as well as clusters and particles, and the dislocation loops form during secondary ageing. Acted as nucleation sites, the precipitation of T1 and/or δ′ accelerates during final re-ageing, and this makes their tensile strengths treated by T6I6 and their modified processes be higher than those of single aged alloy. Upon final re-ageing at 165 ℃ for T6I6 and its modified process, particles of δ′, GP zones and T1 less than critical size reverse, and those are larger than critical size reserve and coarsen fast, the strength produce in process 3 is higher than that of process 2, but the strength of process 4 is lower than that of process 5. It is mentioned above that some dislocations interacted with fine particles transform to dislocations loops during secondary ageing, which make it decrease slightly for the number density of particles formed during final re-ageing of process 6, the strength of process 5 is lower than that of process 6.
It shows that coarsening of Li-/Cu-rich grain boundary precipitates, widening of δ′ or θ″/θ′ PFZs and segregation of lithium on grain boundaries embrittled the Al-Li alloys for single and double aged alloy[6, 14-15]. It seems that high strength and ductility for the alloy treated by T6I6 and their modified processes, especially for process 5 with low temperature final re-ageing, relate to the small size of δ′ or θ″/θ′ PFZ resulting from the under-aged double ageing, the strengthening of grain boundary resulting from plastic deformation during secondary ageing and coarsening of existence of δ′, θ″/θ′ and T1 and the formation of T1 and δ′ resulting from secondary ageing and plastic deformation prior to and after secondary ageing.
5 Conclusions
1) σb and σ0.2 and δ5 of 2A97 alloy treated by triple ageing with ageing at 80 ℃ for 24 h after initial ageing at 150 ℃ for 12 h are 493 MPa, 428.5 MPa and 6.5%, respectively, and the strengths of final re-ageing at 165 ℃ for 24 h are close to the peak strength of aged alloy in T6 temper.
2) Under addition of plastic deformation after initial ageing at 155 ℃ for 12 h and secondary aging at 80 ℃ for 24 h, the T1 and δ′ precipitation, during final re-ageing at 135 ℃ for 36 h correspond to relative triple ageing, so the Al-Li alloy 2A97 displays highest strength for the modified process of T6I6 with 4% plastic deformation, σb and σ0.2 and δ5 are 542 MPa, 486 MPa and 6.35%, respectively.
3) When the 4% plastic deformation was introduced prior to secondary ageing into the triple ageing, the increased values of σb and σ0.2 for this modified process of T6I6 are 32 MPa, 74 MPa, respectively corresponding to the relative triple ageing.
References
[1] KIELDING P S, WOLF G J. Aluminum-lithium alloy for aerospace[J]. Advanced Materials and Processes, 1996, 10: 21-23.
[2] HUANG B P, ZHENG Z Q, YIN D F, MO E M. Effects of trace Ag and Mg additions on mechanical properties and microstructures of 2195 alloy [J]. Materials Science Forum, 1996, 217/222: 1239-1244.
[3] GABLE B M, ZHU A W, CSONTOS A A, STARKE J E A. The role of plastic deformation on the competitive microstructural evolution and mechanical properties of a novel Al-Li-Cu-X alloy[J]. Journal of Light Metals, 2001, 1: 1-14.
[4] HUANG B P, ZHENG Z Q. Independent and combined roles of trace Mg and Ag additions of Al-Li-Cu-(Mg)-(Ag)-Zr-Ti alloys[J]. Acta Mater, 1998, 46: 4381-4393.
[5] CSONTOS A A, STAKE J E A. The effect of processing and microstructure development on the slip and fracture behavior of the 2.1wt. pct Li AF/C489 and 1.8 wt. pct Li AF/C458 Al-Li-Cu-X alloys[J]. Metallurgical and Materials Transactions A, 2000, 31: 1965-1975.
[6] ANANIEV U N. The influence of double-aging on the short transverse elongation of Al-Li-Cu-Zr rolled plate[J]. Materials Science Forum, 1996, 217/222: 865-870.
[7] PARRISH C, BARBA J, OH H M, PERAZA J, FOYOS J, LEE E W, ES-SAID O S. Alternate heat treatment of AF/C458 Al-Li alloy[J]. Materials Science Forum, 2000, 331/337: 655-662.
[8] LUMLEY R N, POLMEAR I J, MORTON A J. Interrupted aging and secondary precipitation in aluminum alloys[J]. Materials Science and Technology, 2003, 19: 1483-1490.
[9] LUNLEY R N, POLMEAR I J, MORTON A J. Control of secondary precipitation to improve the performance of aluminum alloys[J]. Materials Science Forum, 2002, 396/402: 893-898.
[10] DAVYDOV V G, FRIDLYANDER J N, SAMARINA M V. OROZOV L B, BER L B, YELAGIN V I, LANG R, PFANNENMULLER T. The heat treatment of Al-Li-Cu alloys ensured the stability of structure and properties at long low temperature exposure[J]. Materials Science Forum, 2000, 331/337: 1049-1054.
[11] STARINK M J, HOBSON A J, SINCLAIR I, GREGSON P J. Embrittlement of Al-Li-Cu-Mg alloys at slightly elevated temperatures: Microtructural mechanisms of hardening[J]. Materials Science and Engineering A, 2000, 289: 130-142.
[12] KERR M J, SWEET E D, BENNETT C G, MUDDLE B C. Effect of Alkali metal impurities of 2090 Al-Cu-Li alloys[J]. Materials Science Forum, 1996, 217/222: 1079-1084.
[13] NOBLE B, THOMPSON G E. T1(Al2CuLi) precipitation in Aluminum-Copper-Lithium alloys[J]. Metal Science Journal, 1972, 6: 167-174.
[14] PITCHER P D, SEWART R J, GUPTA S, THOMSON J J. A study of reversion behaviour in 8090 alloys using small angle neutron scattering and transmission electron microscopy[J]. Scripta Metallurgica, 1992, 26: 511-516.
[15] LYNCH S P. Fracture of 8090 Al-Li plate Ⅰ: Short transverse fracture toughness[J]. Materials Science and Engineering A, 1991, 136: 25-43.
(Edited by LI Yan-hong)
Foundation item: Projects(2005CB623705) supported by Major State Basic Research and Development Program of China
Corresponding author: YUAN Zhi-shan; Tel: +86-10-62496381; E-mail: yuanzs@163.com