Trans. Nonferrous Met. Soc. China 23(2013) 2348-2353
Nickel composite plating with fly ash as inert particle
Viet Hue NGUYEN, Thi Anh Tuyet NGO, Hong Hanh PHAM, Ngoc Phong NGUYEN
Institute of Materials Science, 18 Hoang Quoc Viet, 10 000, Hanoi, Vietnam
Received 6 September 2012; accepted 8 January 2013
Abstract: The Ni/FA composite plating was realized by electrodeposition with fly ash (FA) as inert particles. The main compositions of FA are 72% SiO2 and 25% Al2O3 in the size of 3-7 μm. Electrodeposition was performed in Watts bath containing FA with concentrations of 5, 20, 50 g/L, current densities of 2 and 4 A/dm2, temperature of 50 °C and magnetic stirring of 250 r/min. Scanning electron microscope (SEM+EDX), electrochemical and mechanical technique were used to study morphology, composition and properties of coating. FA content in deposit is dependent on the FA concentration in solution, as well as the plating parameters. Since FA particles were incorporated in the coating, the mechanical and electrochemical properties of the coating were increased. The microhardness of Ni/FA composite plating reaches HV 430 in comparison with HV 198 of pure Ni coating. It was confirmed by electrochemical measurement that the corrosion resistance of Ni/FA composite coating was higher than that of pure Ni.
Key words: composite plating; fly ash; microhardness; corrosion resistance; inert particles
1 Introduction
Composite plating (CP) is a useful, low cost way to provide special surface properties, such as high hardness, abrasion and corrosion resistance, low friction coefficient to meet the requirements of advanced materials [1]. Recently, the composite plating has been extensively developed on both experimental and theoretical aspects [1-4]. It has been shown that the mechanical and chemical properties of composite coatings are dependent on type, shape size, and content of inert particles in the deposit [5-11].
Many types of inert particles of nano- to micro-scales were used for composite plating, particularly for Ni-composite plating, such as carbides (e.g., SiC, TiC, Cr3C2, WC, B4C, ZrC, HfC), oxides (e.g., Al2O3, SiO2, TiO2, Cr2O3, ZrO2, UO2, BeO), borides and nitrides (e.g., Cr3B2, TiB2, ZrB2, HfB2, BN, B4N, TiN, AlN), diamond, graphite, MoS2, polymers (e.g., polytetrafluoroethylene, polyethylene, polyvinyl chloride, acrylonitride-butadiene-styrene), metals (e.g., Cr, W) and others (e.g., glass, CaF2, BaSO4).
In this work, fabricating the Ni-composite plating with fly ash (FA) as inert particles was performed. FA is a byproduct of thermal electric plants. FA has been studied as an additive for improving mechanical and chemical properties of metallic alloys, particularly magnesium and aluminum. Inclusion of FA in metals alloys results in high abrasion, wear resistance and low friction coefficient [12,13].
The FA used in this study was a product made in-house at our Institute in the partition of 3-7 μm. Its main compositions were 72% SiO2, 25% Al2O3, <2% Fe2O3, trace of moisture and minerals. SiO2 (silica) is an inert particle that has been studied for composite plating [4,14,15]. However, as shown by SHRESTHA et al [15], SiO2 particles can hardly co-deposit with nickel in an aqueous nickel bath due to their strong hydration energy. Using stimulator or PC technique, the Ni/SiO2 composite plating was fabricated, but normally the content of SiO2 deposited in coating was less than 1%. In the presence of 150 g/L of SiO2 in plating solution, the hardness of coating was HV 410, in comparison with HV 210 of pure Ni coating [4]. Al2O3 (corundum) with the hardness of HV 2000-2300 was used to fabricate Ni/Al2O3 composite coating. With the inclusion of 0.8% Al2O3 in the Ni plating, the hardness of the coating reached HV 450 (more than 2 times higher than that of pure Ni) and abrasion resistance increases by 4 times [4,16-18].
This study described the coating procedure of Ni/FA composite on a copper/mild steel substrate. The hardness and corrosion resistance of the coatings were discussed in comparison with the Ni coating.
2 Experimental
2.1 Materials and chemicals
2.1.1 Materials
Fly ash (FA) was produced in-house by a special magnetic sifter procedure using the byproducts of the PhaLai electric plant. In order to obtain SiO2 and Al2O3, the ash was treated for 30 min in nitric acid to dissolve impurities. Treatment with nitric acid was also to activate the surface of SiO2 and Al2O3 particles. The compositions of FA powder after treatment are shown in Table 1.
Table 1 Composition of FA powder (mass fraction, %)

2.1.2 Chemicals
All plating chemicals (NiSO4, NiCl2, H3BO3, wetting reagent, brighteners, additives) for Ni Watts bath were supplied by INCO (Electroplating USA Company).
2.2 Sample preparation and characterization
Copper or mild steel substrate samples with the dimensions of 2 cm×5 cm×0.05 cm were cleaned with oil and grease, rinsed in water, then distilling water and finally activated in a solution of 10% H2SO4.
After surface cleaning, the samples were plated in the Watts bath. Its compositions are shown in Table 2. The work conditions were: pH=4, temperature of 50 °C, current density of 2-4 A/dm2, magnetic stirring of 250 r/min.
Table 2 Compositions of plating bath and working conditions
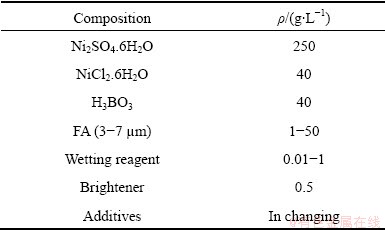
Plated samples with a minimum thickness of 20 μm were processed in metallographic procedures. Measure- ments of the thickness were conducted with an Axiover 40 MAT (Carl Zeiss), and the hardness was tested with IndentaMet 1106 (Buehler) in accordance with ASTM B578-87. The coating compositions were analyzed with SEM Jeol 6490-2300 in combination with EDX.
Electrochemical polarization curves were measured in 3.5% chloride solution by PARSTAT 2273 Potentio/ Gavanostat (USA). Traditional three-electrode cell was used. The working electrode made from composite plating sheet was mounted in epoxy resin with a working area of 1 cm2. Polarization measurement was used to calculate the corrosion (φcorr), passive (φpass), pitting (φpit) potentials, and corrosion (Jcorr), passive (Jpass), pitting (Jpit) current densities [19]. The polarization resistance (Rp) characterizing the corrosion behavior of metals was obtained by the method of STERN [20].
The amount of inert particles in the deposit was determined by two methods: 1) SEM/EDX with zone analysis technique to separately determine the amount of SiO2 and Al2O3, and 2) the gravimetric method. A stainless steel substrate was used. After plating, the coating layer was scraped, cleaned, dried, dissolved in 20% nitric acid over night, and then filtrated. The powder obtained was cleaned and dried in on oven at 110 °C, then cooled to room temperature in an exsiccator and weighed. By this procedure, the FA mass (i.e., total mass of SiO2 and Al2O3) in deposit was determined.
3 Results and discussion
3.1 Morphological study
Morphologies of Ni and Ni-composite plating were studied by SEM for morphological analyses as shown in Fig. 1. From this figure it was observed that the crystalline structure of Ni coating became finer in the presence of FA. At the FA concentration of 50 g/L, the plating crystalline obtained higher mechanical and electrochemical properties as shown later.
3.2 Content of inert particles in deposit
The micrograph and the EDX spectrum on the cross section of composite coating are shown in Fig. 2. From Fig. 2, it can be seen that FA particles were distributed in the deposit uniformly.
On the other hand, it is able to estimate the size of FA particles in the range of 3-7 μm. Therefore, the suggestion is that no aggregation of FA particles occurred in the plating solution as well as in the deposit. The thickness of coating estimated from this Fig. 2 was from 20 to 30 μm. This micrograph also showed the zone (frame of rectangle) where the contents of SiO2 and Al2O3 were separately determined.
Figure 3 shows the contents of SiO2 and Al2O3 with different FA concentrations in the plating bath. The total content of SiO2 and Al2O3 was also determined by gravimetric method and the results are shown on the third column of Fig. 3. Agreement of the results obtained by two methods is quite well. It can be seen that the contents of SiO2 and Al2O3 as well as their total in deposit are dependent on the FA concentrations in the plating bath (5-50 g/L) (Fig. 3) and on plating current density (2 and 4 A/dm2) (Fig. 4). The FA content in the deposit increased with increasing concentration in the plating bath and reached 12.5% at 50 g/L and J=2 A/dm2. The higher FA concentrations were not studied since the plating solution became too viscous and thus the quality of coating was poor. It was reported [7,14] that the content of SiO2 or Al2O3 in the deposit increased with increasing their concentration in solution. Also, it was noted that FA in the deposit decreased at a higher current density. When the FA concentration was 5 g/L, SiO2 content was 5% at J=2 A/dm2 and 3% at J=4 A/dm2; Al2O3 content was 3% at J=2 A/dm2 and 2.2% at J= 4 A/dm2, respectively.
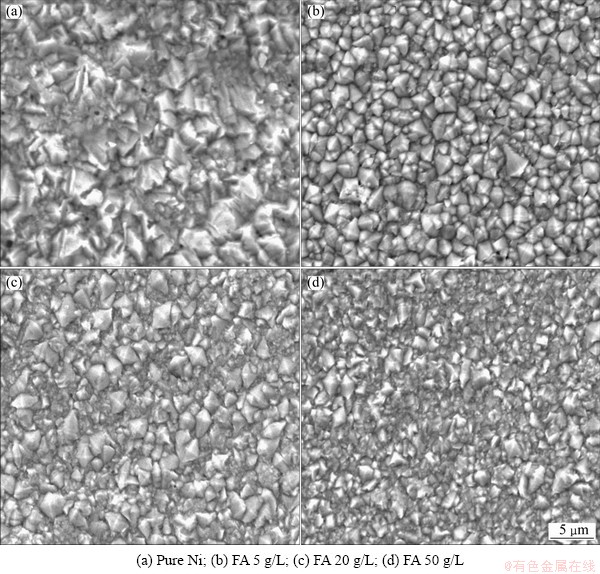
Fig. 1 SEM micrographs of pure Ni (a) and Ni composite coatings (b, c, d)
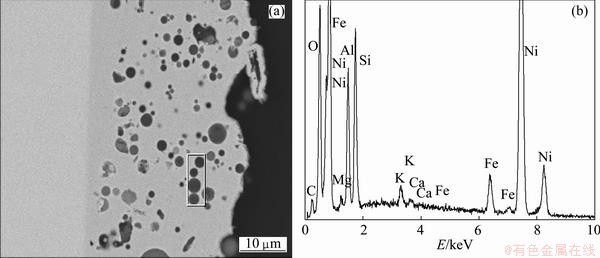
Fig. 2 SEM (a) and EDX spectrum (b) of Ni/FA composite sample
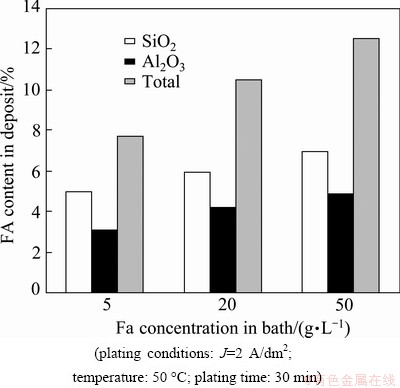
Fig. 3 Relationship between FA content in deposit and its concentration in plating bath
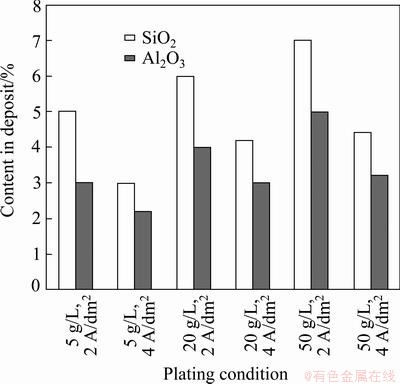
Fig. 4 Relationship between content of SiO2 and Al2O3 in deposit and different plating conditions
Based on Kurozaki’s model for particle-reinforced coatings involving a three-step process, SAHA et al [18] suggested that in the case of Ni/Al2O3 composite plating when electrodepositing at higher current densities, nickel ions dissolved from the anode bar and moved faster than the Al2O3 particles transported by the mechanical agitation and thus caused a low content of co-deposited Al2O3 particles in the coatings. Also, due to the increase of polarization caused by the higher current density (>2 A/dm2), more hydrogen was generated, leading to the decrease of particles incorporated in the coatings. On the other hand, at low current densities the nickel ions dissolved from the nickel bar (anode) moved slowly and there was not enough time for these ions to adsorb onto the particles. As a result, the Coulomb force between anions adsorbed on particles and the cathode became weak causing lower content of co-deposited Al2O3 particles in the coatings. This implied the existence of an optimum current density which would allow a maximum content of the co-deposited particles in the composite coatings. In Refs. [11,18,20-23] the optimum current density for the maximum co-deposition of inert particles was found to be 1-2 A/dm2. As indicated by our data above, the current density for a maximum content of the FA particles incorporated in the Ni composite plating process was 2 A/dm2.
3.3 Microhardness of coatings
As shown in Table 3, the microhardness of Ni/FA composite coating was higher than that of pure Ni. Increasing the FA concentration in solution, the microhardness of the composite coating increased due to an increased content of FA incorporated to deposit. In certain plating conditions, the microhardness of Ni/FA composite coating is more than 2 times higher than that of pure Ni. These microhardness values changed from HV 210 for pure Ni to HV 430 for the Ni composite coating at current density 2 A/dm2 and HV 198 to HV 410 at current density 4 A/dm2. The microhardness of coating increased with the content of FA particles in deposit due to 1) the presence of hard SiO2 and Al 2O3 particles, or 2) modification of microstructures of the coating due to the inclusion of FA towards to more refine microstructures of the Ni matrix [11,24-26].
Table 3 Microhardness of Ni/FA composite coating
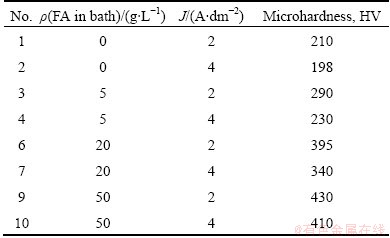
3.4 Electrochemical property of coatings
The representative anode polarization curves of Ni-composite coatings formed at different FA concentrations in solution are shown in Fig. 5(a) and at different plating current densities in Fig. 5(b). From these figures, the electrochemical parameters of Ni/FA composite coatings were calculated and listed in Table 4.
From Table 4, it is confirmed that FA concentration in solution, the corrosion potential, corrosion current and passive current of the composite coatings are more noble. For example, at the FA concentration of 50 g/L, φcorr/CP=-165 mV, Jcorr=0.03μA/cm2, Jpass=0.4μA/cm2 in comparison with corresponding values of φcorr= -190 mV, Jcorr=0.10 μA/cm2 and Jpass=1 μA/cm2 of pure Ni at J=2 A/dm2. Obviously, this is due to a higher content of the FA powder in the composite coating.
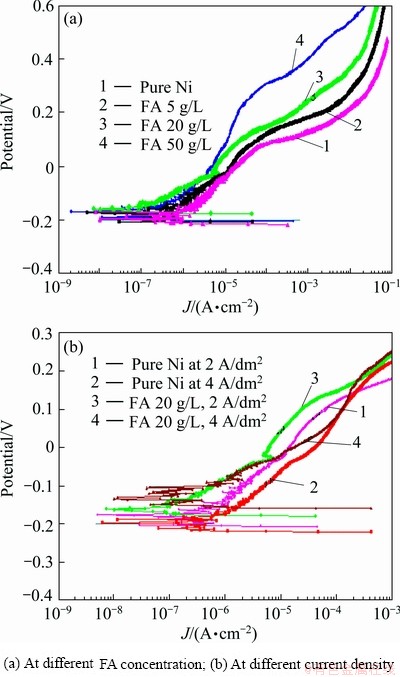
Fig. 5 Anode polarization curves of composite coatings at different concentrations of FA in solution under different plating conditions (temperature of 45 °C, potential scan rate of 0.5 mV/s, measurement solution of 3.5% NaCl)
The Ni/FA composite coating formed at lower current density exhibits a higher corrosion resistance. For example, for pure Ni plating at J=2 A/dm2: Jcorr=0.1 μA/cm2 and at J=4 A/dm2, Jcorr=0.6 μA/cm2 ; whereas, for Ni/FA composite plating at J=2 A/dm2: Jcorr=0.03 μA/cm2 and at J= 4 A/dm2, Jcorr=0.05 μA/cm2at FA concentration of 50 g/L.
Polarization resistance, Rp, calculated from linear polarization curves measurement shown in Table 4, exhibited the same tendency with other electrochemical parameters. In the case of a high content of FA particles in the deposit formed at lower current density, the polarization resistance was larger, indicating high corrosion resistance.
4 Conclusions
1) It is able to fabricate the Ni/composite coating with fly ash that is a micro-sized industry byproduct. The FA content in the deposit increases with increasing its concentration in the plating bath and reaches 12.5% when its concentration in the bath is 50 g/L and at current density of 2 A/dm2.
2) The mechanical/electrochemical properties of composite coating were improved with the increasing content of FA particles in the deposit, which was in turn dependent on the FA particle concentrations in solution and plating conditions. Microhardness of the Ni/FA composite coating is more than 2 times higher than that of pure Ni. Corrosion resistance of the composite increases by about 30 times and the passivity ability is 3 times in chloride solutions.
3) The Ni/FA composite coatings formed at a low current density (2 A/dm2) exhibit higher mechanical and electrochemical properties than at a high current density (4 A/dm2).
Acknowledgement
The authors would like to thank National Foundation for Science and Technology Development– NAFOSTED for the financial support provided by Contract, Coded: 104.03.117.09, Vietnam.
Table 4 Electrochemical parameters of Ni-composite coatings in 3.5 % solution
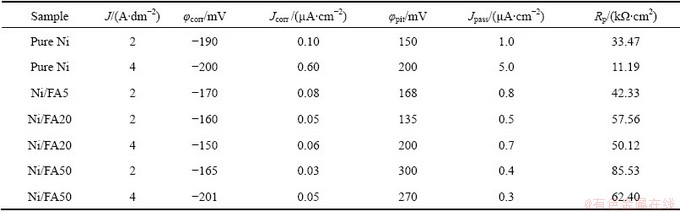
References
[1] HOVESTAD A, JANSSEN L J J. Electrochemical codeposition of inert particles in a metallic matrix [J]. Applied Electrochemistry, 1995, 25: 519-527.
[2] GUGLIELMI N. Kinetics of the deposition of inert particles from electrolytic baths [J]. Electrochemistry Society, 1972, 119: 1009-1015.
[3] CELIS J P, ROOS J R. Kinetics of the deposition of alumina particles from copper sulfate plating baths [J]. Electrochemistry Society, 1997, 124: 1508-1518.
[4] WIELAGE B, STEINHAUSER S, HENKER A. Manufacture and properties of composite coatings: An introduction [J]. Thermal Spray Technology, 1998, 8: 512-516.
[5] GARCIA I. Effect of the size and amount of reinforcing particles on the corrosion behavior of co-electrodeposited SiC-nickel composite coatings [C]//15th International Corrosion Conference. Granada, Spain, 2000: 672-676.
[6] LEE H K, LEE H Y, JEON J M. Codeposition of micro- and nano-sized SiC particles in the nickel matrix composite coatings obtained by electroplating [J]. Surface and Coatings Technology, 2007, 1: 4711-4717.
[7] LEE H K, LEE H Y, JEON J M. Electrolytic deposition behaviors of Ni-SiC composite coatings containing submicron-sized SiC particles [J]. Metals and Materials International, 2008, 14: 599-605.
[8] WANG S C, CHENG W, WEI J. Kinetics of electroplating process of nano-sized ceramic particle/Ni composite materials [J]. Chemistry and Physics, 2003, 8: 574-580.
[9] SHRESTHA N K, TAKEBE T, SAJI T. Effect of particle size on the co-deposition of diamond with nickel in presence of redox-active surfactant and mechanical property of the coating [J]. Diamond and Related Materials, 2006, 15: 1570-1575.
[10] LAMPKE T, LEOPOLD A, DIETRICH D. Correlation between structure and corrosion behavior of nickel dispersion coatings containing ceramic particles of different sizes [J]. Surface and Coating Technology, 2006, 201: 3510-3517.
[11] JEONG D H, GONZALEZ F, PALUMBO G., AUST K T, ERB U. The effect of grain size on the wear properties of electrodeposited nanocrystalline nickel coatings [J]. Scripta Materials, 2001, 44: 493-499.
[12] EMENIKE R O. Corrosion behaviour of fly ash-reinforced aluminum-magnesium alloy A535 composites [D]. Canada: University of Saskatchewan, 2008.
[13] HUANG Z Q, YU S R, LI M Q. Microstructures and compressive properties of AZ91D/fly-ash cenospheres composites [J]. Transactions of Nonferrous Metals Society of China, 2000, 20: 458-462.
[14] NOWAK P, SOCHA R P, KAISHEVA M, FRANSAER J, CELIS J P, STOINOV Z. Electrochemical investigation of the codeposition of SiC and SiO2 particles with nickel [J]. Applied Electrochemistry, 2000, 30: 429-437.
[15] SHRESTHA N K, KOBAYASHI G, SAJI T. Electrodeposition of hydrophobic nickel composite containing surface-modified SiO2 particles under the influence of a surfactant with an azobenzene moiety [J]. Chemistry Letters, 2004, 33: 207-214.
[16] BADARULZAMAN N A, PURWADARIA S, MOHAMAD A A, AHMAD Z A. The production of nickel-alumina composite coating via electroplating [J]. Ionics, 2009, 15: 603-607.
[17] CHEN L, WANG L P, ZENG Z X, XU T. Influence of pulse frequency on the microstructure and wear resistance of electrodeposited Ni–Al2O3 composite coatings [J]. Surface and Coatings Technology, 2006, 201: 599-605.
[18] SAHA R K, KHAN T I. Effect of applied current on the electrodeposited Ni–Al 2O3 composite coatings [J]. Surface and Coatings Technology, 2010, 205: 890-895.
[19] NGUYEN N P, NGUYEN V H, DO C L, NGO T A T, KWON S C, LEE J Y. An application of electrochemical method for studying nano-composite plating [J]. Metals and Materials International, 2006, 12: 493-498.
[20] STERN M. A method for determining corrosion rates from linear polarization data [J]. Corrosion, 1958, 9: 440-444.
[21] WANG C, ZHONG Y, REN W, LEI Z, REN Z, JIA J, JIANG A. Effects of parallel magneticfield on electrocodeposition behavior of Ni/nanoparticle composite electroplating [J]. Applied Surface Science, 2008, 254: 5649-5656.
[22] GUEL H, KILIC F, ASLAN S, ALP A, AKBULUT H. Characteristics of electro-co-deposited Ni–Al2O3 nano-particle reinforced metal matrix composite (MMC) coatings [J]. Wear, 2009, 267: 976-982.
[23] KIM S K, YOO H J. Formation of bilayer Ni–SiC composite coatings by electrodeposition [J]. Surface and Coatings Technology, 1998, 108-109: 564-570.
[24] PARK S Y, KIM R H, KIM J S, KIM C K. Composite coating of suspended inert particles in the rotating disk electrode [J]. Korean Surface Engineering, 1992, 25: 73-82.
[25] KIM M J, KIM J S, KIM D J, KIM H P. Electro-deposition of oxide-dispersed nickel composites and the behavior of their mechanical properties [J]. Metals and Materials International, 2009, 5: 789-795.
[26] NGUYEN V H, HOANG N P, NGUYEN N P, KWON S C, KIM M, LEE J Y. Cr/nanodiamond composite plating with cobalt cation additive [J]. Transactions of Nonferrous Metals Society of China, 2009, 19: 975-979.
粉煤灰作惰性粒子的镍复合镀层
Viet Hue NGUYEN, Thi Anh Tuyet NGO, Hong Hanh PHAM, Ngoc Phong NGUYEN
Institute of Materials Science, 18 Hoang Quoc Viet, 10 000, Hanoi, Vietnam
摘 要:用粉煤灰(FA)作为惰性粒子,电沉积得到复合镀层Ni/FA。粉煤灰的尺寸为3-7 μm,主要成分是72% SiO2和25% Al2O3。进行电沉积的工艺条件是瓦兹镀镍液含粉煤灰的浓度为5、20、50 g/L,电流密度为2、4 A/dm2, 温度为50 °C,磁搅拌速度为250 r/min。采用扫描电子显微镜(SEM+EDX)、电化学测试和硬度测量来研究复合镀层Ni/FA的形态、成分和性能。镀层中粉煤灰的含量与镀液中粉煤灰的浓度以及相关工艺参数有关。由于镀层中加入了粉煤灰从而使镀层的力学与电化学性能得到改善。复合镀层Ni/FA的硬度达HV430,而纯Ni镀层的硬度只有HV198。电化学测试表明,复合镀层Ni/FA的耐腐蚀比纯镍镀层的高。
关键词:复合镀层;粉煤灰;显微观硬度;耐腐蚀性;惰性粒子
(Edited by Hua YANG)
Corresponding author: Viet Hue NGUYEN; Tel: +84-04-3756-2738; E-mail: hue.nguyenviet@yahoo.com.vn
DOI: 10.1016/S1003-6326(13)62740-5