
J. Cent. South Univ. (2020) 27: 2538-2547
DOI: https://doi.org/10.1007/s11771-020-4479-7

Ablation resistance and mechanism of SiC/ZrC-ZrB2 double layer coating for C/C composites under plasma flame
LIU Han-zhou(刘汉周)1, YANG Xin(杨鑫)1, 2, FANG Cun-qian(方存谦)1,SHI An-hong(石安红)1, CHEN Lei(陈蕾)1, HUANG Qi-zhong(黄启忠)1
1. State Key Laboratory of Powder Metallurgy, Central South University, Changsha 410083, China;
2. Science and Technology of Advanced Functional Composite Laboratory, Aerospace Research Institute of
Materials & Processing Technology, Beijing 100076, China
Central South University Press and Springer-Verlag GmbH Germany, part of Springer Nature 2020
Abstract: To improve the ablation resistance of carbon/carbon (C/C) composites, a SiC/ZrC-ZrB2 double layer coating was fabricated by pack cementation and slurry-sintering method. The ablation resistance of the SiC/ZrC-ZrB2 coating was tested under plasma flame above 2300 °C. The results indicate that the SiC/ZrC-ZrB2 double layer coating exhibits superior ablation resistance than the ZrC-ZrB2 single layer coating. After being ablated under the plasma flame for 20 s, the mass and linear ablation rates of the ZrC-ZrB2 coating are 0.89 mg/s and 15.3 μm/s, while those for SiC/ZrC-ZrB2 coating are 0.09 mg/s and 24.15 μm/s, respectively. During ablation, the SiC inner layer can generate SiO2 glass and result in the formation of ZrO2-SiO2 molten film. Compared with the ZrO2 molten film formed on the ZrC-ZrB2 coating surface, the ZrO2-SiO2 molten film with lower oxygen diffusion rate and viscosity enables the SiC/ZrC-ZrB2 coating to have better self-healing ability. Therefore, the enhanced ablation resistance of the SiC/ZrC-ZrB2 coating can be attributed to the formation of dense ZrO2-SiO2 molten film under the plasma flame.
Key words: ablation; pack cementation; slurry; SiC/ZrC-ZrB2 coating
Cite this article as: LIU Han-zhou, YANG Xin, FANG Cun-qian, SHI An-hong, CHEN Lei, HUANG Qi-zhong. Ablation resistance and mechanism of SiC/ZrC-ZrB2 double layer coating for C/C composites under plasma flame [J]. Journal of Central South University, 2020, 27(9): 2538-2547. DOI: https://doi.org/10.1007/s11771-020-4479-7.
1 Introduction
Owing to excellent thermal shock resistance, high thermal conductivity and low density, carbon/carbon (C/C) composites are considered the most promising candidate thermal structural components applied in aerospace and astronautics area such as engine nozzles and heat protection systems [1-8]. However, the rapid oxidation of C/C composites in an oxidizing atmosphere above 500 °C results in degradation of their high temperature performance [9-11]. Therefore, to extend their application in severe environment, it is essential to improve the ablative property of C/C composites.
At present, preparing an ablative coating that isolates the C/C composites and the external atmosphere is an effective method to improve the ablation resistance of the C/C composites [1, 5, 12, 13]. Among the developed anti-ablation coatings, Zr-base ultra-high temperature ceramics (such as ZrB2 and ZrC) are the attractive coating material due to their high melting point, good strength, excellent ablation resistance and thermal stability [14-18]. Besides, the oxidation product ZrO2 can maintain the integrity of the oxidation surface and retard the further pervasion of the oxygen because of a low vapor pressure on the surface [19, 20]. B2O3 provided by ZrB2 also has high wettability and considerable surface tension to heal up the cracks in the coating, which improves the oxidation resistance at medium temperature (800-1200 °C) [12, 21]. However, the application of Zr-based coating is seriously confined because of the low self-healing ability of ZrO2 and the loss of B2O3 above 1200 °C [15, 16].
According to the previous reports [13, 20, 22, 23], the oxidation resistance of ZrB2 could be enhanced by the addition of SiC with volume fraction of 10%-30% for the formation of silicate- based glasses at high temperatures (1200-1600 °C). In addition, SiC coating is considered as one of the best bonding layers between C/C composites and many kinds of outer layers because of its outstanding physical and chemical compatibility [24-26].
In this paper, SiC/ZrC-ZrB2 double layer coating was prepared through two-step methods. The inner SiC coating was developed by pack cementation and the outer ZrC-ZrB2 coating was brushed by slurry method. Besides, to make a comparison of the ablation performance, C/C composites with ZrC-ZrB2 coating and SiC/ZrC- ZrB2 coating were manufactured, respectively. The composition and microstructure of the coatings were investigated. The ablation ability tested under plasma flame above 2300℃ was also studied.
2 Experimental
2.1 Preparation
Commercially available powders of Si (average particle size of 1 μm, purity of >99%), Zr (average particle size of 3 μm, purity of >99%), B4C (average particle size of 1 μm, purity of >99%) were used as coating materials. 2.5 dimensional C/C composites with a density of 1.70 g/cm3 were used as the coating substrate. Specimens with dimensions of f30 mm×10 mm were cut from bulk 2.5D C/C composites. The specimens were hand- polished using 600 grit SiC paper, and then cleaned ultrasonically with ethanol and dried at 120 °C for 1 h. The inner SiC coating was prepared by pack cementation process. C/C specimens with Si powders were put in a graphite crucible and then were heated in the high temperature furnace (model ZGSJ-100-28) at 1800 °C for 2 h in argon protective atmosphere.
The ZrC-ZrB2 coating was prepared by slurry- sintering method. Before brushing process, Zr and B4C powders were mixed (in mass ratio 7:1) for 10 h in planetary ball mill using absolute ethylalcohol. Then the above powders were dried and mixed with phenolic resin solution to form the slurry. The prepared slurry was brushed onto the surface of the C/C composites and the SiC coated C/C composites specimens. After drying in the vacuum drying oven at 120 °C, the brushed specimens were heat treated at 1500 °C under argon atmosphere for 1 h to prepare the ZrC-ZrB2 coating.
After the above coating process, the ZrC-ZrB2 single layer coating and SiC/ZrC-ZrB2 double layer coating were prepared on C/C composites.
2.2 Ablation
The ablation test is investigated by using Multiplaz 3500 plasma ablation equipment. The surface of the samples is perpendicular to the plasma gun with 10 mm distance. The inner diameter of the nozzle tip was 2 mm. The flame temperature was measured by using an optical pyrometer and reached 2300 °C.
The specific mass ablation rate (mg/s) is calculated by measuring the mass before and after ablation with the following equation:
(1)
where Rm is the mass ablation rate (mg/s); m1 and m2 are the mass (mg) of specimen before and after ablation, respectively; and t is the ablation time (s).
The specific linear ablation rate (mm/s) is calculated by measuring the thickness before and after ablation with the following equation:
(2)
where Rl is the linear ablation rate (mm/s); l1 and l2 are the thickness (mm) of the specimen before and after ablation, respectively; and t is the ablation time (s).
2.3 Characterization
The crystalline structure and morphology of the prepared coating before and after ablation were examined by X-ray diffraction (Rigaku Dmax/ 2550VB+18KW) and scanning electron microscope (NOVA NanoSEM230, Czech Republic) equipped with an energy dispersive spectroscope (EDS, EDAX Inc).
3 Results and discussion
3.1 Microstructure of SiC/ZrC-ZrB2 double layer coating
Figure 1 shows the microstructure and the XRD pattern of the inner layer coating obtained by pack cementation. As Figure 1(a) shows, the surface of inner layer is composed of numerous crystalline and the size of crystalline grain is in the range of 5-30 μm. From Figure 2(b), the diffraction peaks of SiC indicate that the phase composition of the inner coating is SiC.
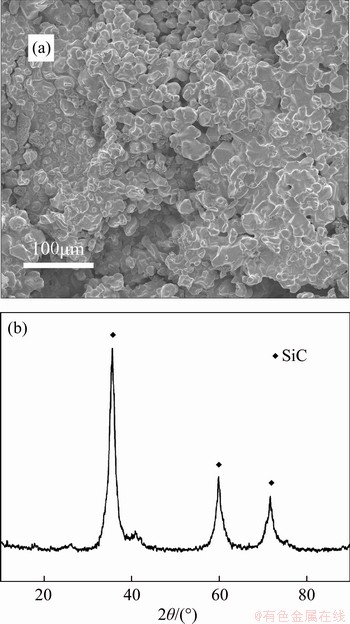
Figure 1 SEM micrograph (a) and XRD pattern (b) of inner layer coating prepared by pack cementation
Figure 2 shows the XRD patterns of the ZrC-ZrB2 out layer prepared on C/C composites and SiC coated C/C composites. From Figure 2(a), it is seen that the dominant phase of the single layer coating is ZrC and ZrB2, and the existence of carbon peak is attributed to the carbonization of phenolic resin. Figure 2(b) shows that the phase composition of the out layer on SiC coating is ZrC and ZrB2, accompanied with little carbon phase. The XRD analysis demonstrates that ZrC-ZrB2 coating can be prepared by slurry-sintering method.
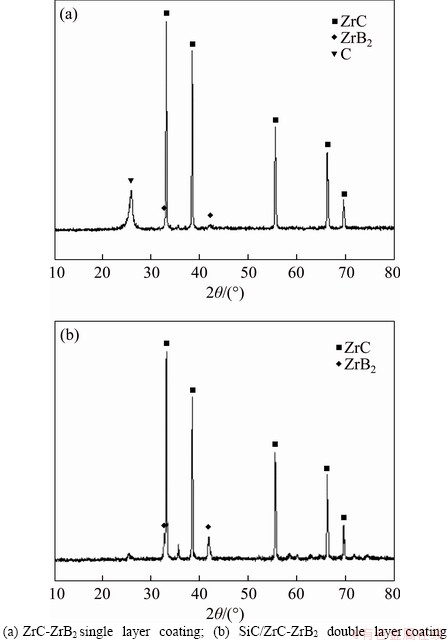
Figure 2 XRD patterns of coated samples:
Figure 3 exhibits the surface images of the ZrC-ZrB2 single layer coating and SiC/ZrC-ZrB2 double layer coating. As shown in Figures 3(a) and (b), the obtained ZrC-ZrB2 coating reveals a nearly dense structure, with some micro-pores present in the coating surface. The formed micro-pores are mainly ascribed to the escaping of gas-by products during carbonization of the phenolic resin. From Figures 3(a) and (b), it is seen that the ZrC-ZrB2 coating is mainly composed of the bright and dark phases. According to EDS analysis results (Figures 3(c)-(e)), the bright phase contains relatively higher Zr elements, while the dark phase shows higher C elements, indicating that the former is ZrC-ZrB2 rich phase and the latter is carbon rich phase. Because EDS cannot detect the light weight element of B, B element is missing in Figures 3(c)-(e). Meanwhile, it is noted that the distributed bright phase forms a network skeleton in the ZrC-ZrB2 coating, which is beneficial for the improvement of ablation resistance during ablation.
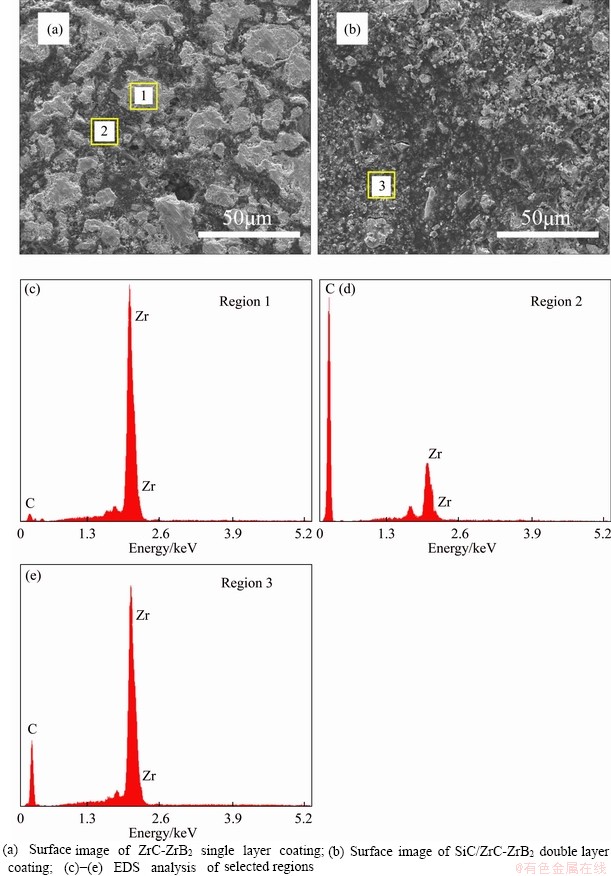
Figure 3 SEM images and EDS analysis of surface of coated samples:
Figure 4 exhibits the cross-sectional morphologies of the ZrC-ZrB2 single layer coating and SiC/ZrC-ZrB2 double layer coating. As for the ZrC-ZrB2 single layer coating (Figure 4(a)), a transition layer is formed between the C/C matrix and ceramic coating (the yellow line), indicating that the prepared coating has good combination with C/C substrate due to the reaction of partial carbon matrix with Zr element (from ZrC-ZrB2 coating) in the sintering process. The thickness of the ZrC-ZrB2 single layer coating is about 30 μm, which is identical with the prepared outer layer ZrC-ZrB2 in the SiC/ZrC-ZrB2 double layer coating, displayed in Figure 4(b). Figure 4(b) shows the typical cross-sectional image of the prepared SiC/ZrC-ZrB2 double layer coating. From Figure 4(b), it is observed that the coating reveals an obvious double layer structure, and no obvious interface is found between the coating layers and the substrate, indicating a good bonding ability of the prepared coating. The thickness of the SiC inner layer and ZrC-ZrB2 outer layer is about 20 and 35 μm, respectively. Compared with the ZrC-ZrB2 single layer coating, the SiC transition layer in SiC/ZrC-ZrB2 double layer coating plays a positive role in the improvement of the coating performance. On one hand, the SiC inner coating prepared by reaction method is chemical bonding, which combines well with the substrate; meanwhile, it can effectively alleviate the coefficient of thermal expansion (CTE) mismatch of ZrC-ZrB2 with carbon substrate and enhance the thermal shock resistance of the ZrC-ZrB2 coating. On the other hand, the SiC transition layer can improve the anti-oxidation property of the SiC/ZrC- ZrB2 double layer coating. In the ablation process, the oxidized SiC inner coating can generate silica glass to improve the self-healing ability of the ZrO2 film, which enhances the ablation resistance of the ZrC-ZrB2 coating.
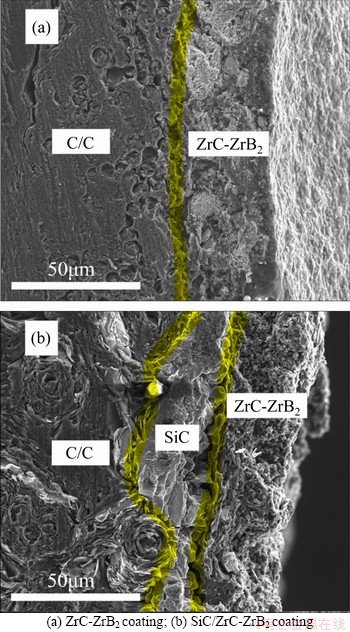
Figure 4 Cross-sectional SEM images of coated samples:
3.2 Ablation property of SiC/ZrC-ZrB2 double layer coating
The mass and linear ablation rates of the coated samples after ablation for 20 s are listed in Table 1. According to ablation results, the mass and linear ablation rates of ZrC-ZrB2 single layer coating are 0.89 mg/s and 15.30 μm/s, respectively, while those for SiC/ZrC-ZrB2 double layer coating are 0.09 mg/s and 24.15 μm/s, respectively. It is obvious that the mass ablation rate of the SiC/ZrC-ZrB2 double layer coating is one order of magnitude less than that of ZrC-ZrB2 single layer coating, indicating that the preparation of SiC inner coating can effectively enhance the ablation resistance of the single ZrC-ZrB2 coating. After being ablated for 20 s, the linear ablation rate of SiC/ZrC-ZrB2 double layer coating is close to that of ZrC-ZrB2 single layer coating. The slight increase of linear ablation rate for SiC/ZrC-ZrB2 double layer coating is mainly attributed to the ablation of SiC phase. The SiC exhibits better anti-oxidation resistance than ZrC-ZrB2 coating, however, the ZrC-ZrB2 shows superior ablation resistance than SiC ceramic due to their relative high melting point. Therefore, the introduction of SiC inner coating increases the linear ablation resistance of the SiC/ZrC-ZrB2 double layer coating, with the mass ablation rate significantly declined.
Table 1 Mass and linear ablation rates of coated samples after ablation for 20 s
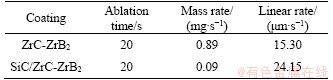
3.3 Morphology of ablated coating
Figure 5 displays the XRD pattern of the ablated SiC/ZrC-ZrB2 double layer coating. As shown in Figure 5, diffraction peaks of ZrO2, SiO2 were characterized, which indicates that the molten oxides are generated on the ablated coating surface. Due to the low melting point of B2O3 (450 °C), B2O3 was volatilized completely under the scouring plasma flame. Similar results can also be found in other work [23].
Figure 6(a) shows the SEM images and EDS analysis of the ablation central for the ablated ZrC-ZrB2 single layer coating. As shown in Figure 6(a), droplet-like molten phase with smooth morphology forms a continuous film on the coating surface. The magnified image of Figure 6(b) shows that micro-pores are present in the molten film due to the high viscosity of the ZrO2 scales under the plasma flame. EDS analysis (Figure 6(c)) demonstrates that the molten phase is ZrO2. The formation of the molten ZrO2 layer can effectively improve the ablation resistance of the coating. However, the high viscosity of the molten ZrO2 results in a weak self-healing ability of the protective layer; therefore, the molten ZrO2 layer with rough morphology and micro-pores was formed in the ablation center. The formed micro-pores can provide pass channel for inward diffusion of the oxygen, which is harmful to the ablation resistance of ZrC-ZrB2 coating.
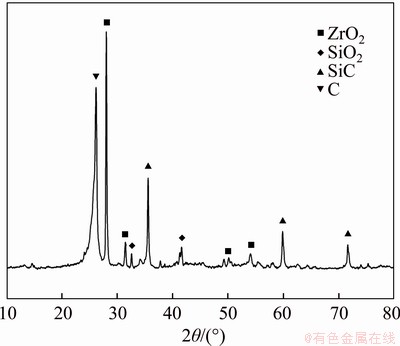
Figure 5 XRD pattern of ablated SiC/ZrC-ZrB2 double layer coating
Figure 7 shows the SEM images and EDS analysis of the ablation central for the ablated SiC/ZrC-ZrB2 double layer coating. From Figure 7(a), it can be seen that the ablated surface was covered with a smooth molten film with numerous scattered particles. Compared with the ablated ZrC-ZrB2 single layer coating, the formed ZrO2-SiO2 molten film is much more compact (Figure 7(a)), with fewer micro-pores observed on the ZrO2-SiO2 molten film (Figure 7(b)). EDS analysis results demonstrate that the particle-like molten product is ZrO2 rich phase (Figure 7(c)), and the smooth glass-like product is SiO2 rich phase (Figure 7(d)). During ablation, the inner SiC coating was partially oxidized and generated SiO2 glass. Unlike the particle-like ZrO2 molten phase, the silica glass has much lower viscosity and good flowability, which enables the protective film to have better self-healing ability. Compared with the single ZrO2 molten film, the formed ZrO2-SiO2 molten film exhibits a superior anti-oxidation and ablation resistance. Firstly, the ZrO2-SiO2 molten film with better self-healing ability can effectively bond the ZrO2 molten particles in the ablation center, reducing the scoured ZrO2 molten particles in the ablation process. Secondly, due to the good self-healing ability of the ZrO2-SiO2 molten film,the sealing of micro-pores cuts the diffusion channel of oxygen to attacking the inner region. Moreover, the oxygen permeability rate of silica glass is much lower than that of ZrO2 film [26, 27]; therefore, the reduced oxygen permeability rate of ZrO2-SiO2 molten film is beneficial for the improvement of ablation resistance for the double layer coating.
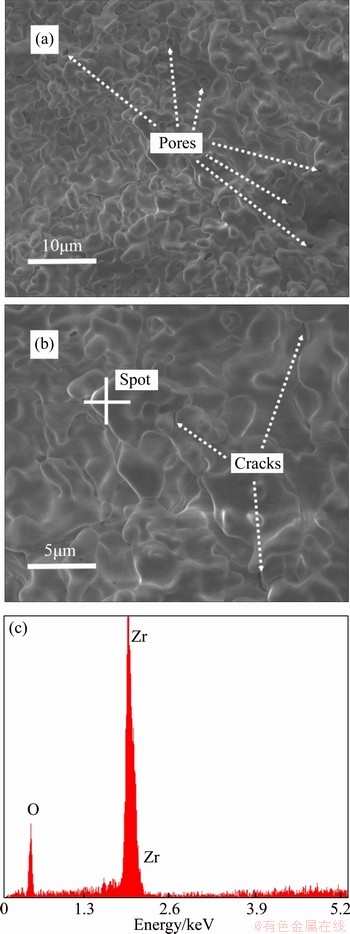
Figure 6 SEM images (a, b) and EDS analysis (c) of ablation central for ZrC-ZrB2 single layer coating after ablation
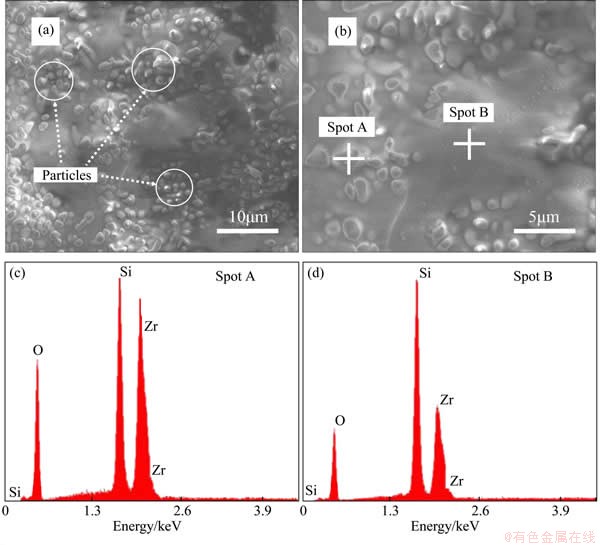
Figure 7 SEM images (a, b) and EDS analysis (c, d) of ablation central for SiC/ZrC-ZrB2 double layer coating after ablation
3.4 Ablation mechanism of SiC/ZrC-ZrB2 double layer coating
Based on the above analysis, several processes were proposed to illustrate the ablation mechanism of the SiC/ZrC-ZrB2 double layer coatings (Figure 8). During the ablation, the main reactions of the SiC/ZrC-ZrB2 coating are as follows:
C(s)+O2(g)→CO(g) (1)
ZrB2(s)+O2→ZrO2(s)+B2O3(l) (2)
ZrC(s)+O2(g)→ZrO2(s)+CO(g) (3)
SiC(s)+O2(g)→SiO(g)+CO(g) (4)
SiC(s)+O2(g)→SiO2(s)+CO(g) (5)
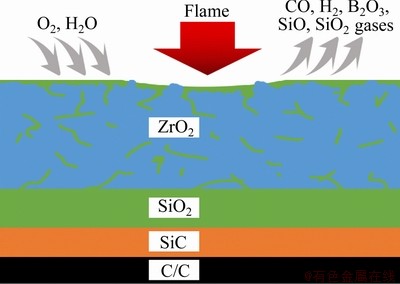
Figure 8 Ablation mechanism of SiC/ZrC-ZrB2 double layer coating
SiC(s)+H2O(g)→SiO2(s)+CO(g)+H2(g) (6)
ZrB2(s)+H2O(g)→ZrO2(s)+B2O3(l)+CO(g)+H2(g) (7)
ZrC(s)+H2O(g)→ZrO2(s)+CO(g)+H2(g) (8)
B2O3(l)→B2O3(g) (9)
ZrO2(s)→ZrO2(l) (10)
SiO2(s)→SiO2(l) (11)
SiO2(l)→SiO2(g) (12)
The ZrC-ZrB2 coating reacts with oxygen in the beginning of ablation, and ZrO2 and B2O3 are generated rapidly on the coating surface based on Eqs. (2), (3), (7) and (8). Meanwhile, the oxidation of residual carbon results in escaping of CO gas (Eq. (1)), which provides a space for the volume expansion of Eqs. (2), (3), (7) and (8). Therefore, the residual carbon maintains the structural completeness of the ablated surface due to the reduced cracks generated by the volume expansion.
With increasing temperature of the ablated surface and the inner diffusing of oxygen, the SiC coating was gradually oxidized (Eqs. (4)-(6)). In this condition, the oxidized outer layer is a skeleton of ZrO2 filled with B2O3 glass. Owing to the good wettability and low viscosity of B2O3 glass, the defects in the ZrO2 skeleton are sealed. However, as the ablation temperature exceeds 1200 °C, B2O3 glass is volatilized and evaporated (Eq. (6)). In the following ablation, the SiO2 glass (Eqs. (5) and (6)) replaces the position of B2O3 glass and seals the pores of the ZrO2 skeleton, enabling the SiC/ZrC- ZrB2 double layer coating to have better anti-oxidation and ablation resistance. Due to the high temperature of the plasma flame, the molten SiO2 glass was partially evaporated and removed from the surface in the ablation center. However, unlike the single SiO2 glass phase that can be easily scoured away from the surface, the formed ZrO2-SiO2 molten film plays a positive role in reducing the ablation of SiO2 glass in the ablation center. Firstly, the ZrO2 skeleton combines with the SiO2 glass to form the ZrO2-SiO2 molten film with better self-healing ability. Secondly, owing to the high viscosity of ZrO2, the SiO2 glass can be effectively immobilized in the skeleton of ZrO2 (Figures 7(a) and (b)), therefore, the scouring of SiO2 glass was retarded. In the ablation process, the formed ZrO2-SiO2 molten film with good self-healing ability and low oxygen diffusion rate can act as a thermal barrier to protect the substrate from further ablation. Therefore, the SiC/ZrC-ZrB2 double layer coating exhibits a significantly improved mass ablation resistance than the ZrC-ZrB2 single layer coating.
4 Conclusions
SiC/ZrC-ZrB2 double layer coating and ZrC-ZrB2 single layer coating are prepared on C/C composites to enhance the high temperature ablative property. The prepared inner SiC coating has a great effect on improvement of the ablation resistance for ZrC-ZrB2 coating. The inner SiC coating can generate SiO2 glass and result in the formation of ZrO2-SiO2 molten film; therefore, the mass ablation rate of the SiC/ZrC-ZrB2 coating is one order of magnitude lower than that of ZrC-ZrB2 single layer coating. After ablated under the plasma flame for 20 s, the mass and linear ablation rates of the ZrC-ZrB2 single layer coating are 0.89 mg/s and 15.3 μm/s, respectively, while those for SiC/ZrC-ZrB2 double layer coating are 0.09 mg/s and 24.15 μm/s. Compared with the ZrO2 molten film that formed on the ZrC-ZrB2 coating surface, the ZrO2-SiO2 molten film with lower oxygen diffusion rate and viscosity enables the SiC/ZrC-ZrB2 double layer coating to have better self-healing ability. Therefore, the SiC/ZrC-ZrB2 double layer coating exhibits superior ablative property than ZrC-ZrB2 coating due to the formed dense ZrO2-SiO2 molten film under the plasma flame.
Contributors
The overarching research goals were developed by YANG Xin and HUANG Qi-zhong. LIU Han-zhou prepared the coatings and conducted the micro-structure characterization. LIU Han-zhou, FANG Cun-qian, SHI An-hong and CHEN Lei performed the ablation tests. LIU Han-zhou, YANG Xin, FANG Cun-qian, SHI An-hong and CHEN Lei analyzed the ablation results. The initial draft of the manuscript was written by LIU Han-zhou, YANG Xin and HUANG Qi-zhong. All the authors replied to reviewers’ comments and revised the final version.
Conflict of interest
LIU Han-zhou, YANG Xin, FANG Cun-qian, SHI An-hong, CHEN Lei and HUANG Qi-zhong declare that they have no conflict of interest.
References
[1] ALIASGARIAN R, NADERI M, MIRSALEHI S E, SAFI S. The ablation behavior of ZrB2-SiC coating prepared by shrouded plasma spray on SiC-coated graphite [J]. Journal of Alloys and Compounds, 2018, 74: 797-803.
[2] WANG P, ZHOU S B, HU P, CHEN G Q, ZHANG X H, HAN W B. Ablation resistance of ZrB2-SiC/SiC coating prepared by pack cementation for graphite [J]. Journal of Alloys and Compounds, 2016, 682: 203-207.
[3] WANG P, HAN W B, ZHANG X H, LI N, ZHAO G D, ZHOU S B. (ZrB2-SiC)/SiC oxidation protective coatings for graphite materials [J]. Ceramics International, 2015, 41(5): 6941-6949.
[4] WANG P, HAN W B, JIN X X, ZHANG X H, GAO J X, ZHOU S B. Oxidation resistant zirconium diboride-silicon carbide coatings for silicon carbide coated graphite materials [J]. Journal of Alloys and Compounds, 2015, 629: 124-130.
[5] XIANG Y, PENG Z H, WANG Y, XING Z F, CAO F. ZrB2-SiC ceramics coating for C-f/SiC composites: Microstructure and anti-ablation mechanism [J]. Journal of Alloys and Compounds, 2018, 750: 857-862.
[6] YUAN Yi-dong, ZHANG Fu-kuan, ZHOU Wan-cheng. Preparation and characteristics of C/C composite brake disc by multi-cylindrical chemical vapor deposition processes [J]. Journal of Central South University of Technology, 2005, 12(4): 400-402.
[7] VIGNOLES G L, ASPA Y, QUINTARD M. Modelling of carbon-carbon composite ablation in rocket nozzles [J]. Composites Science and Technology, 2010, 70(9): 1303- 1311.
[8] ZAMAN W, LI K Z, IKRAM S, LI W, ZHANG D S, GUO L J. Morphology, thermal response and anti-ablation performance of 3D-four directional pitch-based carbon/ carbon composites [J]. Corrosion Science, 2012, 61: 134-142.
[9] ZHOU S B, QI Y S, WANG P, CHENG Y H, HAN W B. A ZrB2-SiC/SiC oxidation protective dual-layer coating for carbon/carbon composites [J]. Advances in Applied Ceramics, 2017, 116(8): 462-467.
[10] PAUL B, PRAKASH J, SARKAR P S. Formation and characterization of uniform SiC coating on 3-D graphite substrate using halide activated pack cementation method [J]. Surface & Coatings Technology, 2015, 282: 61-67.
[11] SUN C, LI H J, FU Q G, ZHANG J P, PENG H. Double SiC coating on carbon/carbon composites against oxidation by a two-step method [J]. Transactions of Nonferrous Metals Society of China, 2013, 23(7): 2107-2112.
[12] YAO X Y, LI H J, ZHANG Y L, REN J J, YAO D J, TAO J. A SiC/ZrB2-SiC/SiC oxidation resistance multilayer coating for carbon/carbon composites [J]. Corrosion Science, 2012, 57: 148-153.
[13] REN X R, LI H J, CHU Y H, FU Q G, LI K Z. Ultra-high-temperature ceramic HfB2-SiC coating for oxidation protection of SiC-coated carbon/carbon composites [J]. International Journal of Applied Ceramic Technology, 2015, 12(3): 560-567.
[14] JIA Y J, LI H J, FENG L, SUN J J,LI K Z,FU Q G. Ablation behavior of rare earth La-modified ZrC coating for SiC-coated carbon/carbon composites under an oxyacetylene torch [J]. Corrosion Science, 2016, 104: 61-70.
[15] FENG T,LI H J, SHIX H, YANGX,WANG S L. Oxidation and ablation resistance of ZrB2-SiC-Si/B-modified SiC coating for carbon/carbon composites [J]. Corrosion Science, 2013, 67: 292-297.
[16] HUANG D, ZHANG M Y, HUANG Q Z, WANG L P,TONG K. Mechanical property, oxidation and ablation resistance of C/C-ZrB2-ZrC-SiC composite fabricated by polymer infiltration and pyrolysis with preform of C-f/ZtB(2) [J]. Journal of Materials Science & Technology, 2017, 33(5): 481-486.
[17] HU C, NIU Y R, HUANG S S, LI H,REN M S,ZENG Y, ZHENG X B,SUN J L. In-situ fabrication of ZrB2-SiC/SiC gradient coating on C/C composites [J]. Journal of Alloys and Compounds, 2015, 646: 916-923.
[18] XIN Y, Qizhong H , ZHEAN S, YANG X,HUANG Q Z,SU Z A,CHANG X,XUE L,ZHONG P,LI J. Ablative property and mechanism of C/C-ZrB2-ZrC-SiC composites reinforced by SiC networks under plasma flame [J]. Corrosion Science, 2016, 107: 9-20.
[19] JIA Y J, LI H J, FU Q G, SUN J J. A ZrC-SiC/ZrC-LaB6/ZrC multilayer ablation resistance coating for SiC-coated carbon/carbon composites [J]. Surface & Coatings Technology, 2017, 309: 545-553.
[20] LI L, LI H J, YIN X M, LIN H J,SHEN Q L,YAO X Y,FENG T,FU Q G. Microstructure evolution of SiC-ZrB2-ZrC coating on C/C composites at 1773 K under different oxygen partial pressures [J]. Journal of Alloys and Compounds, 2016, 687: 470-479.
[21] LIU Y S, CHENG L F, ZHANG L T, WU S J,LI D,XU Y D. Oxidation protection of multilayer CVD SiC/B/SiC coatings for 3D C/SiC composite [J]. Materials Science and Engineering A-Structural Materials Properties Microstructure And Processing, 2007, 466(1, 2): 172-177.
[22] LI L, LI H J, YIN X M, CHU Y H,CHEN X,FU Q G. Oxidation protection and behavior of in-situ zirconium diboride-silicon carbide coating for carbon/carbon composites [J]. Journal of Alloys and Compounds, 2015, 645: 164-170.
[23] HUANG D, ZHANG M Y, HUANG Q Z, WANG L P,TANG X,YANG X,TONG K. Fabrication and ablation property of carbon/carbon composites with novel SiC-ZrB2 coating [J]. Transactions of Nonferrous Metals Society of China, 2015, 25(11): 3708-3715.
[24] FENG T, LI H J, FU Q G, SHEN X T,WU H. Microstructure and oxidation of multi-layer MoSi2-CrSi2-Si coatings for SiC coated carbon/carbon composites [J]. Corrosion Science, 2010, 52(9): 3011-3017.
[25] CHU Y H, FU Q G, LI H J, LI K Z,ZOU X,GU C G. Influence of SiC nanowires on the properties of SiC coating for C/C composites between room temperature and 1500 °C [J]. Corrosion Science, 2011, 53(9): 3048-3053.
[26] REN X R, LI H J, CHU Y H, LI K Z,FU Q G. ZrB2-SiC gradient oxidation protective coating for carbon/carbon composites [J]. Ceramics International, 2014, 40(5): 7171-7176.
[27] SQUIRE T H, MARSCHALL J. Material property requirements for analysis and design of UHTC components in hypersonic applications [J]. Journal of the European Ceramic Society, 2010, 30(11): 2239-2251.
(Edited by FANG Jing-hua)
中文导读
等离子火焰下C/C复合材料的SiC/ZrC-ZrB2双层涂层的耐烧蚀性能及其机理
摘要:为了提高碳/碳(C/C)复合材料的耐烧蚀性能,采用包埋法和料浆刷涂法制备了SiC/ZrC-ZrB2双层涂层。在2300 °C以上的等离子火焰下测试了SiC/ZrC-ZrB2涂层的耐烧蚀性能。结果表明,SiC/ZrC-ZrB2双层涂层的耐烧蚀性能优于ZrC-ZrB2单层涂层。等离子火焰烧蚀20 s后,ZrC-ZrB2涂层的质量烧蚀率和线烧蚀率分别为0.89 mg/s和15.3 μm/s,而SiC/ZrC-ZrB2涂层的质量烧蚀率和线烧蚀率分别为0.09 mg/s和24.15 μm/s,烧蚀过程中,SiC内层产生SiO2玻璃相,形成ZrO2-SiO2熔融膜。与ZrC-ZrB2涂层表面形成的ZrO2熔融膜相比,具有较低氧扩散速率和黏度的ZrO2-SiO2熔融膜使SiC/ZrC-ZrB2涂层具有较好的自愈性。因此,SiC/ZrC-ZrB2涂层耐烧蚀性能的提高可归因于等离子烧蚀过程中形成的致密的ZrO2-SiO2熔融膜。
关键词:烧蚀;包埋法;料浆刷涂法;SiC/ZrC-ZrB2涂层
Foundation item: Project(51304249) supported by the National Natural Science Foundation of China; Project(14JJ3023) supported by the Science Foundation of Hunan Province, China
Received date: 2019-03-04; Accepted date: 2020-01-15
Corresponding author: YANG Xin, PhD, Associate Professor;Tel: +86-731-88836078; E-mail: yangxincsu@csu.edu.cn; ORCID: https://orcid.org/0000-0001-8889-9230