
J. Cent. South Univ. (2021) 28: 48-57
DOI: https://doi.org/10.1007/s11771-021-4585-1

Production of titanium powder by metallothermic reduction of TiO2 in cold pressed pellets
ZHENG Hai-yan(郑海燕)1, 2, GUO Yong-chun(郭永春)1, 2, SHEN Feng-man(沈峰满)1, 2
1. Key Laboratory for Ecological Metallurgy of Multimetallic Mineral (Ministry of Education),Northeastern University, Shenyang 110819, China;
2. School of Metallurgy, Northeastern University, Shenyang 110819, China
Central South University Press and Springer-Verlag GmbH Germany, part of Springer Nature 2021
Abstract: In order to produce low-cost titanium (Ti) with high productivity, fundamental studies on producing metallic Ti from titanium dioxide (TiO2) in the cold pressed pellets were conducted by metallothermic reduction with an indirect contact method. This paper focuses on discussing the mechanism of the reduction process and the relationships of RM (a revised reduction index) with reduction temperature, reduction time, and mole ratio of TiO2 to CaCl2 (nTiO2/nCaCl2) in the pellets. The results show that metallic Ti was obtained from the reduction of TiO2 in the pellets by calcium (Ca) vapor; pellets were reduced homogenously and Ca vapor diffused into the porous pellets by Knudsen diffusion or the mixing diffusion of molecular diffusion and Knudsen diffusion at 1273 K; RM increased with the increases of temperature and reduction time and was 96.34% when TRedu=1273 K, tRedu=6 h, and nTiO2/nCaCl2=4; the reasonable nTiO2/nCaCl2 value is 3-5 for the pellets with enough strength and high RM.
Key words: titanium dioxide; titanium powder; metellothermic reduction; cold pressed pellet; reduction index
Cite this article as: ZHENG Hai-yan, GUO Yong-chun, SHEN Feng-man. Production of titanium powder by metallothermic reduction of TiO2 in cold pressed pellets [J]. Journal of Central South University, 2021, 28(1): 48-57. DOI: https://doi.org/10.1007/s11771-021-4585-1.
1 Introduction
Titanium (Ti) with excellent physical and chemical properties is used in various fields from the aerospace industry, marine industry, and chemical plants to buildings, implants, and the production of several consumer products. Ti is not actually a rare substance as it ranks as the ninth most plentiful element [1]. Unfortunately, it is seldom found in high concentrations and never found in a pure state. Thus, the difficulty in processing the metal makes it expensive. Even today it is produced only in a batch process—Kroll process [2-4], and no continuous process exists as for other structural metals. Many researchers around the world are attempting to improve the current smelting process or develop an alternative Ti smelting process in which metallic Ti of the requested specification can be produced with a high productivity and low cost [2-13].
Based on preform reduction process (PRP) [11], the preparation of metallic Ti by metallothermic reduction of TiO2 in the cold pressed pellets (CPP) with an indirect contact method was investigated in this study. This CPP reduction process consists of four major steps: 1) cold pressing for pelletization from a mixture of TiO2 or Ti ore, flux, and binder at room temperature under a pressure; 2) calcination of the pellets at elevated temperatures; 3) reduction of TiO2 by calcium with an indirect contact method, i.e. no direct contact of TiO2 with reducing agent (calcium shots), at elevated temperatures; and 4) acid pickling in an ultrasonic wave field. It is worthy to mention that the amount of flux (or molten salt) used in the reduction process can be decreased substantially in this process as compared to the electrochemical processes in which molten salt is used. In addition, contamination from the reactor and reducing agent can be prevented and purity can be controlled because the surface of the pellets contacting with the reactor is small and there is no direct contact of pellets with reducing agent. As compared to the conventional metallothermic reduction process, the technology of cold pressing pelletization is introduced for reducing the amount of flux and binder further because it is easy to prepare the pellets under the pressure and beneficial to homogenous reduction; removing impurities by acid pickling in an ultrasonic wave field is more effective than that in conventional process. Thus, this process by metallothermic reduction of CPP is suitable for producing high-purity Ti powder directly from TiO2, and it possesses a flexible scalability.
This work focuses on discussing the mechanism of the reduction from TiO2 in the cold pressed pellets, which indirectly contacts with reducing agent of calcium shots, and the relationships of RM (a reduction index) with reduction temperature, reduction time, and nTiO2/nCaCl2 (mole ratio of TiO2 to CaCl2 ) in the pellets.
2 Metallothermic reduction
It is well known that it is difficult to remove the dissolved oxygen in solid Ti due to the high oxygen affinity of Ti [14-16]. From Ellingham diagrams of the oxides, TiO2 can be reduced only by a few reactive metals, such as Ca and Mg, and the reducibility of Ca is higher than that of Mg. Standard Gibbs energy change (△GΘ) in the reduction reaction of TiO2 by Ca is shown as [17]:
TiO2(s)+2Ca(s,l)=Ti(s)+2CaO(s),
–332.12+ 0.034T (1)
where T is in rage of 600-1500 K.
Furthermore, the vapor pressure of Ca as shown in reaction (2) is 1722 Pa at 1273 K, which is high enough and can be supplied as a vapor at elevated temperatures higher than 1273 K. In addition, there is no alloy or solid solution between Ti and Ca based on Ref. [18] and the calculation of FactSage Thermochemical Software. These facts indicate that Ca vapor is suitable for reducing TiO2 in the pellets. The overall reaction of the reduction process can be expressed by reaction (3).
Ca(l)=Ca(g),
167.98+0.098T [17],
43.1 kJ/mol at 1273 K,
1722 Pa at 1273 K[17]. (2)
TiO2(s)+2Ca(g)=Ti(s)+2CaO(s),
–668.09+0.230T [17],
–659.87+0.029T[17],
–375.3 kJ/mol at 1273 K,
–659.4 kJ/mol at 1273 K [17]. (3)
3 Experimental
In a typical experiment, the feed pellets (d20 mm×8 mm) were fabricated at room temperature under 45 MPa pressure from a mixture that was prepared by mixing TiO2, CaCl2 as the flux, and a small amount of binder (dextrin from maize starch). It is noteworthy that CaCl2 was used as the flux because the oxygen in Ti could be extracted as CaO that dissolves into molten CaCl2 at elevated temperatures [19, 20]. On the other way, the amount of CaCl2 should be controlled that the feed pellets are prepared with a certain mechanical strength and proper porosity. In this study, the value of nTiO2/nCaCl2 was controlled between 2 and 6 for discussing the effect of nTiO2/nCaCl2 on reduction. Then, the fabricated pellets were heated at 1273 K for 2 h under the air atmosphere in order to remove the binder and water present in the pellets and to ensure the pellets with a certain mechanical strength and proper porosity. In the reduction step, the calcined pellets and calcium shots (99%, approximately one and a half of the stoichiometric amount) were placed in a sealed reactor as shown in Figure 1. The reactor was then heated at elevated temperatures (1173-1373 K) for 3-7 h, respectively. After the reduction, the reactor was removed from the furnace and quenched quickly.
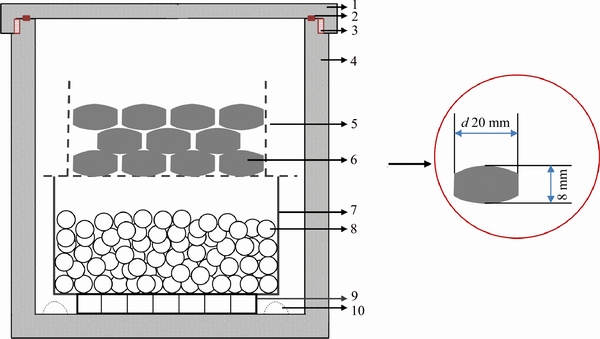
Figure 1 Schematic illustration of experimental apparatus for reduction (1-Stainless cover; 2-Sealing gasket; 3-Screw thread; 4-Stainless steel reactor; 5-Ni-Cr net cup; 6-Calcined pellets; 7-Stainless steel holder; 8-Ca particles)
The pellets recovered at room temperature were crushed roughly and subjected to various analyses and acid pickling for removing the impurities from the sample. In the acid pickling process, the sample obtained from the reduction process reacted with 50% acetic acid solution for 6 h and 20% hydrochloric acid solution for 0.5 h in order to remove the by-product CaO and the excess reductant Ca present in the sample. Further, the sample was rinsed thrice with distilled water, twice with isopropanol, and once with acetone at room temperature. Finally, the obtained powder was dried in a vacuum dryer.
The raw materials and the experimental conditions in this study are shown in Tables 1 and 2, respectively. As shown in Table 2, single factor experiment was employed in order to clear the mechanism of the reduction process and the effects of reduction temperature, reduction time, and mole ratio of TiO2 to CaCl2 (nTiO2/nCaCl2) on the reduction. In other words, experiments for investigating the effect of reduction temperature on the reduction were designed as the conditions of Exp. Nos. 1-5, in which the interval temperature was 50°C; experiments for investigating the effect of reduction time on the reduction were introduced as the design of Exp. Nos. 3, 6-8 and 9, in which the interval time was 1 h; experiments for investigating the effect of mole ratio of TiO2 to CaCl2 (nTiO2/nCaCl2) on the reduction were conducted as Exp. Nos. 10, 11, 3, 12, and 13.
Table 1 Raw materials used in this study
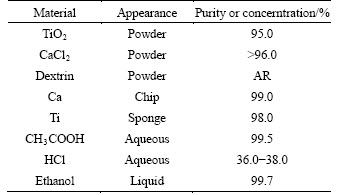
The samples in this study were characterized by analytical instruments, e.g., X-ray fluorescence spectrometer (XRF, Germany, Bruker Q4), X-ray diffractometer (XRD, Germany, Bruker D8), electron probe micro-analyzer (EPMA, Japan, Shimazu EPMA-1610), and scanning electron microscope-energy dispersive spectrometer (SEM- EDS, Japan, Shimazu SSX-550TM).
4 Results and discussion
4.1 Morphology of samples
Figures 2(a)-(c) show the photographs of the samples before calcination, after calcination, and after reduction. The sample before calcination (Figure 2(a)) was a white, hard, and compact pellet, and the sample after calcination turned into brown and no obvious change appeared. But the sample after reduction (Figure 2(c)) turned into gray black, looked fluffy, and easily to be crushed. The most important phenomenon after reduction was that there was no obvious difference between the outside layer and the core of the pellets shown in Figure 2(c) and it indicates that the reduction of the pellets by Ca vapor would be homogeneous. Figures 2(d)-(f) are the SEM images of the samples after reduction. As shown in Figure 2(d), the sample consists of porous particles. Figures 2(e) and (f) show that the diameter of the pore (dpore) in the particles is mainly over the range of 0.5-5 μm and it is big enough for the diffusion of Ca vapor into the pellets (the diameter of Ca atom is 3.98× 10-4 μm). The mean free path
of Ca atom at 1273 K is 20.5 μm which is calculated by Eq. (4) [20] for the perfect gases and
is between 4.1 and 41, so the diffusion of Ca vapor in the pellets should be expressed by Knudsen diffusion or the mixing diffusion of molecular diffusion and Knudsen diffusion. All of the above mentioned facts indicate that Ca vapor is easy to reach the reaction surface inside the pellets at elevated temperatures and the reduction of the pellets by Ca vapor would be homogeneous and proceed smoothly. Thus, the pellets after reduction were easily crushed due to their porous microstructure.
(4)
where kB is Boltzmann constant, 1.38×10-23 J/K; T is temperature, K; d is diameter of Ca atom, 0.398 nm; p is pressure of Ca at 1273 K, 1216 Pa.
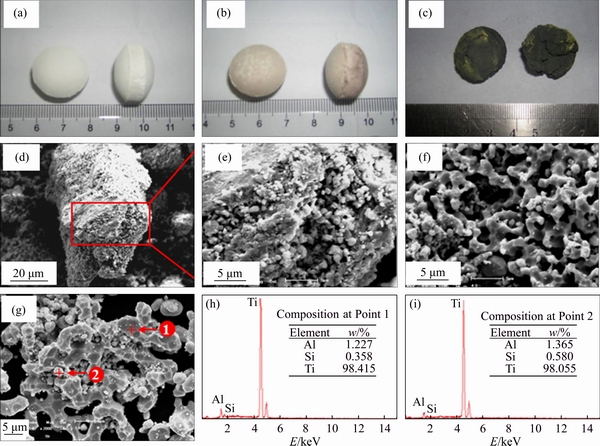
Figure 2 Appearance of samples after cold pressing pelletization (a), after calcination (b), after reduction (c); SEM images of samples after reduction (Exp. No. 8) (d, e), after reduction (Exp. No. 9) (f), after pickling (Exp. No. 5) (g); EDS analyses of Point 1 (Exp.No. 5) (h), Point 2 (Exp.No. 5) (i)
Table 2 Experimental conditions of reduction in this study
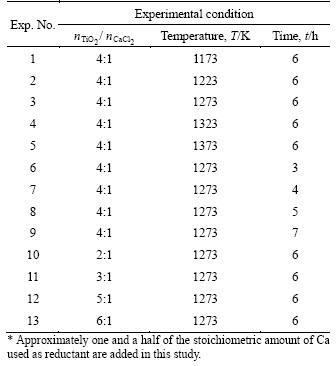
4.2 Phases of sample by XRD
Because by-product CaO can be dissolved into acids easily, the samples after reduction were crushed roughly and subjected to acid pickling for removing the impurities, and finally a grey powder was obtained.
Figure 3 shows the XRD and EPMA analyses of the samples obtained after reduction and acid pickling. Combining the XRD patterns with EPMA analyses in Figure 3, the sample after the reduction was the mixture of product Ti, reductant Ca, and by-product CaO; the sample after acid pickling was only α-Ti, and Ca and CaO were removed during the acid pickling. These results indicate that TiO2 in the form of CPP was reduced by Ca vapor and metallic Ti was obtained, which agrees well with the thermodynamic analysis. Therefore, this process for producing metallic Ti directly from TiO2 by CPP is feasible.
In some experiments (Exp. Nos. 1 and 10), CaTiO3 phase was also identified in the sample after reduction. The formation of CaTiO3 is partly due to the reaction of TiO2 with CaO, a by-product of the reduction process, which is difficult to be removed by acid pickling.
4.3 Reduction index, RM
The main reaction in this study was a gas (Ca vapor)-solid (TiO2 in the pellets) reaction shown in reaction (3), and there was no change for Ti mass and some increment for Ca mass in the pellets due to the generation of by-product CaO from Ca vapor. So, there must be certain relationship between the increment of Ca mass and the reduction degree, and the mass balance of the reduction reaction is shown in Table 2.
R in Table 3 is the molar conversion ratio, i.e. the molar ratio of reacted TiO2 to original TiO2, and defined as reduction index (R), a criterion of the reduction degree. nTiO2/nCaCl2 is the molar ratio of TiO2 to CaCl2 in the pellets and its value is n (n=2, 3, 4, 5 or 6) in this study.
The relationship of the masses of Ti (wTi×m) and Ca (wCa×m), n, and R is shown as:
(5)
Therefore,
(6)
where n is the ratio of nTiO2/nCaCl2, n=2, 3, 4, 5, or 6 in this study; wCa is the concentration of Ca in the sample after reduction (%); wTi is the concentration of Ti in the sample after reduction (%); m is the mass of the sample after reduction (g).
It is noted that wCa is the concentration of Ca in the sample after reduction and in theory is the sum of Ca from CaO by-product generated and CaCl2 added as the flux. However, wCa obtained from the analysis data in Eq. (5) actually included not only Ca from CaO by-product generated in the reduction step and CaCl2 added as the flux, but also metallic Ca adhered in the sample from Ca vapor. Therefore, the metallic Ca adhered in the sample will cause wCa increase and R even larger than 1 in some cases. For eliminating the effect of metallic Ca adhered in the sample, a blank experiment was introduced for determining the amount of metallic Ca (w′Ca), which was the concentration of metallic Ca adhered in the sample and used for revising R. Figure 4 shows the relationships of w′Ca obtained from the blank experiment with temperature and time, and a revised R (RM) by subtracting w′Ca is proposed as shown in Eq. (7), which is based on Eq. (6). In order to facilitate a simple discussion, w′Ca was set as 8.1, which was the average value of w′Ca over the temperature range of 1173-1373 K.
(7)
where RM is the revised R; n is the ratio of nTiO2/nCaCl2, n=2, 3, 4, 5 or 6 in this study; wCa is the concentration of Ca in the sample after reduction (%); w′Ca is the concentration of metallic Ca adhered in the sample (%), which is 8.1 (the average value of w′Ca over the range of 1173-1373 K); wTi is the concentration of Ti in the sample after reduction (%); m is the mass of the sample after reduction (g).
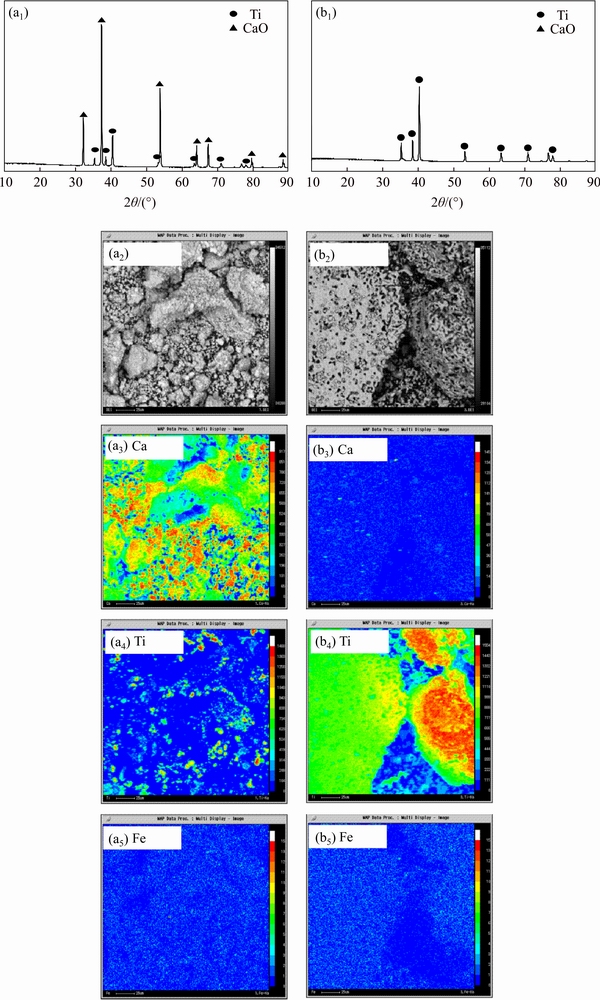
Figure 3 XRD patterns and EPMA analyses of obtained samples after reduction (a) and after acid pickling (b) (Exp. Nos. 2-9, 11-13 )
Table 3 Mass balances of Ti and Ca before and after reduction
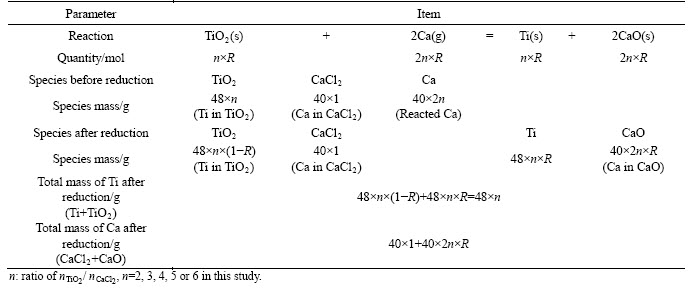
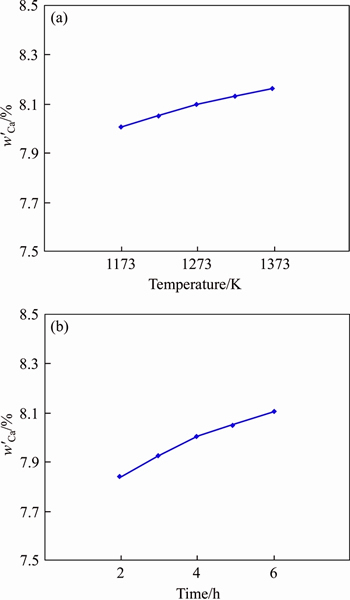
Figure 4 Concentrations of metallic Ca adhered in sample for blank experiment
4.4 Effects of various factors on RM
Chemical compositions of the obtained samples after reduction by XRF analysis are listed in Table 4.
Table 4 Chemical compositions of obtained samples after reduction
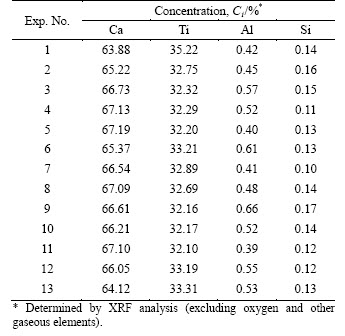
Based on Eq. (7) and Table 3, RM was obtained and the relationships of RM with reduction temperature, reduction time, and nTiO2/nCaCl2 are plotted in Figures 5(a)-(c), respectively.
Figure 5(a) shows that RM increased with the increase of reduction temperature and it increased slowly at temperatures higher than 1273 K. The reduction reaction (reaction (3)) of TiO2 by Ca vapor was influenced by both thermodynamics and kinetics aspects.
for reaction (3) was below zero and the reduction was an exothermic reaction, which would cause K decrease according to isobaric equation of
so the elevated temperatures were unfavorable to the reduction based on Le Chaterlier’s principle. However, it was beneficial to reduction because reduction reaction was faster at elevated temperatures from kinetic viewpoint. The coupled results of thermodynamics and kinetics aspects were that RM increased largely with the increase of reduction temperature below 1273 K in which the control-step of the reduction is kinetic; but it increased slowly at elevated temperatures higher than 1273 K and this indicates that the reduction reaction was mainly affected by thermodynamics aspect because elevated temperature was unfavorable to the exothermic reaction of the reduction.
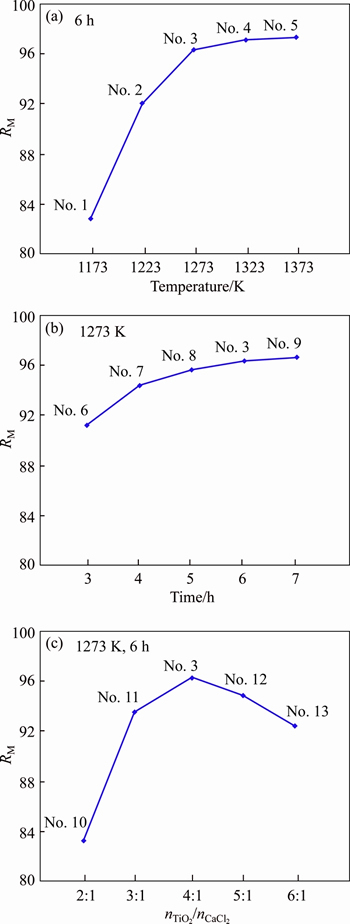
Figure 5 Relationships of RM with reduction temperature (a), reduction time (b) and nTiO2/nCaCl2 (c)
Figure 5(b) shows that RM increased with the increase of reduction time and increased slowly after 5 h and the reduction reaction nearly reached up to the equilibrium after 7 h. Therefore, reasonable reaction time is 6 h from the considerations of energy cost and production yield.
Figure 5(c) shows that RM increased with the increase of nTiO2/nCaCl2 firstly and reached up to the maximum at nTiO2/nCaCl2=4 and then decreased with the increase of nTiO2/nCaCl2. It is found that a proper amount of flux (nTiO2/nCaCl2=3-5) was beneficial to improving the strength and the porosity of the calcined pellets for reducing TiO2 effectively. The mechanism of the reduction for TiO2 in the pellets with different nTiO2/nCaCl2 was considered and assumed as shown in Figure 6. A small amount of flux (nTiO2/nCaCl2≤3) was not good for enhancing the strength of the pellets due to a little binder phase obtained in the TiO2 pellets. Furthermore, a small amount of flux (nTiO2/nCaCl2≤3) was unfavorable to improving RM because by-product CaO can not dissolve infinitely into CaCl2 (Figure 6(a)) [19] and positive reduction of TiO2 (reaction (3)) proceeded difficultly based on Le Chaterlier’s principle. A large amount of flux (nTiO2/nCaCl2≥6) was not suitable for enhancing the porosity, TiO2 particle was surrounded completely by flux CaCl2, and Ca vapor can not reach to the reduction surface of TiO2 as shown in Figure 6(c). On the other way, the possibility of the reduction of TiO2 with Ca vapor or Ca dissolved into CaCl2 was reduced and RM would be low in the cases with a large amount of flux used because only a little Ca can be dissolved into the molten CaCl2 [21]. Thus, the reasonable value of nTiO2/nCaCl2 was 3-5, in which not only RM was high enough but also the obtained pellets were of enough strength and high porosity (Figure 6(b)).
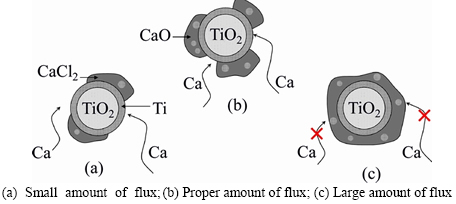
Figure 6 Schematic diagram of TiO2 pellets reduction with different nTiO2/nCaCl2:
In this study, RM was the maximum (97.61%) at 1373 K for 6 h, nevertheless the optimized production condition was 1273 K for 6 h with nTiO2/nCaCl2=4, in which RM was 96.34%, from the considerations of energy cost and production yield.
5 Conclusions
The metallothermic reduction of TiO2 in the cold pressed pellets with an indirect contact method (Ca vapor) was investigated for producing high-purity metallic Ti directly from TiO2. Experiments on the calciothermic reduction of TiO2 were carried out, the feasibility of directly producing metallic Ti from TiO2 by CPP was demonstrated; pellets were reduced homogenously and Ca vapor diffused into the porous pellets by Knudsen diffusion or the mixing diffusion of molecular diffusion and Knudsen diffusion at 1273 K; RM increased with the increase of reduction temperature over the range of 1173-1373 K; RM increased with the increase of reduction time and the reduction reaction reached up to the equilibrium nearly after 6 h; RM increased with the increase of nTiO2/nCaCl2 firstly, reached up to the maximum at nTiO2/nCaCl2=4, and then decreased with the increase of nTiO2/nCaCl2; the optimized production condition was at 1273 K for 6 h with nTiO2/nCaCl2=4, in which RM was 96.34%, from the considerations of energy cost and production yield.
Acknowledgements
The authors are grateful to Professor Toru H Okabe at Institute of Industrial Science, the University of Tokyo, Japan for his support, and my group members and colleagues at School of Metallurgy, Northeastern University, China for their support.
Contributors
The research goals were developed by ZHENG Hai-yan. ZHENG Hai-yan provided the concept, conducted the investigation, and wrote the draft of manuscript. GUO Yong-chun conducted the data curation and investigation. SHEN Feng-man provided a funding acquisition and project administration. All authors replied to reviewers’ comments and revised the final version.
Conflict of interest
ZHENG Hai-yan, GUO Yong-chun and SHEN Feng-man declare that they have no conflict of interest.
References
[1] LIDE D R. CRC Handbook of chemistry and physics [M]. Boca, Raton: CRC Press, 2009.
[2] KROLL W. The production of ductile titanium [J]. Transactions of the Electrochemical Society, 1940, 78: 35-47. DOI: 10.1149/1.3071290.
[3] MORIYA A, KANAI A. Titanium sponge production at sumitomo sitix corporation [J]. Shigen-to-Sozai (Journal of the Mining and Materials Processing Institute of Japan), 1993, 109(12): 1164-1169. DOI: 10.2473/shigentosozai. 109.1164. (in Japanese)
[4] FUKUYAMA T, KOIZUMI M, HANAKI M, KOSEMURA S. Production of titanium sponge and ingot at Toho Titanium Co. Ltd. [J]. Shigen-to-Sozai (Journal of the Mining and Materials Processing Institute of Japan), 1993, 109(12): 1157-1163. DOI: 10.2473/ shigentosozai.109.1157.
[5] ELLIOTT G R B. The continuous production of titanium powder using circulating molten salt [J]. JOM, 1998, 50(9): 48-49. DOI: 10.1007/s11837-998-0415-2.
[6] ARMSTRONG D R, BORYS S S, ANDERSON R P. Method of making metals and other elements from the halide vapor of the metal: US, 5958106 [P]. 1999-09-28.
[7] CHEN G Z, FRAY D J, FARTHING T W. Direct electrochemical reduction of titanium dioxide to titanium in molten calcium chloride [J]. Nature, 2000, 407: 361-364. DOI: 10.1002/chin.200102015.
[8] ONO K, OKABE H T, SUZUKI R O. Design, test and theoretical assessments for reduction of titanium oxide to produce titanium in molten salt [J]. Materials Trans (JIM), 2017, 58(3): 313-318. DOI: 10.2320/matertrans.MK201604.
[9] KUMSMOTO K, KISHIMOTO A, UDA T. Low-temperature electrodeposition of titanium in molten iodides [J]. Journal of Applied Electrochemistry, 2020, 50: 1209-1216. DOI: 10.1007/s10800- 020-01470-9.
[10] TAKENAKA T, SUZUKI T, ISHIKAWA M, FUKASAWA E, KAWAKAMI M. New concept for electrowinning process of liquid titanium metal in molten salt [J]. Electrochemistry (The Electrochemical Society of Japan), 1999, 67: 661-668. DOI: 10.5796/electrochemistry.67.661.
[11] ZHENG Hai-yan, ITO H, OKABE T H. Production of titanium powder by the calciothermic reduction of titanium concentrates or ore using the preform reduction process [J]. Materials Transactions (JIM), 2007, 48(8): 2244-2251. DOI: 10.2320/matertrans.MER2007115.
[12] JIAO Shu-qiang, ZHU Hong-min. Novel metallurgical process for titanium production [J]. Journal of Materials Research, 2006, 21(9): 2172-2175. DOI: 10.1557/jmr.2006. 0268.
[13] WAN He-li, XU Bao-qiang, DAI Yong-nian, YANG Bin, LIU Da-chun, SEN Wei. Preparation of titanium powders by calciothermic reduction of titanium dioxide [J]. Jouranl of Central University, 2012, 19: 2434-2439. DOI: 10.1007/ s11771-012-1293-x.
[14] OKABE T H, HAMANAKA Y, TANINOUCHI Y. Direct oxygen removal technique for recycling titanium using molten MgCl2 salt [J]. Faraday Discuss, 2016, 190: 109-126.DOI: 10.1039/C5FD00229J.
[15] LEYENS C, PETERS M. Titanium and titanium alloys [M]. Weinheim, Germany: Wiley-VCH Verlag GmbH & Co. KGaA, 2003. DOI: 10.1002/3527602119.
[16] HABASHI F. Handbook of extractive metallurgy [M]. Vol II. Weinheim, Germany: VCH Verlagsgesellschaft GmbH, 1997.
[17] BARIN I. Thermochemical data of pure substances [M]. 3rd Ed. Weinheim, Germany: Wiley-VCH Verlag GmbH, 1995. DOI: 10.1002/9783527619825.
[18] MASSALSKI T B. Phase diagrams of binary titanium alloys [M]. Metal Park, Ohio, US: ASM International Society, 1987.
[19] WENZ D A, JOHNSON I, WOLSON R D. CaCl2-rich region of the CaCl2-CaF2-CaO system [J]. Journal of Chemical & Engineering Data, 1969, 14(2): 250-252. DOI: 10.1021/je60041a027.
[20] ATKINS P, de PAULA J. Aktins’s physical chemistry [M]. 7th Ed. Oxford, Britain: Oxford University Press, 2002.
[21] SHARMA R A. Solubilities of calcium in liquid calcium chloride in equilibrium with calcium-copper alloys [J]. The Journal of Physical Chemistry, 1970, 74(22): 3896-3900. DOI: 10.1021/j100716a009.
(Edited by FANG Jing-hua)
中文导读
TiO2冷压块金属热还原法制备钛粉末
摘要:为了高效生产低成本金属钛(Ti),开展了基于非接触式二氧化钛(TiO2)冷压块金属热还原法制备Ti粉末的基础研究。本论文重点考察了非接触式金属热还原法的还原机理和还原指数(RM)与还原温度、还原时间及TiO2/CaCl2摩尔比(nTiO2/nCaCl2)的关系。研究结果显示,钙(Ca)蒸气还原TiO2 冷压块制备金属Ti粉末方法是可行的;在1273 K时,冷压块可被均匀还原,Ca蒸气在多孔冷压块内的扩散属于Knudsen扩散或分子-Knudsen混合扩散;RM 随着温度的升高和时间的延长而增加;在还原温度1273 K、还原时间6 h、nTiO2/nCaCl2=4的实验条件下,RM为96.34%;为了满足冷压块强度和高RM,冷压块内nTiO2/nCaCl2 控制在3~5较为合理。
关键词:二氧化钛;钛粉末;金属热还原;冷压块;还原指数
Foundation item: Projects(51774071, 50804007, 51974073) supported by the National Natural Science Foundation of China
Received date: 2020-05-19; Accepted date: 2020-10-16
Corresponding author: ZHENG Hai-yan, PhD, Associate Professor; Tel: +86-24-83676543; E-mail: zhenghy@smm.neu.edu.cn; ORCID: https://orcid.org/0000-0001-8454-8521