
Hybrid functional IrO2-TiO2 thin film resistor prepared by atomic layer deposition for thermal inkjet printheads
Won-Sub KWACK1, Hyoung-Seok MOON2, Seong-Jun JEONG2, Qi-min WANG1, Se-Hun KWON1
1. National Core Research Center for Hybrid Materials Solution,
Pusan National University, Busan 609-735, Korea;
2. Department of Materials Science and Engineering,
Korea Advanced Institute of Science and Technology, Daejon 373-1, Korea
Received 21 April 2010; accepted 10 September 2010
Abstract: IrO2-TiO2 thin films were prepared by atomic layer deposition using Ir(EtCp)(COD) and titanium isopropoxide (TTIP). The resistivity of IrO2-TiO2 thin films can be easily controlled from 1 500 to 356.7 μΩ·cm by the IrO2 intermixing ratio from 0.55 to 0.78 in the IrO2-TiO2 thin films. The low temperature coefficient of resistance(TCR) values can be obtained by adopting IrO2-TiO2 composite thin films. Moreover, the change in the resistivity of IrO2-TiO2 thin films was below 10% even after O2 annealing process at 600 °C. The step stress test results show that IrO2-TiO2 films have better characteristics than conventional TaN0.8 heater resistor. Therefore, IrO2-TiO2 composite thin films can be used as a heater resistor material in thermal inkjet printhead.
Key words: IrO2-TiO2 film; heating resistor; inkjet
1 Introduction
In recent years, the inkjet printer has emerged as one of the main-stream digital printing techniques. Inkjet technologies are usually divided into continuous and drop-on-demand (DOD) printing methods[1]. Among them, the majority of activity in inkjet printing today is in the DOD methods because of their low cost and high printing quality, and color capability[2]. In the thermal inkjet printer, a thin film resistor heater which converts an electrical energy into a thermal energy is generally used to eject the ink. However, because it is subjected to severe environments such as high operation temperature, chemical attack by the ink, and mechanical stresses arisen from cavitation forces during the operation[3], the choice of adequate heater materials is very important for the application of inkjet printhead.
Therefore, the heater resistor material should have a sufficient resistivity range (>150 μΩ·cm) for making heater layers of controllable thicknesses[3], and a near-zero temperature coefficient of resistance (TCR) for the stable ejection of the ink. In addition, it should have a strong resistance against both corrosion and oxidation to prevent the degradation of the inkjet printhead and a good adhesion property to the underlying SiO2 thermal insulation layer.
Up to now, several materials have been considered in the printhead, such as polysilicon, HfB2, Ta-Al and TaN[3-10]. To protect these heater resistor films against physical or chemical attacks, the passivation layers such as SiN, SiC and Ta are generally used. However, the required onset power for bubbling with these passivation layers is higher than that without passivation layers[11]. Therefore, in order to increase the heat efficiency, the passivation layers should be thinned as possible or removed. However, it should be noted that the above- mentioned heater materials such as polysilicon, HfB2, Ta-Al and TaN can not be used without the passivation-layers because they are easily oxidized and corroded at the operation environmental conditions without passivation layers. Recently, it was reported that atomic layer deposited RuO2-TiO2 has many attractive properties for using it as a heating resistor such as appropriate resistivity range with a sufficiently low temperature coefficient of resistance (TCR) values and high oxidation resistance even at high temperatures[12]. However, RuO2-based heating resistor films have a potential problem for a heating resistor application because RuO2 phases are easily decomposed to volatile RuO4 phases above 800 °C[13].
In this study, we investigated IrO2-TiO2 thin film resistor prepared by atomic layer deposition method as an alternative of RuO2-TiO2 thin films. Similar to RuO2, IrO2 also has low resistivity and good oxygen diffusion barrier properties[14]. Compared with Ru, the volatile phases of Ir form at above 1 000 °C, and no phase transformations are known in the temperature range from 4.2 to 1 000 K[14-15], which means that IrO2 remains more stable than RuO2 at higher annealing temperatures.
2 Experimental
IrO2-TiO2 films were grown on 1 300 nm-thick SiO2 film formed on Si substrates with ALD at a temperature of 230 °C and a pressure of 400 Pa. Titanium isopropoxide (TTIP) and (Ethylcyclopentadienyl) (1, 5-cyclooctadien) iridium (Ir(EtCp)(COD)) were used as Ti and Ir precursors, respectively. TTIP and Ir(EtCp)(COD) were heated at 50 °C and 85 °C, respectively. During the deposition, 100 cm3/min Ar gas was continuously supplied into the reactor. IrO2-TiO2 films were deposited by repeating a super-cycle, which consisted of two groups of sub-cycle dedicated for IrO2 and TiO2, respectively. Also, a sub-cycle consisted of several unit cycles, and in a unit cycle, four consecutive pulses were supplied. A unit-cycle for TiO2 was composed of TTIP vapor pulse with 50 cm3/min Ar carrier gas, a purge pulse with 50 cm3/min Ar, NH3 pulse with 25 cm3/min, and another 50 cm3/min Ar purge pulse, and a unit-cycle for IrO2 was composed of a Ir(EtCp)(COD) vapor pulse with 50 cm3/min Ar carrier gas, a purge pulse with 50 cm3/min Ar, a O2 pulse with 200 cm3/min, and another 50 cm3/min Ar purge pulse. Table 1 summarizes the supercycle design for ALD IrO2-TiO2 thin films. The six samples of IrO2-TiO2 thin films were prepared and labeled as A: (IrO2)0.33- (TiO2)0.67, B: (IrO2)0.56-(TiO2)0.44, C: (IrO2)0.64-(TiO2)0.36, D: (IrO2)0.72-(TiO2)0.28, and E: (IrO2)0.78-(TiO2)0.22, respectively. In the table, the letters ‘a’ and ‘b’ denote the number of unit cycles in IrO2 and TiO2 subcycles, respectively. These samples were prepared by adopting an adequate super-cycle for IrO2-TiO2 ALD, which is composed of IrO2 and TiO2 subcycle.
Table 1 Summary of super-cycle design for preparation of IrO2-TiO2 thin films
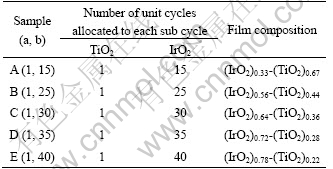
The film thickness was measured with field emission scanning electron microscopy (FESEM), and the film composition was analyzed using 9.0 MeV He2+ Rutherford backscattering spectroscopy (RBS) and Auger electron spectroscopy (AES). The microstructures of the films were determined by X-ray diffractometer (XRD). For evaluating the electrical characteristics, the sheet resistance of the films was measured through a four-point probe test. The rapid thermal annealing (RTA) was also performed on the as-deposited films in the O2 ambient for 30 min to investigate the thermal stability and oxidation resistance properties of IrO2-TiO2 thin films. After RTA process, the changes in the sheet resistance were measured by four-point probe test. In order to evaluate the TCR properties of the films, sputtered aluminum electrode (100 nm) was patterned on the IrO2-TiO2 (100 nm)//SiO2 (1 300 nm))//Si (100) substrate using a shadow mask with the interval of about 100 μm between Al dots, and the TCR values were measured through heating procedure from 25 to 170 °C in a thermostatically controlled oven using a digital multimeter (HP3457A).
3 Results and discussion
Figure 1 shows the electrical resistivities of the IrO2-TiO2 thin films depending on the IrO2 intermixing ratio. When the IrO2 intermixing ratios of the film were lower than 0.56, the film resistivity was increased drastically with decrease in the IrO2 intermixing ratio. When the IrO2 intermixing ratios in IrO2-TiO2 thin films are over 0.56, the film resistivity did not undergo a steep rate of change with increasing the IrO2 intermixing ratio. For the IrO2 intermixing ratios in the range of 0.56-0.78, the resistivity of the films can be controlled from 1500 to 356.7 μΩ·cm. Initially, the film resistivities were observed to be very high due to the high contents of the highly resistive TiO2. However, the film resistivities decreased gradually as the intermixing ratio of IrO2 increased, because IrO2 is a conductive oxide with a lower electrical resistivity of 40 μΩ·cm.
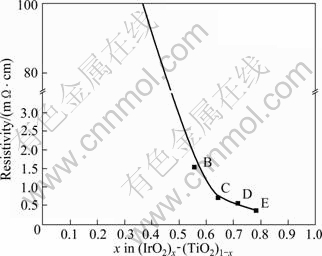
Fig.1 Resistivity of IrO2-TiO2 thin films as function of IrO2 intermixing ratio
Figure 2 shows the TCR characteristics of as-deposited IrO2-TiO2 thin films. At IrO2 intermixing ratios of less than 0.56, the TCR values of the films decreased drastically up to -1 500×10-6/K (sample A). These TCR values are far too low to allow the use of these films in heating resistors. With a thin film resistor having a high TCR, uniform heating rate is hard to be obtained. When the IrO2 intermixing ratios were higher than 0.56, the TCR values slowly varied from –420×10-6/K (sample B) to –75.35×10-6/K (sample E). With appropriate intermixing ratios (Samples C, D and E), the IrO2-TiO2 thin films showed lower absolute values of TCR values than TaN0.8(336.1×10-6/K)[12]. From the results of resistivity and TCR values, samples C, D, and E can be utilized as alternative heating resistor films.
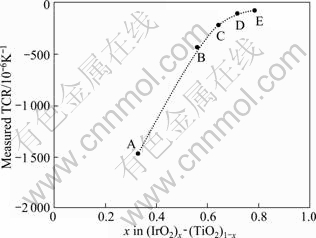
Fig.2 Variation of TCR values of IrO2-TiO2 thin films depending on IrO2 intermixing ratios (Temperatures were varied from 25 to 175 °C)
Figure 3 shows the XRD patterns of the as-deposited 50 nm-thick IrO2-TiO2 thin films depending on the IrO2 intermixing ratios. In order to compare the differences in phase, the XRD patterns of a pure TiO2 and RuO2 films were included. The as-deposited IrO2 and TiO2 films showed clear diffraction peaks, indicating that both films had a poly-crystalline structure. However, none of the IrO2-TiO2 thin films (samples A, B, C, D, E, and F) exhibited distinct diffraction peaks, indicating that the films are nanocrystalline amorphous.
Since there is small solubility between IrO2 and TiO2[16], the resistivity of the films can be changed because heating resistors are utilized at higher temperatures. Therefore, we investigated the effect of O2 annealing on the resistivities of IrO2-TiO2 films, as shown in Fig.4. The O2 annealing was performed on the samples at various temperatures for 30 min. Although some solubility of TiO2 in IrO2 exists, the change in resistivities of IrO2-TiO2 films was below 10%, which means that IrO2-TiO2 thin films can be utilized as a stable heating resistor at a high temperature operation without an additional passivation layers.
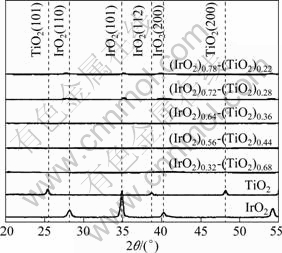
Fig.3 XRD patterns of as-deposited IrO2-TiO2 thin films prepared by atomic layer deposition
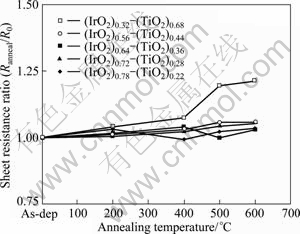
Fig. 4 Variation of sheet resistance of IrO2-TiO2 thin films depending on O2 annealing temperatures
4 Conclusions
The IrO2-TiO2 thin films with low TCR values and appropriate resistivities for heating resistor applications were prepared by controlling the intermixing ratios. The TCR values of the IrO2-TiO2 thin films were maintained at (-420 - -75.35)×10-6/K by varying the IrO2 intermixing ratios from 0.56 to 0.78, with resistivities remaining in the range of 1 500 peration- 356.7 μΩ·cm. Also, the IrO2-TiO2 thin films showed minimal resistivity change even after O2 annealing process at 600 °C. Therefore, IrO2-TiO2 thin films can serve as a suitable heating resistor material for non-passivated thermal inkjet printhead applications.
Acknowledgements
This work was supported by a grant from the Fundamental R&D Program for Core Technology of Materials funded by the Ministry of Knowledge Economy, Republic of Korea. This work was also supported by Basic Science Research program through the National Research Foundation of Korea (NRF) funded by the Ministry of Education, Science and Technology (2010-0001-226).
References
[1] LEE J D, YOON J B, KIM J K, CHUNG H J, LEE C S, LEE H D, LEE H J, KIM C K. A thermal printhead with a monolithically fabricated nozzle plate and self-aligned ink feed hole [J]. Journal of Microelectromechanical Systems, 1999, 8: 229-236.
[2] CHIU S L, WUU D S, WU Y Y. Characteristics of heater films for inkjet printhead [C]//Proceedings of SPIE: Part of the SPIE Conference on Input/Output and Imaging Technologies. Taipei, Taiwan, 1998: 61-68.
[3] ADEN J S, BOH?RQUEZ J H, COLLINS V, CROOK M D, GARC?A A, HESS U E. The third-generation hp thermal inkjet printhead [J]. Hewlett-Packard Journal, 1994: 41-45.
[4] LIM J H, KUK K, SHIN S J, BAEK S S, KIM Y J, SHIN J W, OH Y S. Investigation of reliability problems in thermal inkjet printhead [C]//Proceedings of 42nd Annual 2004 IEEE International: Reliability Physics Symposium. Phoenix, USA, 2004.
[5] WUU D S, CHAN C C, HORNG R H, LIN W C, CHIU S L, WU Y Y. Structural and electrical properties of Ta-Al thin films by magnetron sputtering [J]. Applied Surface Science, 1998, 144: 315-318.
[6] WUU D S, CHAN C C, HORNG R H. Material characteristics and thermal stability of co-sputtered Ta-Ru thin films [J]. Journal of Vacuum Science and Technology A, 1999, 17: 3327-3332.
[7] ELDRIDGE J M, FOROUHI A R, GORMAN G L, MOORE J O. Various properties of sputter-deposited HfB2 films [J]. Journal of the Electrochemical Society, 1990, 137: 3905-3909.
[8] WUU D S, LEE M L, LIN T Y, HORNG R H. Characterization of hafnium diboride thin film resistors by r.f. magnetron sputtering [J]. Materials Chemistry and Physics, 1996, 45: 163-166.
[9] CUONG N D, KIM D J, KANG B D, KIM C S, YU K M, YOON S G. Characterization of tantalum nitride thin films deposited on SiO2/Si substrates using dc magnetron sputtering for thin film resistors [J]. Journal of the Electrochemical Society, 2006, 153: G164-G167.
[10] SAHA R, BARNARD J A. Effect of structure on the mechanical properties of Ta and Ta(N) thin films prepared by reactive DC magnetron sputtering [J]. Journal of Crystal Growth, 1997, 174: 495-500.
[11] WU Y Y, CHIU S L. Effect of passivation layers on inkjet printhead [C]//Proceedings of SPIE: Part of the SPIE Conference on Input/Output and Imaging Technologies, Taipei. Taiwan, 1998: 53-60.
[12] KWON S H, KANG S W, KIM K H. Controlling the temperature coefficient of resistance and resistivity in RuO2-TiO2 thin films by the intermixing ratios between RuO2 and TiO2 [J]. Applied Physics Letters, 2008, 92: 181903.
[13] KWON S H, KWON O K, KIM J H, JEONG S J, KIM S W, KANG S W. Improvement of the morphological stability by staking RuO2 on Ru thin films with atomic layer deposition [J]. Journal of The Electrochemical Society, 2007, 154: H773-H777
[14] CHA S Y, JANG B T, LEE H C. Effect of Ir electrodes on the dielectric constants of Ba0.5Sr0.5TiO3 Films [J]. Japanese Journal of Applied Physics, 1999, 38: L49-L51.
[15] RYDENM W D, LAWSON A W, SARTAIN C C. Temperature dependence of the resistivity of RuO2 and IrO2[J]. Physic Letters A, 1968, 26: 209-210.
[16] LASSALI T A F, BOODTS J F C, BULH?ES L O S. Faradaic impedance investigation of the deactivation mechanism of Ir-based ceramic oxides containing TiO2 and SnO2 [J]. Journal of Applied Electrochemistry, 2000, 30: 625-634.
(Edited by YUAN Sai-qian)
Corresponding author: Se-Hun KWON; Tel: +82-51-510-3775; E-mail: sehun@pusan.ac.kr