
J. Cent. South Univ. (2018) 25: 2953-2961
DOI: https://doi.org/10.1007/s11771-018-3965-7

Removal of impurities from scandium solutions by ion exchange
PENG Zhen(彭桢)1, 2, LI Qing-gang(李青刚)1, 2, LI Zhao-yang(李兆洋)1, 2, ZHANG Gui-qing(张贵清)1, 2, CAO Zuo-ying(曹佐英)1, 2, GUAN Wen-juan(关文娟)1, 2
1. Laboratory of Metallurgical Separation Science and Engineering, School of Metallurgy and Environment, Central South University, Changsha 410083, China;
2. National Engineering Laboratory for Efficient Utilization of Refractory Non-ferrous Metals Resources, Central South University, Changsha 410083, China
Central South University Press and Springer-Verlag GmbH Germany, part of Springer Nature 2018
Abstract: A process of removing impurities, such as Fe, Zr, Ti, Al, Si, from scandium solution by ion exchange was proposed. Various resins’ selectivity was studied in scandium chloride solution. The results indicated that D851 resin had high selectivity of Sc at low acidity, and high selectivity of Zr at high acidity; D370 resin had high selectivity of Fe and Si in high acidity. A new technical process of removing impurities from scandium chloride solution was proposed, which includes D370 resin adsorbing Fe in high acidity, D851 resin adsorbing Zr in medium acidity and D851 resin adsorbing Sc at low acidity. Flow experimental results show that removal rates of Al, Ca, Fe, Zr, Ti and Si, were 100%, 99.6%, 100%, 100%, 99.5% and 100% respectively.
Key words: scandium; ion exchange; separation; adsorption
Cite this article as: PENG Zhen, LI Qing-gang, LI Zhao-yang, ZHANG Gui-qing, CAO Zuo-ying, GUAN Wen-juan. Removal of impurities from scandium solutions by ion exchange [J]. Journal of Central South University, 2018, 25(12): 2953–2961. DOI: https://doi.org/10.1007/s11771-018-3965-7.
1 Introduction
Scandium is one of the rare earth metals, which widely exists around the world. Scandium is rare in independent deposit while it is often associated with Ti, Al, U, Zr, W and Sn minerals [1–3]. China is abundant in Sc resources [4]. Consequently, it is of high economic significance to extract scandium from tungsten residue [5], red mud [6, 7], titania slag [8], waste acid from titanium dioxide production [9, 10], etc.
Waste acid from titanium dioxide production is the main source of scandium, despite its low concentration of Sc, ranging from only a few milligrams to tens of milligrams per liter in general. Impurities concentrations in the waste acid such as Fe, Ti, Zr, Ca, Mn, Al, are hundreds even thousands times than that of extract scandium. So, it is difficult to scandium from the waste acid, and the purification process is very complicated.
There are three ways to extract scandium from the solution which contains a number of impurities. They are precipitation, solvent extraction and ion exchange. Precipitation can only separate scandium with some specific impurities. In this way we can not get pure scandium or its compounds, but it is still an effective way to enrich and purify the solution preliminarily. Solvent extraction is the most widely used method in scandium production, as for its advantages of high efficiency, large treating volume, simple operation, consecutive and automated production, etc [11–14]. Ion exchange has superiority in dealing with solutions of low concentration, by which preparation of single rare earth with high purity is more practical than other approaches [15–19].
According to different forms of scandium in solution, different types of resins can be adopted to extract or enrich Sc [20–23]. Sulfonic cation- exchange resins are mainstream resins in dealing with Sc extraction such as 732 resin made in China, KY-1 and KY-2 resins made in Russia, Dowex-50 resin made in America. LIN et al [24] adsorbed Sc by 732 resins and desorbed with several kinds of desorption agents and separation of Sc from impurities was achieved. OCHSENKUHN et al [14] adsorbed Sc with Dowex-50 resin, and desorbed with 1.5 mol/L hydrochloric acid; Sc was separated from Fe, Al, Ca, Si, Ti, Na ,Ni, Mn, Cr, V, etc, while scandium still can not be separated from other rare earth metals. LI et al [25] separated Sc from Zr, Si and Ti by straight ion exchange, and separated Sc from Fe3+, Fe2+ and Al3+ by adding EDTA(chelating agent) to chelate Sc before the second-step ion exchange, achieving the separation of impurities from ScCl3 solution. LIU et al [26] removed U and Zr by anion exchange resin from scandium solution and the purification of the solution was achieved.
In this article, a brand-new technique to extract Sc by ion exchange was proposed, which possesses the advantages of a simple process and high removal efficiency.
2 Experiment
2.1 Materials
ScCl3 solution was prepared by mixing chemically pure Fe2O3, AlCl3, ZrOCl2·8H2O, CaO, Na2SiO3·9H2O and 99% pure Sc2O3 dissolved in hydrochloric acid. Concentration of each element is shown in Table 1. 10 common resins compared in the experiment were pretreated, which were all made in China. Resin 001 and 732 are acidic cation exchange resin. D001 is macroporous acidic cation exchange resin. 717 is basic anion exchange resin. D202 and D370 are macroporous basic anion exchange resin. D851 and D751 are macroporous chelation resin. XSC-900 is amino methylene phosphoric acid resin (APAR). Resin 331 is epoxy resin.
Table 1 Content of main compositions in solution at pH=1.5 (mass fraction, 10–6)

2.2 Experiment methods
2.2.1 Selection of resins and optimum conditions
Selection of resins and optimum condition experiments were carried out using the shake flask in thermostatic oscillator. First, 5 mL wet resin and 100 mL solution were put into a conical flask and then the conical flask was put in thermostatic oscillator. Shock strength and temperature of thermostatic oscillator were controlled. After a certain shock time, conical flask was taken out from thermostatic oscillator. Concentration of each element in all solution was analyzed by ICP-AES and concentration of H+ was determined by titration at high acidity or pH meter at low acidity. Therefore, concentration of H+ was described as [H+] at high acidity and pH at low acidity.
2.2.2 Flow experiments
Column experiments were conducted in the device installed as shown in Figure 1. 20 mL wet resin was put into φ15 mm×200 mm glass column. The feeding solution entered the column at the bottom, and the flow rate was controlled by a peristaltic pump. Effluent flowed into auto parts collector in turn. Adsorption and desorption rate can be calculated by formula:
(1)
where V0 is the volume of the feed solution; C0 is the concentration of the element; Vn is the volume of the elution in the nth collector test tube.
After the adsorption, loaded resin was washed with high-purity water or eluting reagent, and desorbed with hydrochloric acid. Desorption rate and impurities removal rate were calculated according to the ions concentration in effluent.
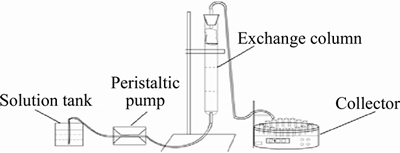
Figure 1 Diagram of experimental device of ion exchange process
3 Results and discussion
3.1 Selection of resins
To avoid the precipitation of some metal ions, the solution was controlled under a certain degree of acidity. The results about 10 different resins’ adsorption performance at CH+=3.16×10–2 (pH=1.5) and CH+=3 mol/L are shown in Tables 2 and 3.
It could be seen from Tables 2 and 3 that cation exchange resins 001, D001, 732 had good adsorptivity of Sc at low acidity. In comparison, D851 resin could separate Sc from Al and Ca at pH 1.5 while 001, D001 and 732 couldn’t as the adsorption ratio of Sc was high and only 3% when [H+]=3 mol/L. Cation exchange resins 001, D001 and 732 have good adsorptivity for some cations in the solution. Though their adsorptivities of cations were relatively good, the separation effect was worse than D851 at low acidity.
Chelation resins D851 and D751 had better separation effects as chelating effect causes a better selectivity to ions in the solution at low acidity. While compared with D751, resin D851 had a better separation coefficient of Sc to other cations. At high acidity, as the [H+] (concentration of H+) was much higher than other ions, most resins were inclined to adsorb H+. Therefore, selectivity of resins was better at high acidity. D851 had good adsorptivity to Zr, and it didn’t adsorb other elements in solution.
Obviously, basic anion exchange resins 717, D202 and D370 had poor adsorptivity to cations at low acidity. At high acidity, resin D370 had good adsorptivity to Fe and Si. Other resins didn’t have obvious separation performance. Weak basic styrene type anion exchange resin D370 can selectively adsorb Fe and Si because high concentration of HCl made Fe and Si become the form of [FeCl4]– and (H2SiO3)n, which were easily adsorbed by weak basic anion exchange resin D370.
Table 2 Adsorption rate of resin to elements at CH+=3.16×10–2 mol/L (pH=1.5)
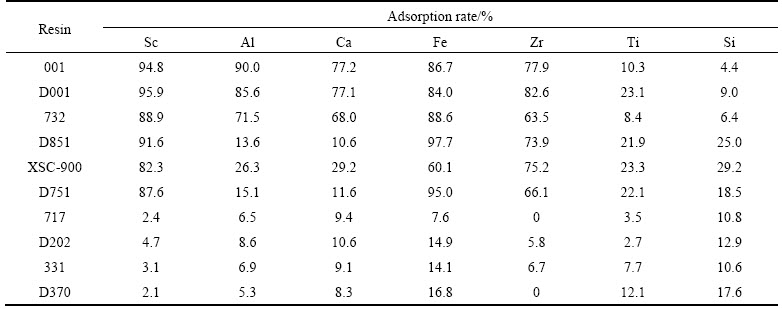
Table 3 Adsorption rate of resin to elements at CH+=3 mol/L
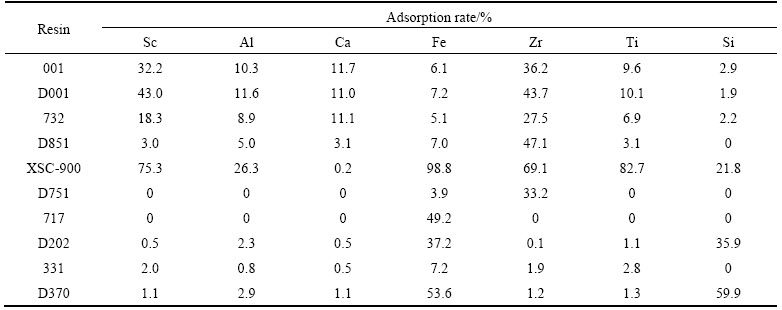
Amino methylene phosphoric acid resin XSC-900 showed preferable adsorptivity of transition metals because amino in the resin had good affinity to them. But the problem was that XSC-900 can not separate Sc from impurities. Adsorptivity of epoxy resin 331 was ordinary as the affinity of oxygen to the ions in the solution was relatively low.
Based on the analysis above, it was feasible to remove Fe and Si with D370 and remove Zr with D851 at high acidity, then selectively adsorb Sc with D851 at low acidity after neutralization, thereby separating Sc from Al, Ca, Fe, Zr, Ti and Si.
3.2 Optimum condition experiment
3.2.1 Selective adsorption of D370 resin to Fe
The adsorption rate of resin D370 to element at high acidity is shown in Figure 2. As shown in Figure 2, the adsorption rate of D370 to Fe increased greatly with the increase of [H+]. When [H+] was higher than 6 mol/L, the adsorption rate exceeded 90%. Meanwhile, D370 manifested a good absorbability to Si. When [H+] was higher than 1.5 mol/L, adsorption rate exceeded 60%. In the range of acidity, Sc, Al, Ca, Ti and Zr were hardly adsorbed. Therefore, resin D370 can remove Fe and most of the Si when acidity was 6.3 mol/L.

Figure 2 Adsorption rate of D370 resin to elements at high acidity (T=20 °C, t=180 min)
Effect of adsorption time on D370 when acidity was 6.3 mol/L is shown in Figure 3. It can be seen from Figure 3 that when adsorption time was more than 5 min, adsorption rate of Fe reached 40%, and when adsorption time was more than 60 min, adsorption rate of Fe reached 90%. This indicates that adsorption of Fe onto D370 was fast. Adsorption rate of Si to D370 increased with time linearly. When adsorption time was more than 150 min, adsorption rate of Si reached 50%.
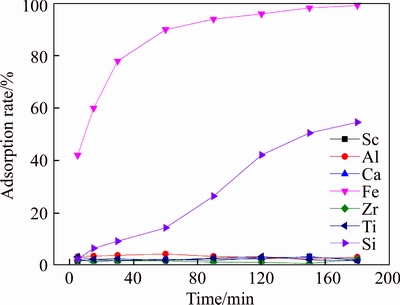
Figure 3 Effect of adsorption time on resin D370 (T=20 °C, [H+]=6.3 mol/L)
3.2.2 Selective adsorption of D851 resin to Zr
Figure 4 shows the adsorption rate of resin D851 to each element at high acidity. It can be seen from Figure 4 that the adsorptivity of D851 to Sc, Al, Ca, Fe, Ti and Si decreased gradually with the decrease of [H+]. When [H+] was higher than 1.5 mol/L, these elements were hardly adsorbed. When [H+] was 1.1 mol/L, the adsorption rate of Zr reached its zenith at 80%, while Sc was hardly adsorbed. Therefore, it was conducive to separate Zr from Sc when [H+] of the solution was 1.1 mol/L.
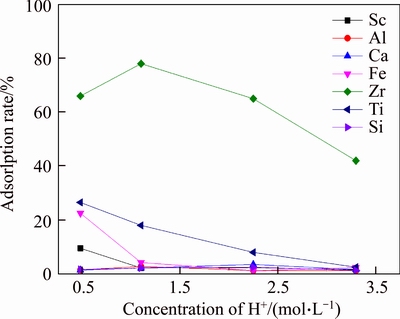
Figure 4 Adsorption rate of D851 resin to elements at high acidity (T=20°C, t=180 min)
The reason that the adsorption rate of Zr was the highest when [H+] was 1.1 mol/L was: There is a great tendency for complexation, hydrolysis and polymerization of Zr in aqueous solution [27, 28], while it exists as polymers in hydrochloric acid solution. A hydrolysis equilibrium exists in the solution:
2[Zr4(OH)8(H2O)16Cl6]2+
[Zr8(OH)20(H2O)24Cl12]+4H++4H2O (2)
When [H+] went higher, Zr turned the form of [Zr4(OH)8(H2O)16Cl6]2+from[Zr8(OH)20(H2O)24Cl12]. But when [H+] went more higher, [Zr4(OH)8(H2O)16Cl6]2+ turned to be [Zr3(OH)6Cl3]3+ and [Zr(H2O)5Cl3]+. D851 had a good adsorptivity of divalent ion [Zr4(OH)8(H2O)16Cl6]2+ in solution.
+ [Zr4(OH)8(H2O)16Cl6]2+ 
+ 2Na+ (3)
where
represents resin D851.
Separation coefficients of Sc to impurities were calculated and the results are shown in Figure 5. It can be seen from Figure 5 that when [H+] was higher than 1 mol/L, separation coefficient of Zr to Sc βZr/Sc>180, indicating that separation of Zr and Sc had been achieved. While combined with Figure 4, as [H+] went higher, adsorptivity of resin D851 to Zr went lower obviously though separation coefficient βZr/Sc increased. Therefore, optimum [H+] is 1.1 mol/L to isolate Zr from solution by D851.
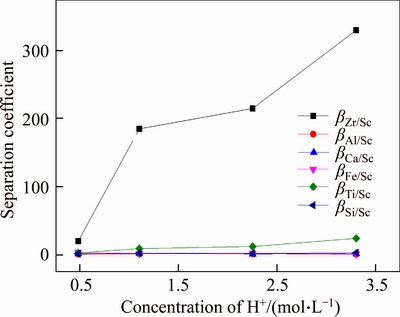
Figure 5 Separation coefficient of D851 resin to Sc at high acidity (T=20°C, t=180 min)
After the determination of [H+] at 1.1 mol/L to adsorb Zr, experiments to explore the effect of adsorption time on D851 were done and the results are shown in Figure 6. According to Figure 6, when [H+] was 1.1 mol/L, the adsorption rate of Zr to resin D851 increased with time linearly. When the adsorption time was about 150 min, the adsorption rate of Fe reached 80% and the adsorption reaction reached the equilibrium point. The adsorption rate of Ti was lower than 20% all the time though it increased continually. Adsorption rates of other elements were lower than 5% and they didn’t change a lot with time. All these show that Zr has the priority to be adsorbed by D851 at medium acidity so that Zr can be removed firstly.
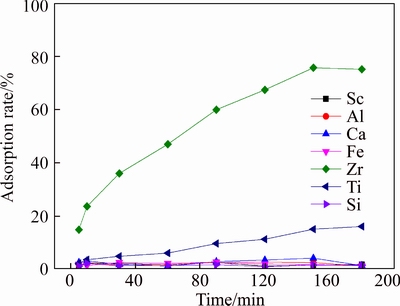
Figure 6 Effect of adsorption time on resin D851 (T=20°C, [H+]=1.1 mol/L)
3.2.3 Selective adsorption of D851 resin to Sc
Figure 7 shows the adsorption rate of resin D851 to element at low acidity. We can learn from Figure 7 that D851 had preferable adsorptivity to Fe, Sc, Zr and Ti at low acidity. Adsorption rates of Fe, Sc and Ti increased with the rise of pH. When pH=2.5, adsorption rates of Fe, Sc and Ti were 91%, 83% and 24% respectively. Adsorption rate of Zr slowly declined with the increase of pH. Adsorption rates of Al, Si and Ca had no obvious change with the increase of pH. They were all below 5%.
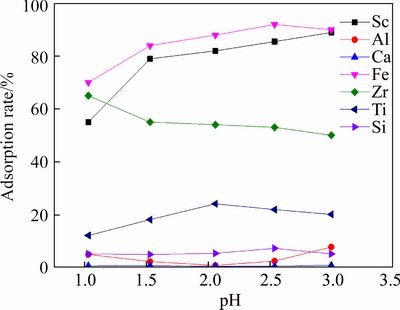
Figure 7 Adsorption rate of D851 resin to element at low acidity (T=20°C, t=180 min)
Separation coefficients of Sc to impurities at low acidity for resin D851 were calculated. The results are shown in Figure 8. According to Figure 8, separation coefficients βSc/Al and βSc/Ca increased and then decreased with the increasing pH. When solution pH=2.0, βSc/Al and βSc/Ca were larger than 450. When pH increased, βSc/Al and βSc/Ca decreased evidently. Separation coefficients of Sc to Fe, Zr, Ti and Si changed little and they were always lower than 100, so isolation effect was ordinary. Therefore, Sc can be adsorbed with resin D851 when pH=2, so it can be separated from Al and Ca.
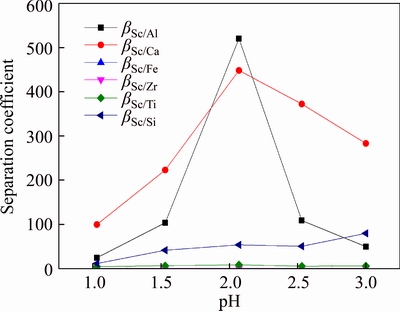
Figure 8 Separation coefficient of D851 resin to Sc at low acidity (T=20°C, t=180 min)
The effect of adsorption time on D851 adsorbing Sc at pH=2 is shown in Figure 9. As shown in Figure 9, when pH=2, adsorption rates of Fe and Sc increased remarkably with time prolonging. When adsorption time was about 30 min, adsorption rates of Fe and Sc reached 60%;after that, they grow up to 80% slowly until 180 min.
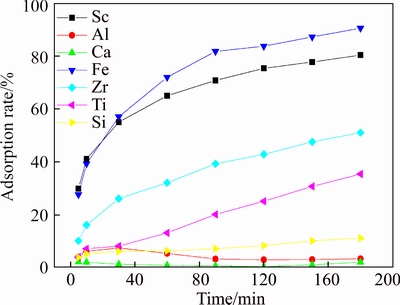
Figure 9 Effect of adsorption time on resin D851 to each element at low acidity (T=20°C, pH=2)
3.3 Flow experiments
According to the previous results, a technical process to remove impurities from scandium chloride solution was formulated with the flowchart shown in Figure 10.
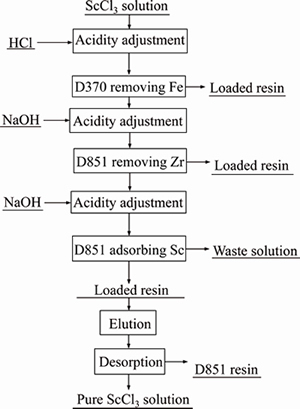
Figure 10 Flowchart of deeply purifing ScCl3 solution by ion exchange
3.3.1 Removal of Fe
Results of flow experiments removing Fe are shown in Figure 11. It can be seen from Figure 11 that in effluent, elements Sc, Al, Ti, Zr and Ca reached the concentrations of primary solution rapidly and concentration of Si rose slowly. The concentration of Fe kept very low until the volume of the effluent reached 480 mL, which showed that resin D370 has a good adsorption capacity and adsorptivity of Fe.
3.3.2 Removal of Zr
Results of flow experiments removing Zr are shown in Figure 12. It can be seen from Figure 12 that elements Sc, Al, Ti, Ca and Si reached the concentrations of the primary solution rapidly; when the volume of the solution was 320 mL, the concentration of Zr reached 5%, so the process of adsorption should be ended.
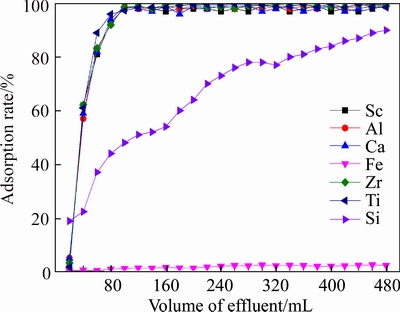
Figure 11 Effluent-curve of D370 resin adsorbing Fe (T=20°C,Vs=80 mL/h,[H+]=6.3 mol/L)
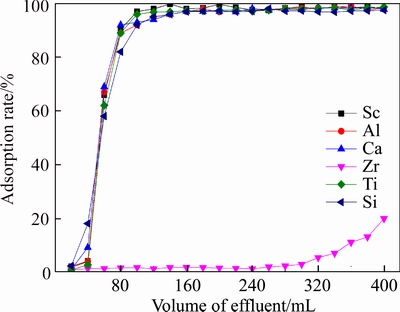
Figure 12 Effluent-curve of D851 resin adsorbing Zr (T=20°C,Vs=80 mL/h,[H+]= 1.1 mol/L)
3.3.3 Adsorption of Sc
Results of flow experiments adsorbing Sc are shown in Figure 13. It can be seen from Figure 13 that when the volume of effluent was under 320 mL, the adsorption rate of Sc was higher than 95%; but when it came to 400 mL, the adsorption rate of Sc was still higher than 90%; when it came to 500 mL, the adsorption rate of Sc was decreased to 80%. Resin D851 hardly adsorbed Ca and Al, and its adsorptivity of Si was ineffective because the adsorption rate of Si kept about 20%. Ti was adsorbed by D851 alone with Sc, which needed further separation in elution. Adsorption process should be ended at 320 mL from the result, which separated Sc from Al, Ca and most of the Si.
3.4 Elution
Loaded resin D851 was washed with distilled water and hydrochloric acid of different concentration to desorb selectively. The results are shown in Figures 14–16. We can learn from the results that concentration of HCl had a great influence on elution. From Figure 14, Si can not be desorbed by HCl, and Sc can be desorbed well when concentration of HCl was 1 mol/L, but a part of Ti would be desorbed as well. From Figure 14, when 0.5 mol/L HCl was used as desorption agent, Sc and Ti would both be desorbed. However, Figure 16 shows that when 0.1 mol/L HCl was used as desorption agent, Ti was desorbed from resin D851 but Sc wasn’t.
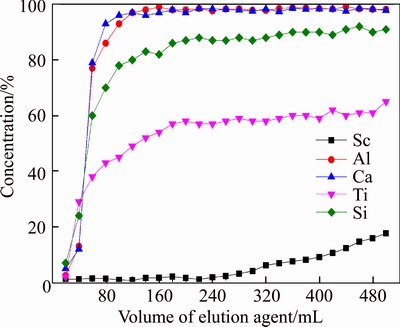
Figure 13 Effluent-curve of D851 resin adsorpting Sc (T=20°C, Vs=132 mL/h, pH=2.0)
Therefore, to desorb Sc from resin D851 and separate Sc from Ti, process of desorption can be divided into two steps: First, desorb Ti by using 16 times the volume of resin in which HCl was 0.1 mol/L; Second, desorb Sc by 16 times the volume of resin which HCl was 1 mol/L.
After the desorption conditions were determined, two-step desorption experiments were done. The results are shown in Table 4. According to the results, the removal efficiency of each element was calculated, as shown in Table 5. The concentration of Sc was kept in 288.1×10–6, and for the impurities only Ca and Ti were left 1.3×10–6 and 0.9×10–6.
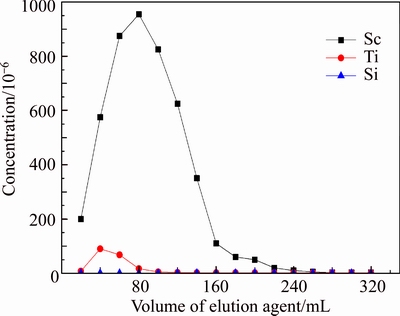
Figure 14 Effluent-curve of D851 resin in 1 mol/L HCl (T=20°C, Vs=40 mL/h)
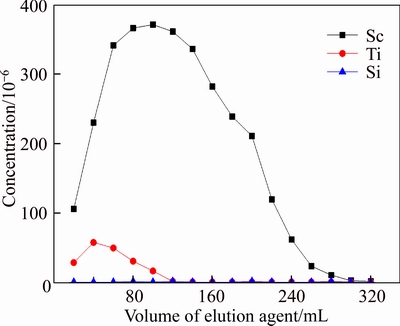
Figure 15 Effluent-curve of D851 resin in 0.5 mol/L HCl (T=20°C, Vs=40 mL/h)
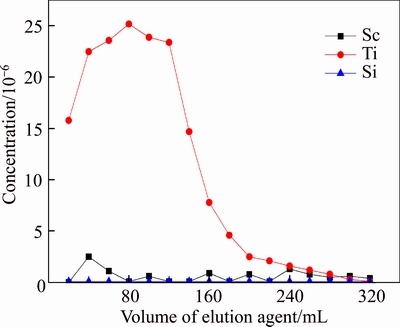
Figure 16 Effluent-curve of D370 resin in 0.1 mol/L HCl (T=20°C, Vs=40 mL/h)
Through the process that “D370 resin adsorbed Fe in high acidity, D851 resin adsorbed Zr in medium acidity, D851 resin adsorbed Sc in low acidity” and two-step elution, pure scandium chloride solution was acquired.
Table 4 Content of Sc, Ti and Si in desorption solution
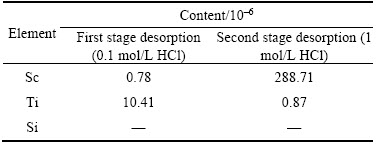
Table 5 Content and removal efficiency of each composition in scandium chloride after deep purification
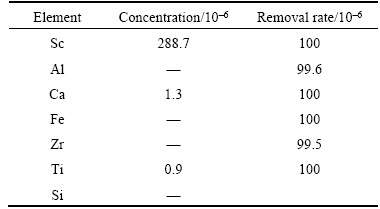
4 Conclusions
1) 10 different resins made in China were researched on their adsorption properties to Sc, Ca, Ti and Zr, etc. The results show that resin D851 has high selectivity to Sc when solution pH=1.5; D851 has high adsorption property for Zr when [H+] was 1.1 mol/L; D370 has high adsorption property to Fe when [H+] was 6.3 mol/L.
2) A brand-new method for purifying scandium chloride solution was proposed: D370 resin adsorbed Fe at high acidity, D851 resin adsorbed Zr at medium acidity; D851 resin adsorbed Sc at low acidity.
3) Flow experiments were conducted according to the above process. The results show that removal rates of Al, Ca, Fe, Zr, Ti and Si, were 100%, 99.6%, 100%, 100%, 99.5% and 100%, respectively.
References
[1] LI Li, JIANG Feng, LI Han-guang. The trend of application and development of scandium in the new century [J]. Rare Metals and Cemented Carbides, 2002(3): 38–41. (in Chinese)
[2] LI Han-guang, YIN Zhi-min. The dynamic development and development strategy of Sc [J]. Rare Metals and Cemented Carbides, 1996(1): 47–51. (in Chinese)
[3] CHI Ru-an. Mineral processing and extraction technology of rare earth [M]. Beijing: Science Publishing Company, 1996: 277–303. (in Chinese)
[4] CHEN Yan-fei, LI Mei, HU De-zhi, LIU Zhao-gang, ZHANG Dong-liang. Study on deironing with exctraction from acid leaching solution to recover scandium from tailings in Baiyenebo [J]. Nonferrous Metal (Metallurgy), 2013(11): 1–15. (in Chinese)
[5] LIU Cai-yun, FU Jian-gang. Experimental study on extraction and recovery of scandium from tungsten residue [J]. Rare Metals and Cemented Carbides, 2015(5): 4–8. (in Chinese)
[6] MEDVEDEV A S, KHAYRULLINA R T, KIROV S S. Technical scandium oxide obtaining from red mud of Urals Aluminium Smelter [J]. Tsvetnye Metally, 2015, 2015(12): 47–52.
[7] XU Lu, SHI Guang-da, LI Yuan-kun. Study of scandium pre-enrichment from red mud leached by hydrochloric acid [J]. Nonferrous Metals, 2015(1): 54–56. (in Chinese)
[8] HARTLEY C J, HAZEN W W, BAUGHMAN D R, BELITS P F, LANYK T J. Methods of recovering scandium from titanium residue streams: US20140193317 [P]. 2014-07-15.
[9] FAN Zhe-feng. Determination of scandium in titanium dioxide waste acid produced by sulfuric acid by solvent extraction ICPAES method [J]. Chinese Journal of Spectroscopy Laboratory, 2000, 17(3): 358–360. (in Chinese)
[10] WANG Wei-wei, PRANOLO Y, CHENG Chu-yong. Metallurgical processes for scandium recovery from various resources: A review [J]. Hydrometallurgy, 2011, 108(1): 100–108.
[11] WU Dong-bei, NIU Chun-ji, LI De-qian, YAN Bai. Solvent extraction of scandium(III), yttrium(III), lanthanum(III) and gadolinium(III) using Cyanex 302 in heptane from hydrochloric acid solutions [J]. Journal of Alloys & Compounds, 2004, 374(1, 2): 442–446.
[12] LI De-qian, WANG Chun. Solvent extraction of scandium (III) by Cyanex 923 and Cyanex 925 [J]. Hydrometallurgy, 1998, 48(3): 301–312.
[13] WANG Wei-wei, CHENG Chu-yong. Separation and purification of scandium by solvent extraction and related technologies: a review [J]. Journal of Chemical Technology and Biotechnology, 2011, 86(10): 1237–1246.
[14] OCHSENKUHN P M, LYBEROPULU T H, PARISSAKIS G. Selective separation and determination of scandium from yttrium and lanthanides in red mud by a combined ion exchange/solvent extraction method [J]. Analytica Chimica Acta, 1995, 315(1): 231–237.
[15] KURODA R, HIKAWA I. Separation of scandium and rare earths by anion exchange in acetic acid-HC1 mixtures [J]. Neurobiology of Learning & Memory, 2015, 118(1): 209–215.
[16] ROURKE W J, NATANSOHN S. Ion exchange method for the purification of scandium [J]. Journal of Biochemistry, 2003, 134(3): 447–457.
[17] VOIGT P, ZONTOV N, CARR J. A Process, method and plant for recovering scandium: WO089614 [P]. 2013-06-17.
[18] MEHTA V P, KHOPKAR S M. Cation exchange chromatographic separation of scandium from other elements on Dowex 50W-X8 [J]. Separation Science and Technology, 1978, 13(10): 933–939.
[19] SOKOLOVA Y V. Sorption purification of scandium(III) to remove zirconium(IV) impurity in hydrochloric acid solutions [J]. Russian Journal of Applied Chemistry, 2001, 74(3): 414–416.
[20] HERCHENROEDER L A, BURKHOLDER H R, BEAUDRY B J. Chelate additives and the purification of scandium by displacement ion-exchange chromatography [J]. Journal of the Less Common Metals, 1989, 155(1): 37–43.
[21] HERCHENROEDER L A, BURKHOLDER H R. Ion exchange purification of scandium: US4965053 [P]. 1989-10-13.
[22] XU Guang-xian. Rare earth: Volumes 1 [M]. 2nd ed. Beijing: Metallurgical Industry Press, 1995: 824–826. (in Chinese)
[23] MOLCHANOVA T V, AKIMOVA I D, SMIRNOV K M. Hydrometallurgical methods of recovery of scandium from the wastes of various technologies [J]. Russian Metallurgy, 2017, 2017(3): 170–174.
[24] LIN Wei-ming, ZENG Jun-qin. Separation of Sc with Ca, Mg, Fe, Ti, Al and Mn by ion exchange [J]. Metallurgical Analysis, 1989, 9(2): 1. (in Chinese)
[25] LI Qing-gang, LI Zhao-yang, SUN Pan. Purifying scandium chloride solution by ion exchange [J]. Journal of Northeastern University (Natural Science), 2016, 37(10): 1410–1414. (in Chinese)
[26] LIU Yu-hui, OUYANG Yu-ping, WU Xi-tao. Method for extracting scandium oxide from waste salt of titanium slag chlorination: CN104498723A [P]. 2014-12-16. (in Chinese)
[27] CHEN Xiao-wen, BAI Xin-de, DENG Ping-ye. Potential pH diagrams of Zr-H2O system at the increased temperatures [J]. Rare Metal Materials and Engineering, 2004, 33(7): 710–713. (in Chinese)
[28] XU Xin. Exctraction of Zr and Hf from acidic chloride solution using P507 [D]. Nanchang: Nanchang University, 2007. (in Chinese)
(Edited by YANG Hua)
中文导读
用离子交换法去除含钪溶液中的杂质
摘要:提出一种用离子交换深度净化钪溶液的工艺,以去除钪溶液中的铁、锆、钛、铝、钙、硅等杂质。研究了在氯化钪溶液中各种树脂的选择性,结果表明,在弱酸条件下,D851树脂对钪具有很强的选择性,随着酸浓度的升高,D851树脂对锆的选择性急剧增强,同时,D370树脂对铁和硅的选择性也随之增强。为此,提出了D370高酸除铁—D851中酸除锆—D851弱酸吸附钪的净化除杂工艺。该工艺处理后,铝、钙、铁、锆、钛、硅的去除率分别为100%、99.6%、100%、100%、99.5%、100%。
关键词:钪; 离子交换树脂; 净化; 吸附
Foundation item: Project(2015BAB19B03) supported by the National Science and Technology Supporting Plan of China
Received date: 2017-06-05; Accepted date: 2018-05-21
Corresponding author: LI Qing-gang, PhD, Professor; Tel: +86-731-88830143; E-mail: xlshlqg@csu.edu.cn