
Nanostructure and corrosion behaviors of nanotube formed Ti-Zr alloy
Won-Gi KIM, Han-Cheol CHOE
Department of Dental Materials & Research Center of Nano-Interface Activation for Biomaterials,
College of Dentistry, Chosun University, Gwangju, 501-759, Korea
Received 18 June 2008; accepted 10 March 2009
Abstract: In order to investigate the nanostructures and corrosion behaviors of Ti-Zr alloys, nanotube formed Ti-Zr(10%, 20%, 30% and 40% in mass fraction) alloys were prepared by arc melting and the condition of controlling nanostructure was at 1 000 ℃ for 24 h in argon atmosphere; formation of nanotubes was conducted by anodizing a Ti-Zr alloy in H3PO4 electrolyte with a small amount of fluoride ions at room temperature. The corrosion properties of specimens were examined through potentiodynamic test (potential range of -1 500-2 000 mV) in 0.9% NaCl solution by using potentiostat. Microstructures of the alloys were observed by optical microscope(OM), field emission scanning electron microscope(FE-SEM) and X-ray diffractometer(XRD). Diameter of nanotube does not depend on Zr content, but interspace of nanotube predominantly depends on Zr content, which confirms that ZrO2 oxides play a role to increase the interspace of nanotube formed on the surface.
Key words: nanostructure; corrosion; Ti-Zr alloy; dental implant
1 Introduction
Titanium alloys are expected to be much more widely used for implant materials in the medical and dental fields because of their superior biocompatibility, corrosion resistance and specific strength compared with other metallic implant materials. The titanium and its alloys in implant applications are mainly limited to the Ti-6Al-4V and CP-Ti alloy[1]. For medical application, titanium and Ti-6Al-4V alloy have been used since 1960s. Ti-6Al-4V alloy gradually replaced commercially high pure Ti due to the increased mechanical strength of plates, nails, screws and endoprostheses[2].
Recently, however, much concern has been paid in biocompatibility with respect to the dissolution of aluminum and vanadium ions and toxic effect[3]. Currently, other titanium alloys to be considered alternatives are Ti-6Al-4V alloys. Therefore, titanium alloys, Al- and V-free and composed of non-toxic element such as Nb and Zr as biomaterials, have been developed. Especially, Zr element belongs to the same family as Ti element in periodic table. Addition of Zr to Ti alloys leads to excellent mechanical properties, good corrosion resistance and biocompatibility[4].
The high degree of biocompatibility of Ti alloys is usually ascribed to their ability to form stable and dense oxide layers consisting mainly of TiO2. The native oxide layers on Ti are usually 2-5 nm thick and are spontaneously rebuilt in most environments whenever they are mechanically damaged. It is believed that thicker and more stable TiO2 based oxide surfaces are generally favorable for surface bioactivity[5]. Spark anodization is one of the conventional routes to increase the biocompatibility of titanium and its alloys. This process typically leads to the formation of a disordered oxide structure (irregular pores with lateral features of 1-10 μm) and several hundreds of nanometers in thickness[6]. In contrast to this approach, the electrochemical formation of novel highly ordered oxide nanotube layers has been reported for Ti anodization in fluoride containing acid electrolytes at moderate voltages [7]. Such TiO2 structures consist of arrays of nanotubes with diameter of 100 nm and thickness up to 400-500 nm.
In this work, the nanostructure and corrosion behavior of nanotube formed Ti-Zr alloy for biomaterials were researched by electrochemical methods.
2 Experimental
Ti (G&S TITANIUM, Grade 4, USA) alloys containing Zr(Kurt J. Lesker Company, 99.95% in purity) up to 10%, 20%, 30% and 40% were melted six times to improve chemical homogeneity in a vacuum arc melting furnace. Heat treatment was carried out at 1 000 ℃ for 24 h for homogenization in argon atmosphere.
The specimens for electrochemical test were prepared by using various grit emery papers and then, polished with 0.3 μm Al2O3 powder. All of polished specimens were ultrasonically cleaned and degreased in acetone.
Microstructures of the alloys were examined by optical microscope (OM, OLYMPUS BM60M, Japan) and field emission scanning electron microscope (FE-SEM, HITACHI-3000, Japan). The specimens for the OM and FE-SEM analysis were etched in Keller’s solution consisting of 2 mL HF, 3 mL HCl, 5 mL HNO3 and 190 mL H2O.
In order to identify the phase constitutents of the Ti-xZr alloys, X-ray diffractometer (XRD, Philips, X’pert Pro MPD) with a Cu Kα radiation was used.
Electrochemical experiments were carried out with conventional three-electrode configuration with a platinum counter electrode and a saturated calomel reference electrode. The sample was embedded with epoxy resin, leaving a square surface area of 10 mm2 exposed to the anodizing electrolyte of 1 mol/L H3PO4 containing 0.5% NaF. Anodization treatments were carried out using a scanning potentiostat (Model 362, EG&G Co., USA). All experiments were conducted at room temperature. In the electrochemical treatments, scanning was first swept from the open-circuit potential to desired final potential with a sweep rate of 500 mV/s, then held for 2 h at that potential. After the treatments, the anodized samples were rinsed with distilled water and dried with dry air stream.
The corrosion behaviors were investigated using potentiostat (Model 2273, EG&G Co., USA) in NaCl solution at (36.5±1.0) ℃. A conventional three-electrode cell, with a highly dense carbon as counter electrode and saturated calomel (SCE) as reference electrode, and specimen as working electrode, connected to a potentio- stat, was used to conduct the potentiodynamic test.
3 Results and discussion
Fig.1 shows the microstructures of Ti-Zr alloys with different Zr contents (10%, 20%, 30% and 40%). The microstructures of Ti-10Zr and Ti-20Zr alloy show lamellar structure and needle-like structure, and these phases change gradually to almost needle-like structure in Ti-40Zr alloy[8]. Consequently, microstructures of Ti-Zr alloys change from lamellar structure to needle- like structure as Zr content increases.
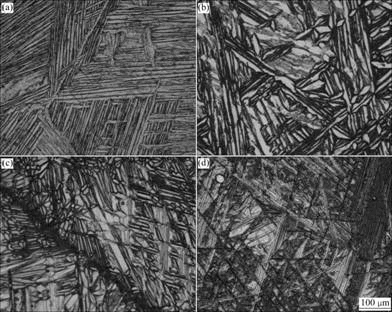
Fig.1 OM micrographs of homogenized Ti-xZr alloys: (a) Ti-10Zr; (b) Ti-20Zr; (c) Ti-30Zr; (d) Ti-40Zr
As a result of interpretation using software (Newtown Square, JCPDS win, USA) for each peak, XRD results of the homogenized Ti-xZr alloys are summarized in Fig.2, indicating the phase transformation in the Ti-xZr alloys sensitive to Zr content. This suggests that β→α transformation progresses gradually with increasing Zr content due to Zr displacement[9]. Each diffraction peak shifts to a lower angle with increasing Zr content. The absence of additional peaks is consistent with single-phase.
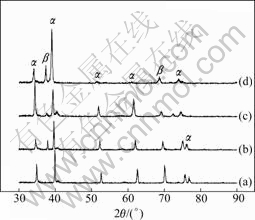
Fig.2 XRD patterns of Ti-xZr alloys: (a) Ti-10Zr; (b) Ti-20Zr; (c) Ti-30Zr; (d) Ti-40Zr
The microstructures shown in Fig.3 were taken from the Ti-20Zr, which is TiO2 nanotube layer formed on Ti substrate. In Fig.3, β phase area shows better nanotube formation than α phase area. The microstructure of Ti-20Zr in Fig.3 reveals elongated α/β phase interface. Nanotube is formed mainly on the α phase with many tubes like stacked ring. It was confirmed that the number of ring was related to nano- tube formation time and the periodicity of current oscillations[10-11]. In α phase, shape of nanotube is different from that in β phase.
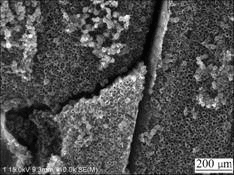
Fig.3 FE-SEM micrograph of TiO2 nanotube formed Ti-20Zr alloy
Fig.4 shows typical FE-SEM images of TiO2 nanotubes prepared by anodization of titanium at 10 V in 1 mol/L H3PO4+0.5% NaF for 2 h. Those nanotubes have an average inner diameter of 150-200 nm with a tube-wall thickness of about 20 nm.
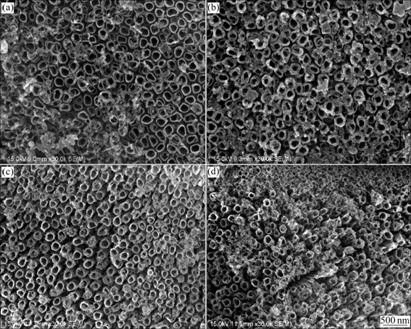
Fig.4 FE-SEM top-view images of TiO2 nanotubes formed on Ti alloy substrates: (a) Ti-10Zr; (b) Ti-20Zr; (c) Ti-30Zr; (d) Ti-40Zr
However, for Zr content of 10% (Fig.4(a)), the interspace of TiO2 nanotubes is very small, about 60 nm. As the Zr content increases, the interspace of TiO2 nanotubes increases to 70, 100 and 130 nm, respectively. Diameter of nanotube does not depend on Zr content, but interspace of nanotube predominantly depends on Zr content. It is confirmed that, ZrO2 oxides play a role to increase the interspace of nanotube formed on the surface[10-12].
Fig.5 shows the results of potentiodynamic test (potential range of -1 500-2 000 mV) in NaCl solution, which was conducted in order to investigate the effect of Zr content on the polarization curve. It can be seen in Fig.5 that the Ti-40Zr alloy has the highest resistance to corrosion. It is thought that the increase of corrosion resistance with Zr content is attributed to those thick passive films such as TiO2 and ZrO2 formed rapidly on the specimen surface[13]. Those thick passive films with thickness of dozens of nanometers can restrict the movement of metal ions from the metal surface to the solution, thus minimizing corrosion (Fig.6).
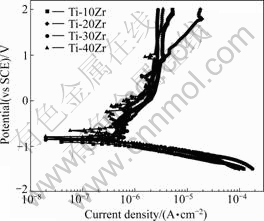
Fig.5 Polarization curves of homogenized Ti-xZr alloys after potentiodynamic test in NaCl solution at (36.5±1) ℃
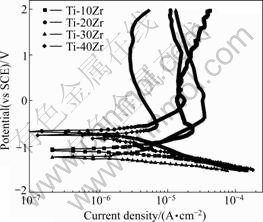
Fig.6 Polarization curves of nanotube surface formed on Ti-xZr alloys after potentiodynamic test in NaCl solution at (36.5±1) ℃
4 Conclusions
1) Microstructures observed by OM and FE-SEM change from lamellar structure to needle-like structure with increasing Zr content.
2) Microstructure changes from β phase to α phase through XRD and α phase increases with the increase of the amount of Zr content.
3) From the results of TiO2 nanotube layer formed on the Ti-xZr alloys, nanotubes have an average inner diameter of 150-200 nm with a tube-wall thickness of about 20 nm. As the Zr content increases to 10%, 20%, 30% and 40%, the interspaces of TiO2 nanotubes increase to 60, 70, 100 and 130 nm, respectively.
4) From the results of polarization behavior in the Ti-xZr alloys, the current density of nanoformed Ti-40Zr in the passive region is the highest among these alloys.
References
[1] KERRZO M A, CONROY K G, FENELON A M, FARRELL S T, BRESLIN C B. Electrochemical studies on the stability and corrosion resistance of titanium-based implant materials [J]. Biomaterials, 2001, 22: 1531-1539.
[2] EISENBARTH E, VELTEN D, MULLER M, THULL R, BREME J. Biocompatibility of β-stabilizing elements of titanium alloys [J]. Biomaterials, 2004, 25: 5705-5713.
[3] KHAN M A, WILLIAMS R L, WILLIAMS D F. The corrosion behaviour of Ti-6Al-4V, Ti-6Al-7Nb and Ti-13Nb-13Zr in protein solutions [J]. Biomaterials, 1999, 20: 631-637.
[4] KURODA D, NIINOMI M, MORIGANA M, KATO Y, YASHIRO T. Design and mechanical properties of new β type titanium alloys for implant materials [J]. Mater Sci Eng A, 1999, 243: 244-249.
[5] YANG B C, UCHIDA M, KIM H M, ZHANG X D, KOKUBO T. Preparation of bioactive titanium metal via anodic oxidation treatment [J]. Biomaterials, 2004, 25: 1003-1010.
[6] MARCHENOIR J J C, LOUP J P, MASSON J E. ?tude des couches poreuses formées par oxydation anodique du titane sous fortes tensions [J]. Thin Solid Films, 1980, 66: 357-369.
[7] GONG D, GRIMES C A, VARGHESE O K, CHEN Z , DICKEY E C. Titanium oxide nanotube arrays prepared by anodic oxidation [J]. Mater Res, 2001, 16: 3331-3334.
[8] OH M Y, KIM W G, CHOE H C. Effects of HA/TiN coating on the electrochemcial charateristics of Ti-Ta-Zr alloys [J]. Kor Inst Met Mater, 2008, 46: 691-699.
[9] DOBROMYSLOV A V, ELKIN V A. Martensitic transformation and metastable β-phase in binary titanium alloys with d-metals of 4-6 periods [J]. Scripta Materialia, 2001, 44: 905-910.
[10] TSUCHIYA H, AKAKI T, NAKATA J, TERADA D, TSUJI N, KOIZUMI Y, MINAMINO Y, SCHMUKI P, FUJIMOTO S. Anodic oxide nanotube layers on Ti-Ta alloys: Substrate composition, microstructure and self-organization on two-size scales [J]. Corrosion Science, 2009, 51(7): 1528-1533.
[11] KIM W G, CHOE H C, KO Y M, BRANTLEY W A. Electrochemical characteristics of nanotube formed Ti-Zr alloy [J]. NSTI-Nanotech, 2008, 1: 462-465.
[12] KIM W G, CHOE H C, KO Y M, BRANTLEY W A. Nanotube morphology changesfor Ti-Zr alloys as Zr content increases [J]. Thin Solid Films, 2009, 517(17): 5033-5037.
[13] KIM W G, CHOE H C, KO Y M. Electrochemical behavors of TiN coated/nanotube formed Ti-Zr alloy [J]. Kor Phys Soc, 2009, 3: 1036-1041.
Corresponding author: Han-Cheol CHOE; Tel: +82-62-230-6896; E-mail: hcchoe@chosun.ac.kr
DOI: 10.1016/S1003-6326(08)60396-9
(Edited by YANG Hua)