Trans. Nonferrous Met. Soc. China 26(2016) 938-944
Effect of T7951 secondary aging treatment on crack propagation behavior of 7055 aluminum alloy
Song ZHOU1,2, Lei WANG2, Li-yang XIE1, Li HUI2, Liang XU2
1. School of Mechanical Engineering and Automation, Northeastern University, Shenyang 110004, China;
2. Key Laboratory of Fundamental Science for National Defense of Aeronautical Digital Manufacturing Process, Shenyang Aerospace University, Shenyang 110136, China
Received 30 April 2015; accepted 15 September 2015
Abstract: The crack propagation rates of T6 peak aging and T7951 secondary aging 7055 aluminium alloys were tested under stress ratios (R) of 0.6, 0.05 and -1, respectively. The microstructures and fracture surfaces were analyzed by TEM and SEM. The results reveal that the crack propagation rate is affected by the stress ratio and microstructure such as the distribution, dimension and volume fraction of matrix precipitates, grain boundary precipitates and precipitate free zone. For both heat-treated specimens, crack propagation rate increases with the improvement of R when it is a positive value while crack propagation rate at R=-1 is much similar to that at R=0.06. The crack growth rates exhibit no obvious difference in lower stress intensity factor range (ΔK), while the difference starts to be obvious when ΔK exceeds certain value. The fracture analysis testifies a better fracture toughness for 7055-T7951 with a smaller striation space in Paris region.
Key words: 7055 aluminum alloy; T7951 secondary aging; crack propagation; fracture surface morphology
1 Introduction
Ultra-high-strength 7055 aluminum alloy has a tendency to replace the traditional 7075 aluminum alloy and has been widely applied to aircraft structural materials because of its excellent formability, high fracture toughness, enhanced corrosion resistance and damage tolerance property [1-7]. The microstructures and mechanical properties of 7055 aluminum alloy will be affected by the heat treatment remarkably [4-7]. For 7xxx series aluminum alloys, the heat treatment processes include homogenization, solid solution, quenching and aging, and the aging process can also be divided into peak aging, double aging, retrogression re-aging and deformation aging.
A number of studies about the effect of heat treatment on the microstructure, such as second phases and precipitates and their influences on mechanical properties of 7xxx series aluminum alloys were conducted through the process control during aging process [1,2,5,8-12], and the corresponding heat treatment methods were developed along T6-T73-T74- T76-T77-T79, but some key process parameters were still undisclosed because of the trade secrets.
7xxx series alloys can obtain the highest strength after peak aging, but the fracture toughness and corrosion resistance ability will decrease accordingly [12]. Double aging such as T74 and T73 can improve alloys’ fracture toughness and corrosion resistance ability with sacrificing strength. As a result, it is still an important engineering problem to achieve good matching between strength, fracture toughness, corrosion resistance ability, fatigue and damage tolerance property of 7xxx series aluminum alloys.
SCHERIBER et al [1] discussed the cyclic stress- and strain-amplitude-control fatigue response of aluminum alloy 7055-T7751 at both ambient and elevated temperatures. ZHONG et al [10] found that 7449-T7951 alloy had an excellent fatigue property with the fatigue limit of smooth specimens reaching 349 MPa for R=0.5 (R is stress ratio) and 134 MPa for R=-1.0, while it still remained 138 MPa for R=0.5 and 70 MPa for R=-1.0 by using notch specimens with the notch factor (Kt) of 3.0. The studies about the effect of under-aging and peak-aging treatments on the fatigue crack propagation behavior showed that the fatigue crack propagation rate of under-aged alloy was lower than that of peak-aged alloy which was explained in terms of the slip planarity of dislocations and the cyclic slip reversibility [11]. However, few works about the T7951 secondary aging treatment on the crack propagation behavior were published for 7055 alloy.
In the present work, the influence of T6 peak aging and T7951 secondary aging treatments on the crack propagation behaviors of 7055 aluminum alloy under three stress ratios was comparatively studied in order to understand the effect of T7951 secondary aging treatment on the crack growth rate and the reason caused by the microstructure evolution.
2 Experimental
The material used in this work was 7055 aluminium alloy plate with thickness of 10 mm. The composition of the alloy was 7.9% Zn, 2.1% Mg, 2.2% Cu, 0.15% Zr, 0.12% Fe, 0.08% Si and 0.025% Mn (mass fraction). Two groups of 7055 alloys were treated by T6 peak aging and T7951 secondary aging separately. The corresponding mechanical properties are shown in Table 1. T7951 secondary aging process involved solid solution, quenching, prestretching and artificial aging, but the detailed parameters were undisclosed.
Table 1 Mechanical properties of 7055 alloys
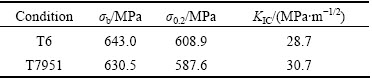
After heat treatment, the 7055 plates were machined into M(T) specimens along the rolling direction as shown in Fig. 1 to be used for the fatigue crack propagation tests according to ASTM E647 standard, and the crack propagation was along the transverse direction. Three stress ratios (R) of 0.5, 0.06 and -1.0 were chosen and three parallel specimens were prepared for each stress ratio. The fatigue crack propagation tests were conducted on the MTS-Land Mark test system in controlled laboratory air environment with a sinusoidal waveform at ambient of 27 °C and the frequency was set to 20 Hz. A traveling optical microscope was utilized to monitor the crack propagation behavior. The stress intensity factor range (△K) can be calculated according to Eq. (1):
(1)
where ΔP is the load range, B is the specimen thickness and α (α=2a/W) is the ratio of twice the crack length (2a) to the specimen width (W). The equation is effective within a certain range when 2a/W≤0.9.
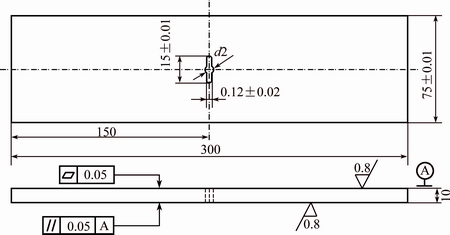
Fig. 1 Dimensions of M(T) specimen (unit: mm)
Fine microstructural features for two types of heat-treated materials were observed by a Philips Tecnai transmission electron microscope (TEM), and the fracture surfaces of the failed test specimens were comprehensively examined on a Hitachi S-3400N scanning electron microscope (SEM) to determine the macro-fracture mode and characterize the micro-scale topography and mechanisms governing fracture.
3 Results and discussion
3.1 Influence of stress ratio and heat treatment on crack propagation ratios
da/dN versus ΔK curves were utilized to describe the fatigue crack propagation ratios in Paris region. Fatigue crack growth rates for 7055 alloys under different stress ratios are shown in Fig. 2, in which the crack growth rates increase significantly with the improvement of the stress ratio when it is a positive value for both T6 and T7951 heat-treated 7055 alloys, but the crack growth rate is much similar to that at R=0.06 for a negative stress ratio R=-1.
Figure 3 shows the difference of fatigue crack growth rates between T6 and T7951 heat-treated specimens. For all three stress ratios, the crack growth rates exhibit no obvious difference for T6 and T7951 heat-treated specimens in lower stress intensity factor range, but with the increase of the stress intensity factor range, secondary aging T7951 specimen exhibits a lower crack growth rate compared with T6-treated specimen. This difference starts to be visible when ΔK exceeds about 10 MPa/m1/2 for R=0.5 and 16 MPa/m1/2 for R=0.06 and R=-1.
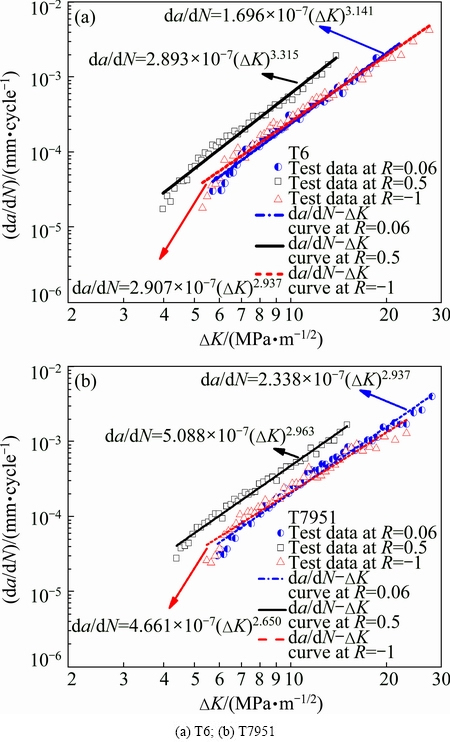
Fig. 2 Fatigue crack growth rates for 7055 alloys under different stress ratios
CHEN et al [9] and DESMUKH et al [13] found that the key reason controlling the fatigue crack growth rate of 7xxx alloy was the reversibility of dislocation motion. A part of dislocations of specimens under R=-1 are able to move backwards during unloading and compression processes on the same slip plane during tension and do not contribute to crack propagation, as a result, the crack growth rate under R=-1 is comparable to the counterpart under R=0.06 and lower than the counterpart under R=0.5, although it has a large stress range.
Crack propagation rate will be affected by the distribution, dimension and volume fraction of matrix precipitates (MPs), grain boundary precipitates (GBP) and precipitate free zone (PFZ) under the same stress level. According to the results of CHEN et al [9], the fatigue crack growth rate of 7055 aluminium alloy decreased with the increase of the precipitate size when the precipitate was coherent with the Al matrix. The basic strengthening phases of 7055-T6 aluminium alloy were uniformly dispersed GP zone with high density and η′ phase, which were easily cut repeatedly by the dislocation, giving rise to planar slip during deformation. After T7951 secondary aging, precipitate dimension increased accompanied with the enlarged particle spacing of 7055 aluminium alloy, the η phase nucleated and grew preferentially at the dislocations which were introduced by the prestretching process, eventually the matrix structure was composed of fine GP zones and η′ phases with distributed coarsen η phases, as shown in Fig. 4, which will cause a decrease of crack growth rate on 7055-T7951 aluminum alloy.
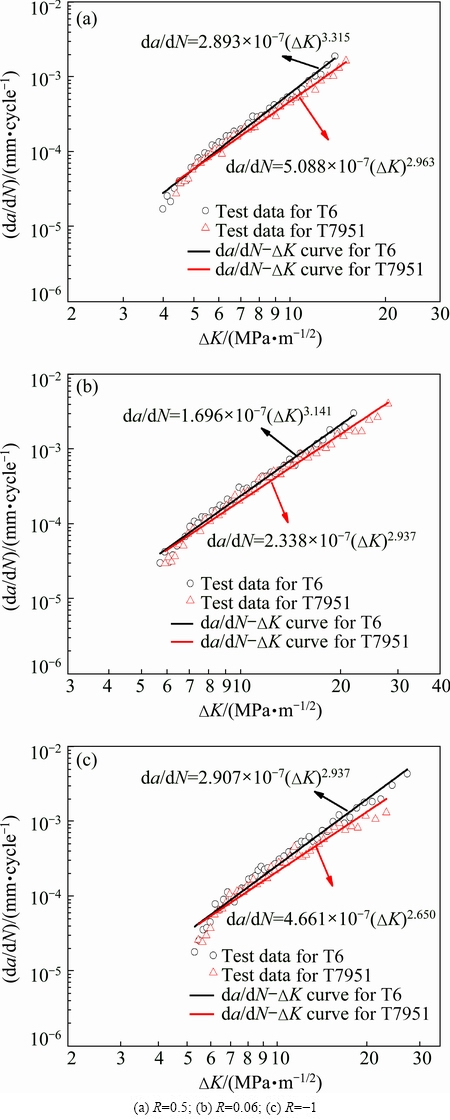
Fig. 3 Fatigue crack growth rates for 7055 alloys in different aging conditions
3.2 Comparative analysis of crack propagation fractures of T6- and T7951-treated 7055 aluminum alloy
Typical SEM observations of the overall crack propagation fracture are shown in Fig. 5. For both T6- and T7951-treated 7055 aluminium alloys, the crack propagation fractures are relatively smooth, showing a transgranular cleavage fracture mechanism.
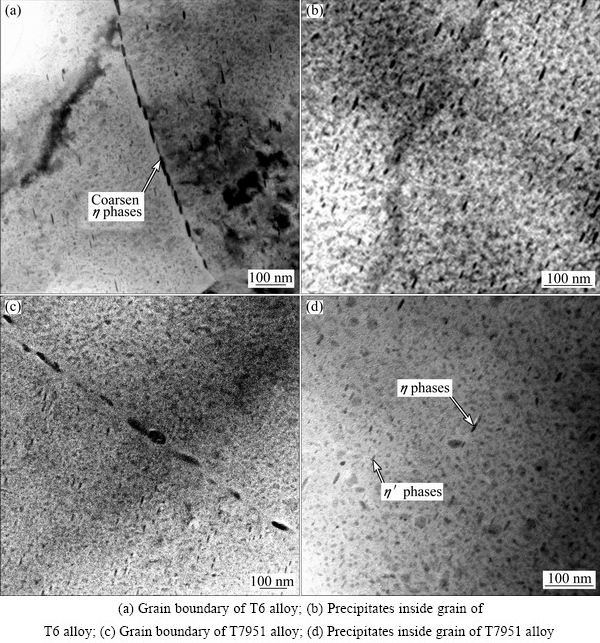
Fig. 4 TEM images of different alloys after different heat treatments
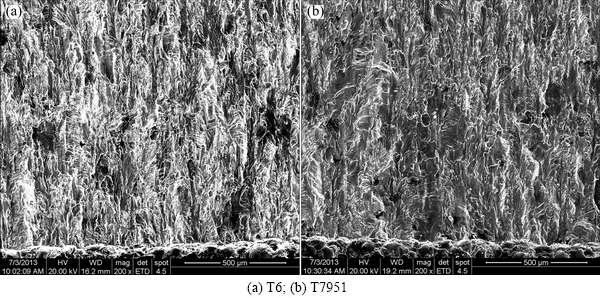
Fig. 5 Overall crack propagation fractures of different alloys
In the near-threshold region, because of the small dimension of crack and low magnitude of the crack driving force, cracks just propagate inside several grains along the dominant crystallographic slip planes depending on the dislocation sliding. As a result, both T6- and T7951-treated 7055 aluminum alloy fractures exhibit significant crystallographic characteristics of rugged crystallographic facets and river pattern, as shown in Fig. 6, the secondary cracks can be seen obviously in T6-treated alloys(Fig. 6(a)).
Fatigue striations appear in the Paris’ fatigue fractures which show a transgranular fracture nature for both specimens as shown in Fig. 7, indicating that relatively large plastic deformation exists at the crack tip.
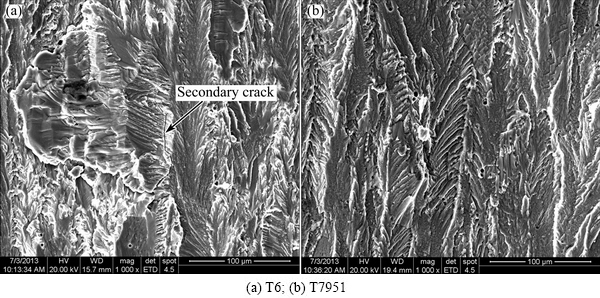
Fig. 6 Near-threshold fractures of different alloys
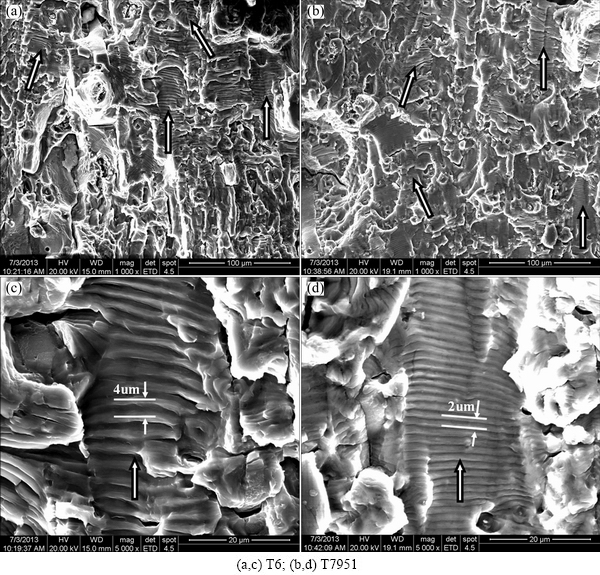
Fig. 7 Paris’ crack propagation regions of different alloys
Fatigue crack propagating directions deflect between different grains (Figs. 7(a) and (b)) but the general propagating direction is along the normal direction to the applied far-field cyclic stress. The fatigue striation space of 7055-T7951 aluminium alloy is smaller than that of the T6 counterpart, testifying a slower crack growth rate for secondary aging-treated 7055 aluminium alloy (Figs. 7(c) and (d)).
Furthermore, continuously coarsening η phases, distributed along the grain boundary for T6-treated 7055 aluminium alloy shown in Fig. 4(a), would bring about the intergranular fracture easily, as shown in Figs. 7(a) and (c). Dislocation pile-up would happen at the continuously distributed η phase chain and plastic deformation would accumulate at the crack tip, giving rise to promoting crack propagation. After T7951 secondary aging treatment, the continuously distributed η phases of GBP cluster into coarse particles with partially spheroidized structures gradually, and the PFZ becomes narrower, which can offer more resistance to deformation as compared with the grain interior at higher ΔK, leading to difficult formation of voids on coarse grain boundary particles [13]. The cutting mechanism of dislocations changes into Orowan bypassing mechanism, resulting in a decrease of dislocation pile-up at grain boundary and crack tip plastic deformation. As a result, the crack growth resistance is enhanced and the crack propagation rate declines for 7055-T7951 alloys compared with the T6-treated counterparts especially at higher ΔK in Paris region, as shown in Fig. 3.
From Fig. 8, it appears that final fracture regions exhibit a mixture of cavitations and cleavage features with rather rough fractures in comparison with the crack propagation region. Voids and dimples of varying sizes and shapes and localized microplasticity but few striations are distributed in the region randomly. The dimple volume fraction of T7951-treated 7055 aluminium alloy is higher than that of the T6-treated counterpart which also shows apparent grain boundary cracking as shown in Figs. 8(c) and (d), testifying a better fracture toughness for secondary aging-treated alloys.
4 Conclusions
1) The crack growth rates increase significantly with the improvement of the stress ratio when it is a positive value for both T6 and T7951 heat-treated 7055 alloys. The crack growth rate of alloys at R=0.06 is very close to that at R=-1.
2) The crack growth rates exhibit no obvious difference for T6 and T7951 heat-treated specimens in lower stress intensity factor range, while the difference starts to be obvious when ΔK exceeds about 10 MPa/m1/2 for R=0.5 and 16 MPa/m1/2 for R=0.06 and R=-1.
3) For both T6- and T7951-treated 7055 aluminium alloys, the crack propagation fracture surfaces are relatively smooth, showing a transgranular cleavage fracture mechanism. The near-threshold fractures exhibit significant crystallographic characteristics of rugged crystallographic facets and river pattern. Fatigue striations appear in the Paris’ fatigue fractures and striation space of 7055-T7951 aluminium alloy is smaller than that of the T6 counterpart. The final fracture region testifies a better fracture toughness for 7055-T7951 alloy.
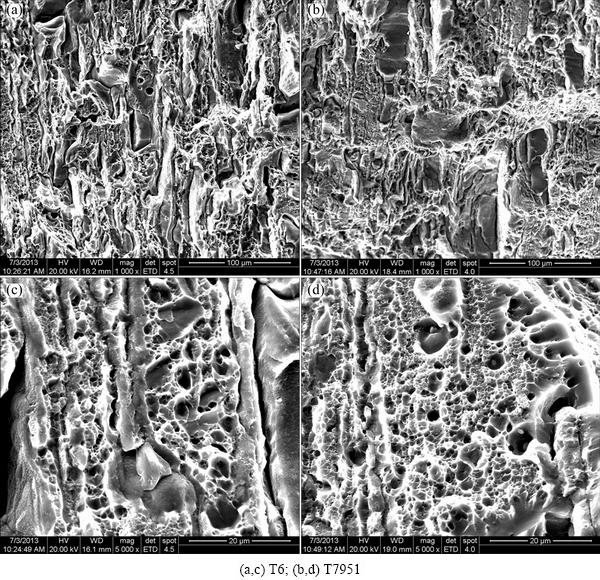
Fig. 8 Final fractures of different alloys
References
[1] SCHREIBER J M, OMCIKUS Z R, EDEN T J, SHARMA M M, CHAMPAGNE V, PATANKAR S N. Combined effect of hot extrusion and heat treatment on the mechanical behavior of 7055AA processed via spray metal forming [J]. Journal of Alloys and Compounds, 2014, 617: 135-139.
[2] MONDAL C, MUKHOPADHYAY A K, RAGHU T, VARMA V K. Tensile properties of peak aged 7055 aluminum alloy extrusions [J]. Materials Science and Engineering A, 2007, 454-455: 673-678.
[3] SHARMA M M, AMATEAU M F, EDEN T J. Corrigendum to “Mesoscopic structure control of spray formed high strength Al-Zn-Mg-Cu alloys” [J]. Acta Materialia, 2010, 58(6): 2292-2292.
[4] ROMETSCH P A, ZHANG Y, KNIGHT S. Heat treatment of 7xxx series aluminium alloys—Some recent developments [J]. Transactions of Nonferrous Metals Society of China, 2014, 24(7): 2003-2017.
[5] YAN Liang-ming, SHEN Jian, LI Zhou-bing, LI Jun-peng. Effect of deformation temperature on microstructure and mechanical properties of 7055 aluminum alloy after heat treatment [J]. Transactions of Nonferrous Metals Society of China, 2013, 23(3): 625-630.
[6] CHEN Jun-zhou, ZHEN Liang, YANG Shou-jie, SHAO Wen-zhu, DAI Sheng-long. Investigation of precipitation behavior and related hardening in AA7055 aluminum alloy [J]. Materials Science and Engineering A, 2009, 500(1): 34-42.
[7] BUHA J, LUMLEY R N, CROSKY A G. Secondary ageing in an aluminium alloy 7050 [J]. Materials Science and Engineering A, 2008, 492(1): 1-10.
[8] SRIVATSAN T S. An investigation of the cyclic fatigue and fracture behavior of aluminum alloy 7055 [J]. Materials and Design, 2002, 23(2): 141-151.
[9] CHEN Jun-zhou, ZHEN Liang, YANG Shou-jie, DAI Sheng-long. Effects of precipitates on fatigue crack growth rate of AA7055 aluminum alloy [J]. Transactions of Nonferrous Metals Society of China, 2010, 20(12): 2209-2214.
[10] ZHONG Shen, ZHENG Zi-qiao, ZHONG Li-ping, LI Hong-ping, WU Qiu-ping. High-cycle fatigue and crack initiation behavior of 7449 alloy [J]. The Chinese Journal of Nonferrous Metals, 2012, 22(10): 2734-2742. (in Chinese)
[11] HUANG Min, CHEN Jun-zhou, DAI Sheng-long, ZHEN Liang, YANG Shou-jie. Effect of aging condition on fatigue crack growth in 7055 aluminum alloy [J]. Journal of Aeronautical Materials, 2008, 28(6): 23-26. (in Chinese)
[12] FAN Xi-gang, JIANG Da-ming, ZHONG Li, WANG Tao, REN Shi-yu. Influence of microstructure on the crack propagation and corrosion resistance of Al-Zn-Mg-Cu alloy 7150 [J]. Materials Characterization, 2007, 58(1): 24-28.
[13] DESMUKH M N, PANDEY R K, MUKHOPADHYAY A K. Effect of aging treatments on the kinetics of fatigue crack growth in 7010 aluminum alloy [J]. Materials Science and Engineering A, 2006, 435(11): 318-326.
T7951二次时效对7055铝合金裂纹扩展行为的影响
周 松1,2,王 磊2,谢里阳1,回 丽2,许 良2
1. 东北大学 机械工程与自动化学院,沈阳 110004;
2. 沈阳航空航天大学 航空制造工艺数字化国防重点学科实验室,沈阳 110136
摘 要:测定应力比(R)为0.6、0.05和-1的T6峰时效和T7951二次时效7055铝合金的裂纹扩展速率,并利用透射电镜(TEM)和扫描电镜(SEM)观察材料的显微组织和断口形貌。结果显示,裂纹扩展速率受应力比、微观组织(如基体沉淀相、晶界析出相和无沉淀析出带)分布、尺寸和体积分数的影响。两种热处理状态材料在正应力比条件下的裂纹扩展速率随应力比增大而增大,当应力比R=-1时,裂纹扩展速率与R=0.06时的接近。在较小的应力强度因子变化范围内(△K),两种热处理状态材料的裂纹扩展速率差别不大,当△K超过一定值时,开始出现差异。断口分析表明,二次时效7055-T7951铝合金Paris扩展区疲劳条带间距比峰时效的小,表现出更好的断裂韧性。
关键词:7055铝合金;T7951二次时效;裂纹扩展;断口形貌
(Edited by Wei-ping CHEN)
Foundation item: Project (51405309) supported by the National Natural Science Foundation of China; Project (2013024012) supported by the Natural Science Foundation of Liaoning Province, China
Corresponding author: Li HUI; Tel: +86-24-89724608; E-mail: syhuili@126.com
DOI: 10.1016/S1003-6326(16)64190-0