Trans. Nonferrous Met. Soc. China 23(2013) 2336-2340
Synthesis and photoluminescence of Y and Cd co-doped ZnO nanopowder
Ji-ling SONG1, Jia-hong ZHENG1,2, Zhen ZHAO1, Bai-yu ZHOU1, Jian-she LIAN1
1. Key Laboratory of Automobile Materials, Ministry of Education, Jilin University, Changchun 130025, China;
2. Key Laboratory of Preparation and Applications of Environmental Friendly Materials, Ministry of Education, Jilin Normal University, Siping 136000, China
Received 17 July 2012; accepted 15 March 2013
Abstract: Y and Cd co-doped ZnO nanopowders were prepared via chemical precipitation method in order to modify the band gap and increase the luminescent intensity. The structures and optical properties of the as-synthesized samples were characterized by X-ray diffraction (XRD), X-ray photoelectron spectroscopy (XPS) and photoluminescence (PL). The effects of Y and Cd ions on the optical properties of the samples were studied. Doping of Y into ZnO evidently increases the intensity of UV emission, or co-doping of Y and Cd enhances the UV emission, narrows the band gap of ZnO and hence red shifts the UV emission at the same time. Therefore, Y and Cd co-doped ZnO nanopowders exhibit an intense violet emission in the room temperature PL spectrum, which could be a potential candidate material for optoelectronic applications.
Key words: ZnO nanopowders; chemical precipitation; optical properties
1 Introduction
In the last few years, the hexagonal wurtzite ZnO, especial doped ones, has attracted numerous attention due to its unique optical properties and promise applications in optoelectronic devices [1-3]. In order to obtain properties expected, different kinds of dopants have been doped into ZnO lattice, such as rare earth elements [4,5], metals [6-10]. One important aim of doping elements into ZnO is called as “band gap engineering (or modification)”, i.e., increasing or decreasing the band gap energy of ZnO through doping specific elements, and hence the intrinsic UV emission of ZnO in its PL spectrum can be blue or red shifted to satisfy some specific applications [11,12]. For example, it was reported that doping Mg could effectively widen the band gap of ZnO as the oxide of Mg (MgO) shows a wide band gap [13], and doping Cd could narrow the band gap of ZnO for the same reason [14]. ZnO:Y thin films were prepared via sol-gel technique and it was found that the intensity of UV emission centered at 375-380 nm was enhanced by choosing appropriate annealing temperature without obviously position changing [15]. In our previous work [16], similar phenomena were observed. However, when the UV emission blue- or red-shifted, the emission intensity usually decreased. On the other hand, enhanced emitting intensity often existed without “band gap engineering”, that is, one disadvantage is that doping of heterogeneous element can induce various lattice defects or split band gap, which decreases the UV emission intensity or broadens the emission wavelength range. So, it is sometimes difficult to red or blue shift the UV emission without decreasing its luminescent intensity. Therefore, one potential aim on band gap modification is to hold or increase the emission intensity while the intrinsic emission is blue- or red-modified through doping or co-doping.
In the present work, we intend to add two elements of Y and Cd into ZnO crystalline lattice with an expectation that an intensive and red-shifted UV emission would be attained. Y and Cd co-doped ZnO powders were synthesized via chemical precipitation method and the photoluminescence properties were investigated.
2 Experimental
In our experiments, all reagents were in analytical grade (purchased from Sinopharm Chemical Reagent Co., Ltd.) and used without further purification. Three powders of ZnO, Zn0.97Y0.03O and Zn0.94Y0.03Cd0.03O were prepared by chemical precipitation method. The synthesized process of Zn0.94Y0.03Cd0.03O was as follows: Zn(NO3)2·6H2O and the stoichiometric quantities of Y(NO3)3·6H2O and Cd(NO3)3·6H2O were dissolved in deionized water with stirring to form main mixture solution. And a solution of NH4HCO3 was added into the main mixture solution gradually. The reaction between the two solutions lasted for 4 h until adequate white precipitate was formed. The white precipitate was filtered and washed with alcohol several times and then placed in a drying oven at 50 °C for drying. At last, the dried product was annealed at 400 °C for 2 h to form the final powder.
X-ray diffraction was employed to determine the phase structure on a D/max-Rigaku XRD diffraction spectrometer with a Cu Kα line of 1.5417
and a monochromator at 40 kV and 250 mA. X-ray photoemission spectroscopy (XPS) was used to investigate the energy states of yttrium and cadmium elements in the Zn0.94Y0.03Cd0.03O powder. The XPS spectra were obtained with an ESCALABMK II (Vacuum Generators) spectrometer using unmonochromatized Al Kα X-rays (240 W). Cycles of XPS measurements were done in a high vacuum chamber with a base pressure of 133.322×10-8 Pa. PL spectra were measured at room temperature with a fluorescence spectrophotometer using a He-Cd laser of a wavelength of 325 nm as the excitation light source. All powder samples for PL measurement had the same quality and were tested under the same condition.
3 Results and discussion
X-ray diffraction patterns of the three as-synthesized powders (ZnO, Zn0.97Y0.03O and Zn0.94Y0.03Cd0.03O) are shown in Fig. 1. All the diffraction peaks correspond to the wurtzite structure of ZnO, without any secondary phases detected, which indicates that both Y and Cd elements have been doped into ZnO crystalline lattice. The Scherrer formula was used to estimate the crystallite sizes of these powders. The estimated average grain sizes are 38.9 nm, 13.9 nm and 12.9 nm, for ZnO, Zn0.97Y0.03O and Zn0.94Y0.03Cd0.03O, respectively. So these powders can be called as nanopowders. The evident reduction of grain size of the doped samples is attributed to the crystal lattice distortion caused by the doping of bigger radium Y and Cd ions into ZnO lattice, which restrains crystalline grains from growing up.
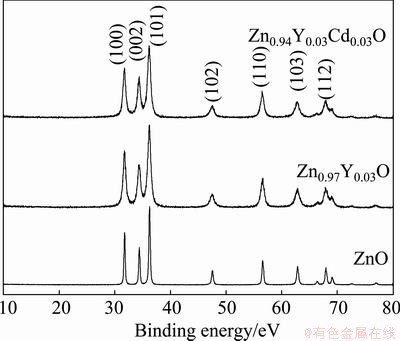
Fig. 1 XRD patterns of ZnO, Zn0.97Y0.03O and Zn0.94Y0.03Cd0.03O
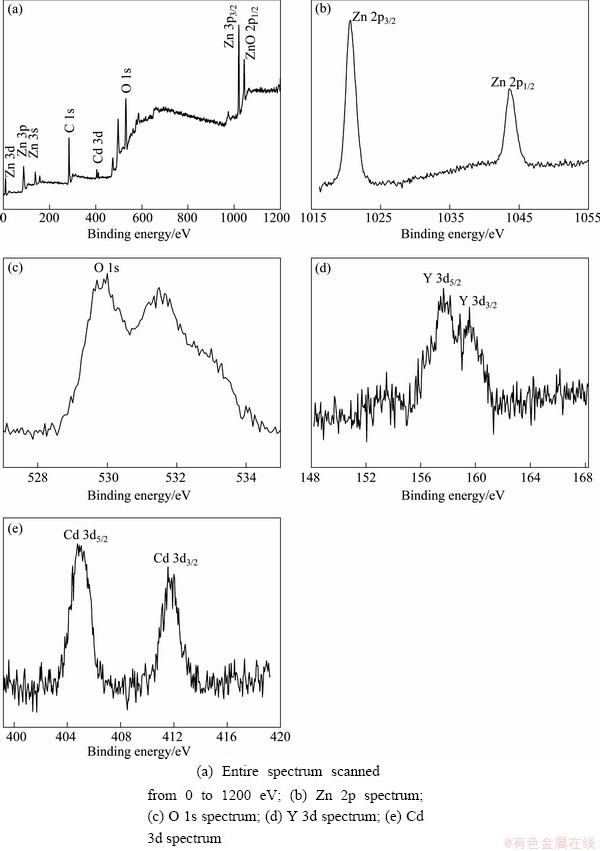
Fig. 2 XPS spectra of Zn0.94Y0.03Cd0.03O nanopowders
In order to determine the chemical states of Y and Cd in the as-synthesized nanopowders, the XPS spectra of Zn0.94Y0.03Cd0.03O were measured and are shown in Fig. 2. Figure 2(a) depicts the XPS survey spectrum, in which all the peaks of Zn, Y, Cd, O and C elements are labeled, with the adventitious carbon C 1s peak at 285.0 eV used as reference for calibrating the binding energy. Figure 2(b) shows the XPS spectrum of Zn 2p in the Y and Cd co-doped ZnO particles. The binding energies of Zn 2p3/2 and Zn 2p1/2 locate at 1020.7 eV and 1043.6 eV, respectively, and the peak separation between them is 22.9 eV, which is well located in the range of the standard reference value of ZnO [17]. It indicates that the majority of Zn ions mainly exist in the lattice of zinc oxide [18].
The spectrum of O 1s is shown in Fig. 2(c), and the peak located at 529.9 eV is attributed to O2- ions at intrinsic sites, that is, O2- ions are surrounded by zinc atoms with the full supplement of nearest-neighbor ones. The peak at 531.7 eV is usually attributed to the presence of loose oxygen on the sample surface of ZnO particle, i.e., adsorbed O2 or H2O [19,20]. As shown in Fig. 2(d), the spectrum of Y 3d has two peaks centered at 157.7 eV and 159.5 eV, which are attributed to the binding energy of Y 3d5/2 and Y 3d3/2, respectively. According to the stand binding energies of Y 3d5/2 at 156.6 eV and Y 3d3/2 at 157.4 eV, there are some shifts of 1.1 eV and 2.1 eV, respectively, in binding energy, which indicates that the binding energy of Y—O band doped in ZnO lattice is slightly different from that of pure Y2O3. Figure 2(e) depicts the XPS spectrum taken from Cd 3d regions, referencing that the binding energy of Cd 3d level in CdO is 403.0 eV [21]. The two peaks located at 404.9 eV and 411.8 eV are attributed to the binding energies of Cd 3d5/2 and Cd 3d3/2 respectively, i.e., the Cd—O bond in Cd doped ZnO lattice; and the shifts of the peaks imply that the Cd—O bond is modified by the environmental ZnO lattice. So, the XPS results also confirm that both Y and Cd elements have been incorporated into ZnO lattice.
Figure 3 shows the room temperature photo- luminescence (PL) emission spectra of the nanopowders. It is seen that all the spectra have a distinct peak of the intrinsic emission originated from the excitonic recombination, corresponding to near-band- edge (NBE) emission of zinc oxide. And a very weak deep level emission peak compared with the intrinsic emission is also observed in visible light region. The UV emission intensity of Zn0.97Y0.03O is more than twice of that of undoped ZnO, which indicates that Y-doping indeed increases the NBE intensity of ZnO [16, 22]. For Zn0.94Y0.03Cd0.03O nanopowders, their NBE emission intensity is rather lower than that of Zn0.97Y0.03O, but is still 1.5 times higher than that of pure ZnO nanopowders. It is interesting to note that the NBE peak of Zn0.94Y0.03Cd0.03O shows an apparent red shift from 384.3 nm to 396.6 nm due to Cd doping. As the synthesis effects, a strong violet emission was achieved through Y and Cd co-doping into ZnO lattice.
The mechanism for Y-doping enhancing UV emission of ZnO can be reasonably explained. It is believed that Y, as a trivalence element, can release more electrons when doping Y into ZnO lattice depresses the formation of intrinsic defects, e.g. VZn and Zni defects. And the ZnO lattice distortion caused by introduced large-sized Y ions can effectively eliminate the existence of interstitial ions like Oi and Zni. Therefore, doping Y can effectively depress DL emission and hence strengthen the intrinsic UV emission.
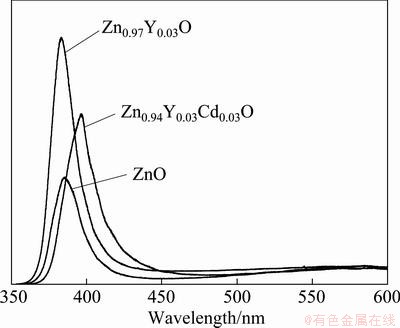
Fig. 3 PL spectra of ZnO, Zn0.97Y0.03O and Zn0.94Y0.03Cd0.03O
When Cd ions are incorporated into ZnO lattice, a strong violet emission centered about 397 nm was obtained. MAITI et al [23] observed a decrease of bandgap in Cd-doped ZnO, which was simply attributed to the fact that the bandgap of CdO (2.23 eV) is much less than that of ZnO (3.32 eV). ZHENG et al [14] deduced that the observed red shift of UV emission peaks was ascribed to the alteration of the Fermi level to a lower position of conduction band, which can be explained by the decrease of free electrons in the bottom of conduction band caused by Cd atoms entering into ZnO lattice.
To sum up, Y element existing in ZnO lattice provides more free excitons to improve the carrier concentration and hence enhances UV emission and constrains the deep-level emission; on the other hand, Cd element in the lattice reforms defect centers through trapping carriers to generate band defects or intrinsic ones and red shifts UV emission peak. In other words, Y provides carrier and Cd depletes it. As a result, co-doping the two elements in to ZnO inevitably causes a competition of carrier concentration. As seen in Fig. 3, the intensity of NBE emission of Zn0.94Y0.03Cd0.03O sample decreases compared with that of Zn0.97Y0.03O, which can be attributed to the reduction of free electrons, and certainly UV emission red shifts as a result of the band defects caused by the existing of Cd ions. By the cooperation effect of Y and Cd, a strong violet emission was observed. Practically, the co-doped ZnO with an intensive violet emission can be a potential candidate for violet emission devices.
4 Conclusions
Zn0.94Y0.03Cd0.03O nanopowders were synthesized by chemical precipitation method. The XRD measurements confirmed that the as-grown powders have a wurtzite ZnO structure. The XPS results demonstrated the presence of both Y and Cd ions in ZnO lattice, which results in an evident decrease of the particle size from 38.9 nm to 12.9 nm. Room temperature PL spectra of the samples showed that doping Y can effectively enhance the UV emission and depress the DL emission, and Y-Cd co-doping can increase the emission intensity, especially, substantially redshift the UV emission from 384.3 nm to 396.6 nm. So, an appropriate co-doping of Y and Cd elements into ZnO lattice endows the material an intense violet emission, which may be a potential candidate for optoelectronic applications.
References
[1] TENG X M, FAN H T, PAN S S, YE C, LI G H. Influence of annealing on the structural and optical properties of ZnO:Tb thin films [J]. J Appl Phys, 2006, 100: 053507.
[2] WANG D D, XING G Z, YANG J H, YANG L L, GAO M, CAO J, ZHANG Y J, YAO B. Dependence of energy transfer and photoluminescence on tailored defects in Eu-doped ZnO nanosheets-based microflowers [J]. J Alloy Compd, 2010, 504: 22-26.
[3] ANEESH P M, JAYARAJ M K. Red luminescence from hydrothermally synthesized Eu-doped ZnO nanoparticles under visible excitation [J]. Mater Sci B, 2010, 33: 227-231.
[4] CHEN Y, XU X L, ZHANG G H, XUE H, MA S Y. Blue shift of optical band gap in Er-doped ZnO thin films deposited by direct current reactive magnetron sputtering technique [J]. Physica E, 2010, 42: 1713-1716.
[5] GAO S, ZHANG H, DENG R, WANG X, SUN D, ZHENG G. Engineering white light-emitting Eu-doped ZnO urchins by biopolymer-assisted hydrothermal method [J]. Appl Phys Lett, 2006, 89: 123-125.
[6] ZHENG B J, LIAN J S, JIANG Q. Highly transparent and conductive Zn0.86Cd0.11In0.03O thin film prepared by pulsed laser deposition [J]. J Supercond Nov Magn, 2011, 24: 1627-1632.
[7] WANG C C, LIU M, MAN B Y, CHEN C S, JIANG S Z, YANG S Y, GAO X G, XU S C, HU B, SUN Z C. Effect of oxygen pressure on the structural, optical and magnetic properties of pulsed laser ablated ZnCoO thin films [J]. J Alloy Compd, 2012, 520: 158-163.
[8] KAUSHIK V K, GANGULI T, KUMAR R, MUKHERJEE C, SEN P K. Growth and characterization of ZnO and MgxZn1-xO thin films by aerosol assisted chemical vapor deposition technique [J]. Thin Solid Films, 2012, 520: 3505-3509.
[9] KARAAGAC H, YENGEL E, ISLAM M S. Physical properties and heterojunction device demonstration of aluminum-doped ZnO thin films synthesized at room ambient via sol–gel method [J]. J Alloy Compd, 2012, 521: 155-162.
[10] SHINDE S S, RAJPURE K Y. Fabrication and performance of N-doped ZnO UV photoconductive detector [J]. J Alloy Compd, 2012, 522: 118-122.
[11] MAKINO T, SEGAWA Y, KAWASAKI M, OHTOMO A, SHIROKI R, TAMURA K, YASUDA T, KOINUMA H. Band gap engineering based on MgxZn1-xO ternary alloy films [J]. Appl Phys Lett, 2001, 78: 1237-1239.
[12] TAN S T, CHEN B J, SUN X W, FAN W J, KWOK H S, ZHANG X H, CHUA S J. Blue shift of optical band gap in ZnO thin films grown by metal-organic chemical-vapor deposition [J]. J Appl Phys, 2005, 98: 013505.
[13] HUANG K, TANG Z, ZHANG L, YU J Y, LV J G, LIU X S, LIU F. Preparation and characterization of Mg-doped ZnO thin films by sol–gel method [J]. Appl Surf Sci, 2012, 258: 3710-3713.
[14] ZHENG B J, LIAN J S, ZHAO L, JIANG Q. Optical and electrical properties of Zn1-xCdxO thin films prepared by PLD [J]. Appl Surf Sci, 2012, 257: 5657-5662.
[15] HSIEH P T, CHUANG R W K, CHANG C Q, WANG C M, CHANG S J. Optical and structural characteristics of yttrium doped ZnO films using sol–gel technology [J]. J Sol-Gel Sci Techn, 2011, 58: 42-47.
[16] ZHENG J H, SONG J L, JIANG Q, LIAN J S. Enhanced UV emission of Y-doped ZnO nanoparticles [J]. Appl Surf Sci, 2012, 258: 6736-6738.
[17] WANGY, SUN L, KONG L G, KANG J F, ZHANG X, HAN R Q. Room-temperature ferromagnetism in Co-doped ZnO bulk induced by hydrogenation [J]. J Alloy Compd, 2006, 423: 256-259.
[18] LEUNGA C Y,
A B, LEUNG Y H, DING L, YANG C L, GE W K. Influence of the carrier gas on the luminescence of ZnO tetrapod nanowires [J]. J Cryst Growth, 2006, 290: 131-136.
[19] JING L Q, XU Z L, SHANG J, SUN X J, CAI W M, GUO H C. The preparation and characterization ofZnOultrafineparticles of ZnO ultrafine particle [J]. Mater Sci Eng A, 2002, 332: 356-361.
[20] JIN Y X, CUI Q L, WEN G H, WANG Q S, HAO J, WANG S, ZHANG J. XPS and Raman scattering studies of room temperature ferromagnetic ZnO:Cu [J]. J Phys D, 2009, 42: 215007.
[21] BOSE D N, HEDGE M S, BASU S, MANDAL K C. XPS investigation of CdTe surfaces: Effect of Ru modification [J]. Semicond Sci Techn, 1989, 4: 866-870.
[22] YANG J H, WANG R, YANG L L, LANG J H, WEI M B, GAO M, LIU X Y, CAO J, LI X, YANG N N. Tunable deep-level emission in ZnO nanoparticles via yttrium doping [J]. J Alloy Compd, 2011, 509: 3606-3612.
[23] MAITI U N, GHOSH P K, AHMED S F, MITRA M K, CHATTOPADHYAY K K. Structural, optical and photoelectron spectroscopic studies of nano/micro ZnO:Cd rods synthesized via sol-gel route [J]. J Sol-Gel Sci Techn, 2007, 41: 87-92.
钇、镉共掺杂氧化锌纳米粉体的合成及光学性能
宋季岭1,郑佳红1,2,赵 真1,周柏玉1,连建设1
1. 吉林大学 汽车材料教育部重点实验室,长春 130025;
2. 吉林师范大学 环境友好材料制备及应用省部共建教育部重点实验室,四平 136000
摘 要:为了研究能隙修改和发光强度之间的关系,通过化学共沉淀法制备钇、镉共掺杂的氧化锌纳米粉体。采用XRD、XPS及PL对样品的晶体结构和光学性能进行研究。通过对钇、镉离子对氧化锌光学性能影响的研究发现,钇的掺入能够有效地增强氧化锌的紫外发光,钇、镉共掺杂在增强发光的同时,还使得氧化锌的带隙窄化,使得紫外发光红移,从而使得样品的室温光致发光图谱呈现出增强的紫光发射。该材料有望应用在制备光电器件方面。
关键词:氧化锌纳米粉体;化学共沉淀;光学性能
(Edited by Hua YANG)
Foundation item: Project (2010CB631001) supported by the National Basic Research Program of China; Project (20121098) supported by the Foundation from of Key Laboratory of Preparation and Applications of Environmental Friendly Materials, Ministry of Education, China; Project supported by Graduate Innovation Fund of Jilin University, China
Corresponding author: Jian-she LIAN; Tel/Fax: +86-431-85095876; E-mail: lianjs@jlu.edu.cn
DOI: 10.1016/S1003-6326(13)62738-7