J. Cent. South Univ. Technol. (2008) 15: 11-14
DOI: 10.1007/s11771-008-0003-1

Microstructures and magnetic properties of [SiO2/FePt] 5/Ag thin films
FAN Jiu-ping(范九萍), XU Xiao-hong(许小红), JIANG Feng-xian(江凤仙),
TIAN Bao-qiang(田宝强), WU Hai-shun(武海顺)
(School of Chemistry and Materials Science, Shanxi Normal University, Linfen 041004, China)
Abstract:[SiO2/FePt]5/Ag thin films were deposited by RF magnetron sputtering on the glass substrates and post annealing at 550 ℃ for 30 min in vacuum. Vibrating sample magnetometer and X-ray diffraction analyser were applied to study the magnetic properties and microstructures of the films. The results show that without Ag underlayer [SiO2/FePt]5 films deposited onto the glass are FCC disordered; with the addition of Ag underlayer [SiO2/FePt]5/Ag films are changed into L10 and (111) mixed texture. The variation of the SiO2 nonmagnetic layer thickness in [SiO2/FePt]5/Ag films indicates that SiO2-doping plays an important role in improving the order parameter and the perpendicular magnetic anisotropy, and reducing the grain size and intergrain interactions. By controlling SiO2 thickness the highly perpendicular magnetic anisotropy can be obtained in the [SiO2 (0.6 nm)/FePt (3 nm)]5/Ag (50 nm) films and highly (001)-oriented films can be obtained in the [SiO2 (2 nm)/FePt (3 nm)]5/Ag (50 nm) films.
Key words:[SiO2/FePt]5 multilayer films; SiO2-doping; Ag underlayer; (001) orientation
1 Introduction
FePt films with the L10 ordered structure are attractive candidates for ultrahigh density magnetic recording because of the high magnetic anisotropy of L10 phase[1-2]. Recently, many researches have been done on the FePt films with different matrixes, such as Ti, CrW and Ag. The obtained results indicate that the magnetic properties strongly depend on the texture of the underlayer[3-5]. It has been reported that the annealed FePt/Ag multilayer grown onto single crystal MgO [001] substrates consists of L10 FePt granular grains magnetically decoupled by the Ag matrix[6-8]. However, it is more practical to adopt glass substrates. Furthermore, higher anisotropy energy is needed to retain thermal stability. It also requires that the magnetic particles must be isolated to reduce intergrain interaction, which leads to lower media noise. It has been reported that high- density media of FePt:SiO2 granular thin films, which consist of high anisotropy FePt particles, embedded in a SiO2 matrix, can be obtained[9-10] .
In the previous studies, the Ag underlayer was found quite effective to grow the (001)-oriented FePt[11]. Moreover, nonmagnetic doping was of great benefit to getting small particle size and isolating magnetic grains[12]. This would give us some ideas of how to combine the effect of SiO2-doping and Ag underlayer together. So in this work, a triple system ([SiO2/FePt]5/Ag nanocomposite films) on the glass substrates and subsequent postannealing was investigated. Especially, great emphasis was placed on the effect of adding Ag underlayer and SiO2 sublayer thickness on the structures and magnetic properties of the films.
2 Experimental
A series of [SiO2/FePt]5/Ag multilayer films were deposited on the glass substrates by RF magnetron sputtering at room temperature. The base pressure of the deposition chamber was 5×10-5 Pa and high purity argon gas flow with a pressure of 1.3 Pa was used during sputtering. A FePt composite target, separated SiO2 and Ag targets, were used. After deposition, the samples were annealed at 550 ℃ for 30 min under a pressure of 2×10-4 Pa.
The thickness of the layers was measured by an Ambios Tech XP-2 surface profiler; the structures of the films were analyzed by Bruker D8 X-ray diffractometer with Cu Kα radiation, and the magnetic properties were measured by a Lakeshore 7407 vibrating sample magnetometer.
3 Results and discussion
[SiO2 (x nm)/FePt (3 nm)]5/Ag (50 nm) films with x =0.2, 0.4, 0.6, 0.8, 2.0, 3.0 nm were fabricated, in which the total thickness of FePt layer was hold at 15 nm. In order to check the effect of SiO2 on the magnetic properties, the thickness of SiO2 was changed. Fig.1 shows the out-of-plane coercivities (Hc⊥ ) and in-plane ones (Hc∥) of [SiO2 (x nm)/FePt (3 nm)] 5/Ag (50 nm) films as a function of SiO2 thickness. It can also be seen that the out-of-plane coercivities are larger than the in-plane ones. Among the investigated samples, the SiO2 film with thickness of 0.6 nm has the highest value for coercivities. When x<0.6 nm, the perpendicular and in-plane coercivities of the films increase with increasing x. This may be due to the addition of SiO2 acting as pinning sites to impede the domain wall motion and increasing the coercivities; when x>0.6 nm, all the perpendicular and in-plane coercivities of the films decrease with increasing x. The thicker nonmagnetic SiO2 layers in the sample restrain the ordering growth of FePt during annealing. Besides the high activation energy needed to break the deposited SiO2 layers, the broken SiO2 layers also isolate the FePt particles, which will produce a higher activation energy barrier to the disorder-order transformation, therefore leading to decrease in coercivities. When x=2.0 nm the difference between Hc⊥ and Hc∥ is the largest. These results indicate that the appropriate nonmagnetic layer thickness is effective in promoting chemical ordering of the L10 FePt structure. Usually, the variation of coercivities may be due to the variation of the order parameter and grain size.
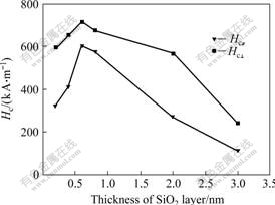
Fig.1 Coercivity of [SiO2 (x nm)/FePt (3 nm)] 5/Ag (50 nm) with thickness of SiO2 layer
The XRD patterns of [SiO2 (x nm)/FePt (3 nm)]5/Ag (y nm) (x=0.2, 0.6, 2.0, 3.0; y=0, 50) thin films are shown in Fig.2. The [SiO2/FePt]5 thin films without Ag underlayer exhibit the disordered FCC structure. With the addition of Ag underlayer, FePt (001) superlattice peaks emerge, which suggests that after adding Ag underlayer, [SiO2/FePt]5 films undergo a phase transition from the disordered FCC to the ordered FCT phase. The main reason is the crystal lattice unmatching between Ag and FePt. The longer crystal lattice parameter of Ag is beneficial to perpendicularity tropism of FePt. Furthermore, in the [SiO2 (x nm)/FePt(3 nm)]5/Ag(50 nm) films, it is interesting to find that the orientation of FePt grains strongly depends on the thickness of SiO2 layer. With increasing the thickness of SiO2 layer from 0.2 to 2.0 nm, the relative intensity of (001) peak increases, which indicates that the ordering degree of FePt grains increases. These results reveal that the appropriate diffusion of SiO2 atom will induce vacancies and increase the mobility of Fe and Pt atoms, which make nucleus when annealed, release free energy and thus enhance the kinetics for phase transformation. When the thickness of SiO2 layer increases up to 2.0 nm, the ratio of the intensity of the (001) peak to that of the (111) peak (I001/I111) gets the maximum value. Thus, a conclusion can be drawn that the development of the FCT (001)

Fig.2 XRD patterns of [SiO2 (x nm)/FePt (3 nm)]5/Ag (y nm) thin films: (a) x=3.0, y=50; (b) x=2.0, y=50; (c) x=0.6, y=50; (d) x=0.2, y=50; (e) x=0.2, y=0
orientation is determined by the combined effect of the Ag underlayer and appropriate SiO2 diffusion. With further increasing SiO2 layer thickness up to 3.0 nm, the relative intensity of (001) peak decreases. This result suggests that thicker SiO2 layer will not result in further development of (001) texture.
To demonstrate the transformation process quantitatively, an order parameter (S) is introduced. Generally, quantitative values of S are obtained from the comparison between the integrated intensities of the superlattice and fundamental reflections of XRD. An approximate relation between S and c/a, which can be obtained from Ref.[13] is adopted
(1)
where (c/a) f is the c/a value of fully ordered FePt films, and it is 0.956. (c/a) is the value of partially ordered FePt film. Fig.3 shows S as a function of the SiO2 layer thickness. It can be clearly observed that S exhibits a maximum value at the SiO2 layer thickness of 0.6 nm. The strain is defined as [(c-c0)/c0]×100%, where c0 is the standard lattice parameter c-axis of the L10 phase of equiatomic FePt. S is the measured lattice parameter c-axis of the L10 (001) plane. The strain dependence on SiO2 layer thickness is also be shown in Fig.3. It indicates that when the thickness of SiO2 sublayer is suitable, S is the largest and the strain is minimized. The corresponding minimum strain is probably associated with the total free energy minimization, which determines the film texture.
Fig.4 shows the relationship between the perpendicular magnetic anisotropy (Ku) and the particle size dependence on the layer thickness of SiO2. Perpendicular magnetic anisotropy is estimated by[14-15]
Hc⊥ =[1-(Dp/D) 0.77] (0.96Ku/Ms) (2)
where Ms is the saturation magnetization; D is the grain size and Dp is the critical particle diameter for superparamagnetism, which is estimated to be
Dp=(150 KT/πKu)1/3 (3)
where K is the Boltzmann constant. For the FePt alloy, Dp is about 3 nm at room temperature. The perpendicular magnetic anisotropy of the films increases initially with increasing x from 0.2 to 0.6, and then decreases with an increase of x from 0.6 to 3.0. However, it is interesting to find that the highest perpendicular magnetic anisotropy is obtained when x is fixed at 0.6. The grain size of [SiO2 (x nm)/FePt (3 nm)]5/Ag (50 nm) films is from the half width of the (001) XRD peaks by the Scherrer formula[9]
d=0.9λ/(Bcos θ) (4)
The grain size first increases and then decreases with the increase of SiO2 content. As a result, the maximum of grain size is observed when the thickness of SiO2 is 0.6 nm. It may be due to the two conflicting ways in which SiO2 influences the grain growth. On the one hand, SiO2 promotes grain growth, on the other hand, SiO2 is nonmagnetic matrix material that physically isolates the magnetic FePt grains. When the thickness of SiO2 is smaller than 0.6 nm, the grain size increases due to the fact that SiO2 promotes grain growth. Therefore, the ordering of phase transformation is more complete and larger FePt grains are formed. When the thickness of SiO2 is larger than 0.6 nm, there are enough SiO2 matrixes to break the FePt grain layers and to isolate FePt grains, leading to the decrease of the grain size of FePt grain. From Fig.4 it can be found that Ku shows strong grain size dependence. Within this grain size range, smaller FePt grains are more difficult to become order, resulting in a much smaller Ku value[16] .
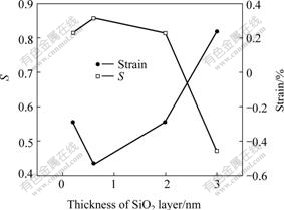
Fig.3 Order parameter (S) and strain of [SiO2 (x nm)/FePt (3 nm)]5/Ag (50 nm) thin films as function of SiO2 layer thickness
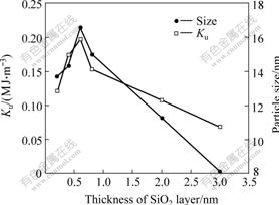
Fig.4 Perpendicular magnetic anisotropy (Ku) and particle size dependence on thickness of SiO2 layer
The decrease of grain size with increasing SiO2 concentration implies that SiO2 hinders grain growth. The decrease of Hc may be due to the decrease of the order parameter and grain size.
Actually, besides a high anisotropy, the high-density recording media also require magnetic grains isolated to reduce intergrain interactions, which leads to lower media noise. δM curve measurement, in which a positive value indicates intergranular exchange coupling and a negative one means dipolar interaction, is a common way to directly study interaction effects. δM curves of [SiO2 (x nm)/FePt (3 nm)]5/Ag (50 nm) (x=0, 0.6, 3.0) are shown in Fig.5. δM depends on the SiO2 content and the magnetic intensity. The trend is apparent that the intergrain interactions are very strong without SiO2 addition. It is observed that δM peak value of [SiO2 (0.6 nm)/FePt (3 nm)] 5/Ag (50 nm) is much smaller than that without SiO2 film. The reduction of intergrain exchange interaction can be attributed to the diffusion of proper SiO2 into the grain boundaries of FePt film. This indicates that nonmagnetic SiO2 matrix can separate FePt grains and decrease the intergranular interaction, and further reduce the media noise. With further increasing SiO2 concentration, a negative δM value means dipolar interaction.
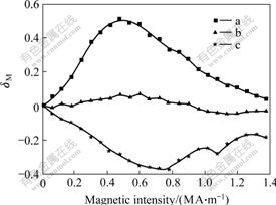
Fig.5 δM plots for FePt (15 nm)/Ag (50 nm) (a), [SiO2 (0.6 nm)/FePt (3 nm)]5/Ag (50 nm)(b) and [SiO2 (2.0 nm)/FePt (3 nm)]5/Ag (50 nm)(c)
4 Conclusions
1) Ag underlayer can induce phase transition from FCC to FCT of FePt film. SiO2-doping plays an important role in increasing the perpendicular magnetic anisotropy. Furthermore, the diffusion of Ag atoms together with SiO2 into the boundaries of FePt grain reduces the intergrain interaction.
2) When the thicknesses of FePt, SiO2 middle layer and Ag underlayer are respectively 3, 0.6 and 50 nm, the films have a high magnetic anisotropy, and highly (001)-oriented films are obtained in the SiO2 middle layer of 2.0 nm.
References
[1] CHRISTODOULIDES J A, HUANG Y, ZHANG Y, HADJIPANAYIS G C, PANAGIOTOPOULOS I, NIARCHOS D. CoPt and FePt thin films for high density recording media[J]. Journal of Applied Physics, 2000, 87: 6938-6940.
[2] SHAO Y, YAN M L, SELLMYER D J. Effects of rapid thermal annealing on nanostructure, texture and magnetic properties of granular FePt:Ag films for perpendicular recording[J]. Journal of Applied Physics, 2003, 93(10): 8152-8154.
[3] YU Hong-yun, SUN Hui-yuan, FENG Shun-zhen, GAO Feng-ju, ZHOU Hong-juan, NIE Xiang-fu, SUN Yu-ping. Microstructure and magnetic properties of granular FePt/(FePt)27Ti73 films for ultrahigh-density recording media[J]. J Cent South Univ Technol, 2005, 12(2): 155-158.
[4] CAO J W, CAI J, LIU Y, YANG Z, WEI F. Effect of CrW underlayer on structural and magnetic properties of FePt thin films[J]. Journal of Applied Physics, 2006, 99: 08F901-903.
[5] LI Bao-He, FENG Chun, YANG Tao, HWANG Pol, TENG Jiao, YU Guang-hua, ZHU Feng-wul. l. Approach to enhance the coercivity in perpendicular FePt/Ag nanoparticle film[J]. Journal of Applied Physics, 2006, 99: 0126101- 0126103.
[6] ZHANG Z G, KANG K, SUZUKI T. Magnetic and magneto-optical properties of ultra thin Fe50Pt50 films with Ag layers inserted[J]. Journal of Applied Physics, 2003, 93: 7163-7165.
[7] CHEN J S, LIM B C, WANG J P. Controlling the crystallographic orientation and the axis of magnetic anisotropy in L10 FePt films[J]. Applied Physics Letters, 2002, 81: 1848-1850.
[8] WAN J, HUANG Y, ZHANG Y, BONDER M J, HADJIPANAYIS G C, WELLER D. Particulate FePt/Ag(C) films with strong perpendicular anisotropy[J]. Journal of Applied Physics, 2005, 97: 10J1211-10J1213.
[9] LUO C P, SELLMYER D J. Structural and magnetic properties of FePt: SiO2 granular thin films[J]. Applied Physics Letters, 1999, 75(20): 3162-3164.
[10] LUO C P, LIOU S H, SELLMYER D J. FePt: SiO2 granular thin film for high density magnetic recording[J]. Journal of Applied Physics, 2000, 87(9): 6941-6943.
[11] XU Xiao-hong, WU Hai-shun, WANG Fang, LI Xiao-li. Structure and magnetic properties of FePt and FePt/Ag thin films deposited by magnetron sputtering[J]. Thin Solid Film, 2005, 472: 222-226.
[12] XU Xiao-hong, LI Xiao-li, WU Hai-shun. Microstructure and magnetic properties of [FePt/AlN]n multilayers deposited by RF magnetron sputtering[J]. Physics B, 2004, 352: 48-52.
[13] CHEN J, ISHIO S, SUGAWARA S. Preparation of L10 ordered CuAu buffer layer and its effect on the L10 ordering in FePt thin film[J]. Thin Solid Films, 2003, 426: 211-215.
[14] PING D H, OHNUMA M, HONO K, WATANABE M, IWASA T, MASUMOTO T. Microstructures of FePt-Al-O and FePt-Ag nanogranular thin films and their magnetic properties[J]. Journal of Applied Physics, 2001, 90(9): 4708-4716.
[15] WAN J, STOYANOV S, HUANG Y, ZHANG Y, HADJIPANAYIS G, SKUMRYEV V, WELLER D. Thickness dependence of coercivity in FePt/C multilayers[J]. Journal of Magnetism and Magnetic Materials, 2004, 272-276: 1625-1627.
[16] KANG K, ZHANG Z G, PAPUSOI C, SUZUKI T. (001) oriented FePt-Ag composite nanogranular films on amorphous substrate[J]. Applied Physics Letters, 2003, 82(19): 3284-3286.
(Edited by CHEN Wei-ping)
Foundation item: Project(10574085) supported by the National Natural Science Foundation of China; Project(207020) supported by the Science Technology Key Project of the Ministry of Education, China
Received date: 2007-06-12; Accepted date: 2007-08-29
Corresponding author: XU Xiao-hong, Professor; Tel: +86-357-2051842; E-mail: xuxh@dns.sxnu.edu.cn