J. Cent. South Univ. Technol. (2011) 18: 1418-1424
DOI: 10.1007/s11771-011-0856-6
Effect of chloride ion on bacterial pre-oxidation of arsenic-containing gold concentrate
YANG Wei(杨玮)1, QIN Wen-qing(覃文庆)1, LIU Rui-qiang(刘瑞强)2, REN Yun-chao(任允超)3
1. School of Resources Processing and Bioengineering, Central South University, Changsha 410083, China;
2. Refinery of Shandong Gold Mining Co., Ltd., Laizhou 261441, China;
3. Engineering Design and Research Co., Ltd., Shandong Gold Corporation, Yantai 264006, China
? Central South University Press and Springer-Verlag Berlin Heidelberg 2011
Abstract: The bacterial pre-oxidation process of arsenic-containing gold concentrates and the bacterial activity under different chloride ion concentrations were studied by using a mixture of thermophilic strains TCJ domesticated in production. The experimental result shows that with different samples and leaching systems, the adaptability and Cl- tolerance of bacteria are different, and that appropriate chloride ion concentration is conductive to bacterial oxidation, while higher chloride ion concentration will inhibit the bacterial activity and affect the pre-oxidation performance. Under the present production conditions, TCJ can adapt to the changes of water quality in the source of water and its critical chloride ion tolerance value is 2.7 g/L.
Key words: chloride ions; arsenic-containing gold concentrate; pre-oxidation; bacterial adaptation; critical concentration
1 Introduction
With the development of biotechnology, the biological treatment of refractory arsenic-containing gold concentrate receives more and more attention. The pre-oxidation of arsenic-containing gold concentrate is a complex four-phase system composed of liquid, solid, gas and bios with the existence of life [1]. Bacteria, as living creatures in the system, are the principal part and have their own special environmental requirements. The bacterial pre-oxidation reaction rate of arsenic-containing gold concentrate is mainly affected by the growing length and the activity of bacteria [2], so it is of great practical significance to study the content of hazardous substances in the biological pretreatment process.
Damages of ions to bacterial growth of leaching minerals in solution have been researched extensively [3-18]. For example, FAN et al [5] summarized the mechanism of bacterial oxidation on refractory gold ore containing arsenic in recent years and researched the influence of Fe2+, Fe3+, As3+, As5+, Cu2+, Ag+, Mg2+, Hg2+, Bi3+, Ni2+ and Co2+. ZHU et al [6] summarized the research achievements on the damages of bacteria in leaching minerals in solution and found that cations such as As3+, Ag+, Hg2+ and Mo6+ would damage the bacteria in the form of delaying bacteria growing, thus reducing the leaching rate of gold, and that anions such as Cl- and SCN- would destroy the inner construction of bacteria in oxidation. WEI et al [7] proposed that other anions would inhibit the growth of bacteria to different degrees except that three kinds of anion, arsenious acid radical, arsenic acid radical and sulfate radical, did not have the inhibitory action on the growth of ferrous oxide thiobacillus. They listed the utmost tolerant density of microorganism to some metals and the ion limit, and pointed out that when the pulp content surpassed the enduring limit of the leaching bacteria, the growth and activeness of the microorganism were obviously lowered, and when their oxidizing ability was suppressed, the bacterium leaching effect dropped suddenly. The research performed by CHEN et al [8] indicated that the fluorine ion played a decisive influence on the bacterial activity, and along with the enlargement of nutrient fluid in fluorine ion concentration, the growth and the acid producing of the bacterium were delayed, with its growth quantity and oxidizing ability of sulfur greatly reduced. The fluorine enduring ability of the bacterium could be enhanced through domesticated way, and the resistant domesticated fluorine speed of bacteria could be improved through the leaching ore to select fine stains.
The bacterial oxidation process was adopted in a gold smelting plant in Shandong Province, China. With the increase of the processing capacity, a water shortage in the original site entailed new source of water. However, the water qualities in original and new sources were quite different. DOUGLAS [19] indicated that the important characteristic of the thermophilic bacteria was their high sensitivity to chloride ion, which was very important to the bacterial pre-oxidation, and set special requests for water quality in source water. So far, the effect of chloride ion on the bacterial pre-oxidation of arsenic-containing gold concentrate has been seldom reported. In this work, the tolerance of TCJ to different concentrations of chloride ions and the critical chloride concentration were examined in the bacterial pre-oxidation of arsenic-containing gold concentrate under the present production conditions.
2 Experimental
2.1 Ore samples
The ore sample used in the experiment was gold concentrate purchased from a gold plant in Liaoning Province, China. The sample was arsenic-containing and the arsenic mineral was mainly arsenopyrite. Gold was wrapped in pyrite or arsenopyrite in the form of micro-grains and it contained carbons in which organic carbon occupied 0.42% in total mass. Usually, the conventional leaching recovery of gold was 30%-40%, but in the pretreatment of bacterial oxidation, it was generally beyond 95%. The multi-element analysis result of the samples is listed in Table 1.
Table 1 Composition of sample (mass fraction, %)
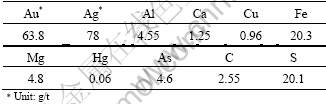
2.2 Bacteria
The TCJ used in the experiments was obtained from the oxidation tank at the production site. The bacteria introduced from Australia were a mixture of thermophilic strains with optimum growth temperature at 46 °C and they could survive for 3 d at 55 °C. The strains were acclimated before they were applied in production.
2.3 Experimental methods
The experiments were conducted in a single 5 L agitation tank, in which the initial pulp concentration was 2%, and the pulp pH value was adjusted with sulfuric acid to 2 before the inoculation was processed. The inoculums were adopted from the oxidation tank at the production site. The temperature was controlled at 40 °C; the aeration was 0.3 m3/h, and the ammonium sulfate, magnesium sulfate and potassium hydrogen phosphate were added as nutrients. The rtal sip of variables in i; 6—Fe2+; 7—PANedox potential (φ(vs SCE)), pH value and Fe2+ concentration were measured every 24 h. When the redox potential was above 590 mV (Hg/HgCl electrode) and Fe2+ concentration was below 30 mg/L, ore samples were added into the reaction tank. The ore samples was 80% passing through 400 mesh and gradually added.
2.4 Measurements
The redox potential was determined by the portable pH meter pHB-4 with Hg/HgCl-Pt electrode. The pH value was measured by the portable pH meter pHB-4 and its attached composite electrode. The Fe2+ concentration was indicated by 2% diphenylamine sulfonate. When it turned purple, the end of oxidization into Fe3+ by K2Cr2O7 was indicated.
3 Conditional experiment on bacterial oxidation with presence of chloride ion
The impact of chloride ion on the bacterial activity and the pre-oxidation process was studied to provide insight into the water utilization in production, and to propose the critical chloride ion concentration according to chloride-ion tolerance of acclimated TCJ bacteria. Therefore, in order to determine whether the new water source would have apparent impact on the bacterial oxidation, experiments were conducted with samples using water of the two sites and the mixed water. Also in order to get the critical chloride ion concentration, NaCl was added to determine the impact of chloride ion.
3.1 Adaptability test on quality of water source
Water samples for the experiment were separately taken from the original and new water sources, and the analysis showed that their chloride contents were 0.30 g/L and 0.78 g/L, respectively. Considering that the two water sources would both be used in the production, water from the two sources was mixed by the ratio of 1:1, with the chloride ion of 0.54 g/L. Therefore, biological pre-oxidation tests were arranged on three water sources from original, new and mixed in the reaction agitation tanks coded as 1#, 2# and 3# . Figure 1 represented the changes of redox potential and pH value in the bacterial oxidation using three different water sources. Figure 2 demonstrated the changes of [Fe2+] in the bacterial oxidation process under different conditions.
It could be seen from Fig.1 and Fig.2 that,
1) In the system, when the chloride ion con- centrations were 0.30, 0.54 and 0.78 g/L, respectively, the changing of the redox potential φ(vs SCE), pH value and [Fe2+] were almost consistent. Under the three different chloride ion concentrations, the increase of chloride concentration facilitated the bacterial oxidation reaction and shortens the oxidation time to some degree.
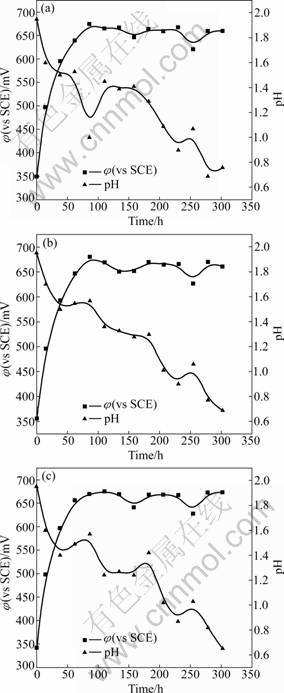
Fig.1 Curves of redox potential and pH in bacterial oxidation under different chloride ion concentrations: (a) 1# oxidation tank, original water, [Cl-]=0.30 g/L; (b) 2# oxidation tank, mixture of original and new water, [Cl-]=0.54 g/L; (c) 3# oxidation tank, new water, [Cl-]=0.78 g/L
2) In the bacterial oxidation system, the value of the redox potential (φ(vs SCE)) increased rapidly from the beginning, and remained stable when the potential rose to around 650 mV; while the pH value went down to a certain degree and leveled off, before the process was repeated. [Fe2+] kept stable after a rapid decrease and could be interpreted as follows.
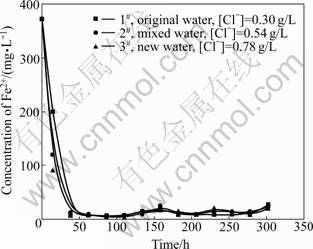
Fig.2 Changes of [Fe2+] in bacterial oxidation process under different chloride ion concentrations
(1) According to the mechanism of bacterial complex oxidation, Fe2+ in the solution was oxidized to Fe3+ by bacteria. Then, Fe3+ oxidized and decomposed the sulfide minerals and released Fe2+ which was oxidized to Fe3+ by bacteria again, thus a redox leaching cycle was formed [2]:
(1)
(2)
(3)
The arsenic-containing gold concentrate used in the experiment might be stacked longer and weathered, so at the beginning, [Fe2+] was high and oxidized to Fe3+ by the highly active TCJ from the oxidation tank, making c(Fe3+)/c(Fe2+) in the solution increase rapidly. It could be seen from the Nernst equation φ=φ0+2.3RT/ nFln(c(Fe3+)/c(Fe2+)) that the redox potential in the solution should increase linearly, and when the reaction reached its equilibrium, the redox potential (φ(vs SCE)) remained nearly unchanged.
(2) With the ongoing of the bacterial oxidation, sulfuric acid was produced by oxidizing a series of reduced sulfur, making the pH value decrease and
sharply increase in the solution. Fe3+ and
and OH- reacted in the solution and generated jarosite (MFe3(SO4)2(OH)6). The consumption of OH- in the solution made [H+] increase and pH value decrease, explaining the fluctuation of pH value.
It could be seen from the above experiments that TCJ from the production site was highly active and reacted quickly with the arsenic-containing gold concentrate. No obvious influence of chloride ion on the bacteria activity was seen. So, it is reasonable that TCJ bacteria could adapt to the water of the both sources and would not have a negative impact on production.
3.2 Test of critical value of chloride ion concentration
On the basis of the work done above, it was reasonable to do further experiments to obtain the critical value of chloride ion concentration affecting bacteria oxidation. Hence, the probe test of the TCJ’s tolerance of [Cl-] was carried out hereby. Controlled experiments were conducted in the 1#, 2# and 3# oxidation tanks used in the previous experiments. First experiment on chloride ion concentration was carried out in 1# oxidation tank, with 2# and 3# oxidation tanks as controlled ones. Then, on the basis of results received in 1# tank, the conditional experiment on the chloride ion concentration was carried out in 3#, with 2# tank as controlled one. Finally, the conditional verification test on the concentration of chloride ion was carried out in 2# tank. In these experiments, NaCl was used as source of chloride ion, and on the basis of the original concentration of chloride ions, the amount of NaCl needed was calculated to add to maintain the concentration needed in the following tests. Distilled water was used, and the redox potential (φ(vs SCE)), pH, Fe2+ concentration were measured and recorded everyday. The time of NaCl addition and the corresponding concentration were listed in Table 2.
3.2.1 [Cl-] exploratory tests
In 1# oxidation tank, when the reaction time reached 308 and 350 h, the calculated amount of NaCl was added to keep [Cl-] at the level of 2 and 4 g/L, while 2# and 3# tanks were used as control tests. Potential φ(vs SCE) curve and [Fe2+] curve were shown in Fig.3 and Fig.4.
As can be seen from Fig.3 and Fig.4, when the concentration of Cl- stood at 2 g/L, the redox potential was on the rise and [Fe2+] increased slightly, but the increment of potential was much smaller than that in the control test and [Fe2+] was a little greater than that in the control test, which showed that TCJ was still able to adapt to such concentration and the bacterial oxidation still worked. When [Cl-] reached 4 g/L, the redox potential dramatically decreased from 653 to 545 mV while [Fe2+] sharply increased from 15 to 444 mg/L, showing that at this level, the activity of bacteria was severely inhibited and the rate of oxidation dropped significantly, exerting great influence on the redox process. In the light of this, when the concentration of chloride ion was from 2 to 4 g/L, the redox process was severely inhibited, thus [Cl-] conditional tests were carried out within this range.
3.2.2 [Cl-] conditional tests
In 3# oxidation tank, when the reaction time reached 422, 470 and 494 h, the calculated amount of NaCl was added to keep [Cl-] at the level of 2, 2.5 and 3 g/L, respectively, while 2# tank was used for control test. Potential φ(vs SCE) curve and [Fe2+] curve were shown in Fig.5 and Fig.6.
As can be seen from Fig.5 and Fig.6, the redox potential in 3# oxidation tank was lower than that in 2# oxidation tank, and when [Cl-] was increased, this difference became larger. In 3# oxidation tank, when [Cl-] was at the level of 2-2.5 g/L, the potential was relatively stable; when [Cl-] was at 2.5-3 g/L, the inflection point was observed and the redox potential dropped dramatically. [Fe2+] in 3# oxidation tank was almost equal to that in 2# tank when [Cl-] was in the range of 2- 2.5 g/L; However, when [Cl-] was 2.5-3 g/L, the inflection point was observed and [Fe2+] was increased significantly. Therefore, it was obvious that in the range of 2.5-3 g/L, [Cl-] would affect the activity of bacteria, so within this range verification tests would be conducted.
3.2.3 [Cl-] conditional verification tests
Similar to the previous experiments, when the reaction time was 542, 566, 590, 614, 638 and 662 h, the calculated amount of NaCl was added to 2# oxidation tank to conduct conditional verification tests at the levels of 2.5, 2.6, 2.7, 2.8, 2.9 and 3.0 g/L for [Cl-]. The potential φ(vs SCE) curve and [Fe2+] curve were shown in Fig.7.
Table 2 Time of NaCl addition and corresponding chloride ion concentration
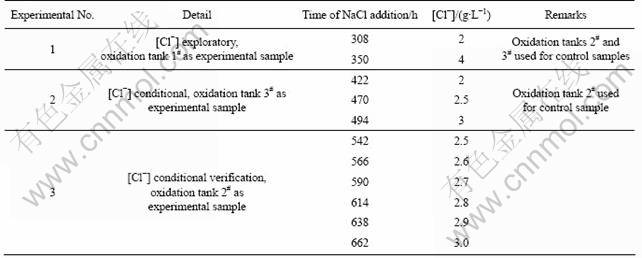
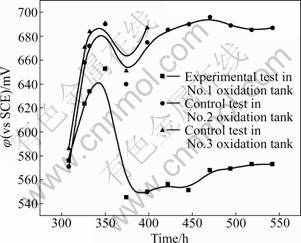
Fig.3 Potential φ(vs SCE) curve as function of time
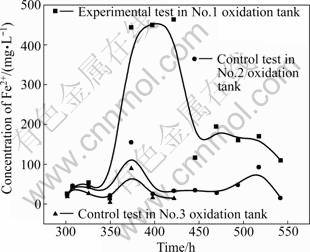
Fig.4 [Fe2+] curve as function of time
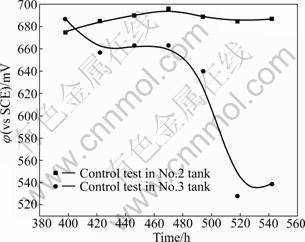
Fig.5 Potential φ(vs SCE) curve in 3# oxidation tank of [Cl-] conditional test
As can be seen from Fig.7, when the concentration of Cl- was 2.5-2.7 g/L, the redox reaction stayed normal. The potential was relatively high and [Fe2+] was low, although potential and [Fe2+] were both stable. When [Cl-] exceeded 2.7 g/L, potential decreased dramatically while [Fe2+] increased significantly. Therefore, the critical chloride ion concentration should be 2.7 g/L in the light of all the experiments done above.
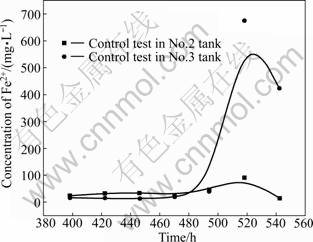
Fig.6 [Fe2+] curve in 3# oxidation tank of [Cl-] conditional test
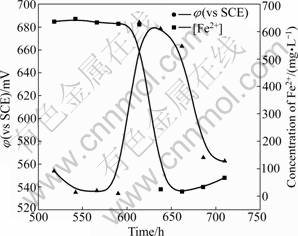
Fig.7 Redox potential and [Fe2+] curves in 2# oxidation tank [Cl-] verification test
4 Discussion
1) When the concentration of chloride ions is relatively low, as the concentration of chloride goes up, the bacterial oxidation is accelerated to some degree. This can be attributed to the salt effect [20] and catalysis [21-22] of Ag+ and Hg2+. The so-called salt effect happens when the solubility of insoluble electrolytes increases as soluble electrolyte is added to the solution. Of course, the salt effect includes salt addition, and it can also happen when strong acid or alkali is added in the precondition of no other reactions. In this study, arsenic-bearing gold concentrate contains little soluble products such as Ag and Hg sulfides. AgCl, Ag2S, HgS and other sediments might be produced in acidic bacterial oxidation environment, and salt effect would occur in NaCl addition, accelerating the dissolution of AgCl, Ag2S and other sediment. [Ag+] then will increase in solution, while Ag2S, HgS and Ag+ can produce catalyst effect on the bacterial leaching of metal sulfide. Because Ag2S, and HgS have much higher potential than other metal sulfides, primary cells are formed and promote the bioleaching. Ag+, Hg2+ and other metal cations could replace Fe2+ from the lattice of arsenic-bearing gold concentrate. Fe3+, oxidized from Fe2+ by bacteria, could oxidize arsenic-bearing gold concentrate.
2) When the chloride ions increase to a certain level, they may inhibit bacterial oxidation from two perspectives. First, Cl- may undermine the bacterial membrane and thus affect its activity [23]. In addition, in the process of bacterial oxidation, the precipitation of jarosite will impede the further oxidation of minerals and subsequent cyanide leaching [21]. In the strong acid environment at 40 °C, the addition of NaCl and H+ in solution may form HCl, which is highly volatile, so the [OH-] in solution increases, forming jarosite (MFe3(SO4)2(OH)6) with Fe3+ and
In the tests where [Cl-] is high, the strong smell of acid validates the produce of HCl.
3) Some researchers claim that when [Cl-] is in the range of 0-5 g/L, no evidence of bacterial oxidation inhibition is found [21]. But the study made by LAWSON et al shows that when the concentration of Cl- is 5 g/L, it will greatly reduce the oxidation activity of ferrooxidans on Fe2+ [23]. While in Australia, a test on a concentrate shows that the best performance of the oxidation of sulfide and cyanide leaching of gold is achieved at the concentration of 1.3-1.5 g/L Cl- [21]. In this study, however, it is shown that the oxidation is reasonable when the concentration does not exceed the critical value of 2.7 g/L. Through examination and analysis of the oxide slag, As content in the oxide slag is reduced to 0.36% from 4.6%, mass loss of solid after bacterial oxidation is about 50%, and the oxidation ratio of As is 96.08%. Fe content in oxide slag decreases from 20.3% to 1.8% and the oxidation ratio of Fe is 96.06%. S content in the oxide slag decreases from 20.1% to 4.8% and its oxide ratio is 88.06%. So, it is obvious that under different systems, bacteria have different adaptability and tolerance of Cl-. Therefore, it is quite necessary to conduct a study on the effects of Cl- on the process of bacterial oxidation in the light of production practice.
5 Conclusions
1) TCJ can adapt to the change of water quality in water sources, showing good compatibility with production requirement. To a certain degree, TCJ shows relatively strong adaptability to Cl- and its salt tolerance is conductive to the industrial production.
2) Because of different raw materials and leaching system, different bacteria have different adaptabilities and tolerance levels on the Cl-. As far as the conditions inferred in this study, TCJ can be tolerant to a critical concentration of Cl- of 2.7 g/L. Therefore, in the production practice, monitoring of chloride ion concentration in oxidation system should be enhanced and the utilization of backwater in the precondition of doing no harm to production to maximize the economic benefits would have a positive impact on the water-deficient areas and sustainable development.
References
[1] YANG Song-rong, QIU Guan-zhou, Hu Yue-hua, XIE Ji-yuan. The bio-oxidation process of arsenic refractory gold ore and its application [M]. Beijing: Metallurgical Industry Press, 2006: 60-63, 20. (in Chinese)
[2] YANG Hong-ying, YANG Li. Bacterium metallurgy [M]. Beijing: Chemical Industry Press, 2006: 76, 72. (in Chinese)
[3] KLAUS B. Bioleaching: Metal solubilization by microorganisms [J]. FEMS Microbiology Reviews, 1997, 20(3): 591-604.
[4] ZHANG Su-wen. The purification of thiobacills ferrooxidans and their tolerance to heavy metals [J]. Jiangxi Copper Engineering, 1998(3): 34-37. (in Chinese)
[5] FAN You-jing, YANG Hong-ying, ZI Jian-wei. The influence of the ions in solution on the growth of bioleaching engineering bacteria [J]. Non-ferrous Mining and Metallurgy, 2004, 20(2): 17-19. (in Chinese)
[6] ZHU Chang-liang, YANG Hong-ying, JIANG Huan-jie, WANG Da-wen. The toxicant influence of the ions in solution on leaching bacteria [J]. Non-ferrous Mining and Metallurgy, 2005, 21(5): 25-27. (in Chinese)
[7] WEI De-zhou, ZHU Yi-min, LI Xiao-an. The application of the biotechnology in mineral processing [M]. Beijing: Metallurgical Industry Press, 2008: 63, 69. (in Chinese)
[8] CHEN Mao-chun, LIANG Bin, ZHANG Yong-kui. Influence of fluorine ion on acidithiobacillus thiooxidans [J]. Sichuan Chemical and Corrosion Control, 2001, 4(2): 14-16. (in Chinese)
[9] TONG Lin-lin, JIANG Mao-fa, YANG Hong-ying, YIN Shu-yan. Cadmium-resistance of ore bioleaching bacteria [J]. Journal of Northeastern University: Natural Science, 2007, 28(10): 1434-1437. (in Chinese)
[10] YAO Jing, XU Wen-jing, LI Hong-yu. Transportation characterization of copper ion in adapted Thiobacillus ferrooxidans [J]. The Chinese Journal of Nonferrous Metals, 2005, 15(12): 2009-2015. (in Chinese)
[11] ZHANG Han-bo, ZHENG Yue, ZENG Fan, ZHU Zhi-ying, WANG Jie. Resistance and adsorption of several bacterial strains to heavy metals [J]. Microbiology, 2005, 32(3): 24-29. (in Chinese)
[12] TONG Lin-lin, JIANG Mao-fa, YANG Hong-ying, FAN You-jing. Study on growth control of bioleaching thermophilic strain HQ0211 by zinc ions [J]. Journal of Northeastern University: Natural Science, 2007, 28(4): 0525-0528. (in Chinese)
[13] SUN Wei, LI Jun-wen. Researches progress of promoting the material and the mechanism of germs growth [J]. Progress in Microbiology and Immunology, 2002, 30(1): 90-92. (in Chinese)
[14] GAHAN C S, SUNDKVISTA J E, SANDSTROM A. A study on the toxic effects of chloride on the biooxidation efficiency of pyrite [J]. Journal of Hazardous Materials, 2009, 172: 1273-1281.
[15] JIANG Tao, ZHANG Yu-zu, YANG Yong-bin, HUANG Zhu-cheng. Influence of copper minerals on cyanide leaching of gold [J]. Journal of Central South University of Technology, 2001, 8(1): 24-28.
[16] MAO An-zhang, QIU Guan-zhou, DENG Zhen-ling, SHE Xi-chang, XUJing, WANG Dian-zuo. One-step leaching of some refractory gold concentrate containing arsenic and theory analysis [J]. Journal of Central South University of Technology, 1997, 4(2): 92-95.
[17] YANG Hong-ying, GONG En-pu YANG Li, CHEN Gang, FAN You-jing, ZHAO Yu-shan, L? Jiu-ji. XPS characteristics of sulfur of bio-oxidized arsenic-bearing gold concentrate and changes of surface nature of bio-oxidation residue [J]. Transactions of Nonferrous Metals Society of China, 2004, 14(6): 1187-1191.
[18] MIN Xiao-bo, CHAI Li-yuan, CHEN Wei-liang, YU Xia, ZHANG Chuan-fu, KUANG Zhong. Bioleaching of refractory gold ore: Ⅲ. Fluid leaching Jinya refractory gold concentrate by Thiobacillus ferrooxidans [J]. Transactions of Nonferrous Metals Society of China, 2002, 12(2): 334-339.
[19] DOUGLAS E R. Biomining: Theory, microbes and industrial processes. [M] Springer-Verlag and Landes Bioscience, 1997: 229-245.
[20] Department of Inorganic Chemistry, Dalian University of Technology. Inorganic chemistry [M]. Beijing: Higher Education Press, 2005: 163. (in Chinese)
[21] YANG Xian-wan, SHEN Qing-feng, GUO Yu-xia. Microorganism hydrometallurgy [M] Beijing: Metallurgical Industry Press, 2008: 127, 185. (in Chinese)
[22] HU Yue-hua, ZHANG Zai-hai, QIU Guan-zhou, WANG Dian-zuo. Catalystic effect of Ag+ on bacterial leaching [J]. Mining and Metallurgical Engineering, 2001, 21(1): 24-28. (in Chinese)
[23] LAWSON E N, NICHOLAS C J, PELLAT H. The toxic effect of chloride ions on Thiobacillus ferrooxidans [M]// Biohydrometallurgical Processing. Santiago: University of Chile Press, 1995: 165-174.
(Edited by YANG Bing)
Foundation item: Project(2007AA060902) supported by the National High Technology Research and Development Program of China; Project (2010CB630905) supported by the National Basic Research Program of China
Received date: 2010-09-06; Accepted date: 2011-01-11
Corresponding author: YANG Wei, PhD Candidate; Tel: +86-18901127887; E-mail: ywmsco@126.com