
J. Cent. South Univ. (2020) 27: 2507-2514
DOI: https://doi.org/10.1007/s11771-020-4471-2

Recovery of Hg(II) from aqueous solution by complexation-ultrafiltration using rotating disk membrane and shear stability of PMA-Hg complex
ZHOU Han(周韩), QIU Yun-ren(邱运仁), CHEN Yu-xin(陈雨欣)
School of Chemistry and Chemical Engineering, Central South University, Changsha 410083, China
Central South University Press and Springer-Verlag GmbH Germany, part of Springer Nature 2020
Abstract: Copolymer of acrylic acid and maleic acid (PMA) was used to remove Hg2+ from aqueous solution by complexation-ultrafiltration (C-UF) through rotating disk membrane (RDM). The effects of P/M (mass ratio of PMA to metal ions), pH and rotation speed (N) on the interception of Hg2+ were investigated. The interception could reach 99.7% at pH 7.0, P/M 6 and N less than 1890 r/min. The shear stability of PMA-Hg complex was studied by RDM. The critical rotation speed, at which the interception starts to decrease, was 1890 r/min, and the critical shear rate, the smallest shear rate at which PMA-Hg complex begins to dissociate, was 2.50×105 s-1 at pH 7.0. Furthermore, the critical radii were obtained at different rotation speeds and pHs. The results showed that the critical radius decreased with the rotation speed and increased with pH. Shear induced dissociation coupling with ultrafiltration (SID-UF) was efficiently used to recover Hg2+ and PMA.
Key words: complexation-ultrafiltration; shear induced dissociation; rotating disk membrane; shear stability; wastewater treatment
Cite this article as: ZHOU Han, QIU Yun-ren, CHEN Yu-xin. Recovery of Hg(II) from aqueous solution by complexation-ultrafiltration using rotating disk membrane and shear stability of PMA-Hg complex [J]. Journal of Central South University, 2020, 27(9): 2507-2514. DOI: https://doi.org/10.1007/s11771-020-4471-2.
1 Introduction
Large amount of wastewater containing Hg2+ is produced from various chemical industries, such as chloralkali, oil refining and rubber processing [1]. Even trace amounts of Hg2+ may cause serious physiological complications to human as prenatal brain damage and motion disorders for its high biological toxicity [2]. Mercury as a highly toxic metal has aroused widespread attention for researchers to recover it from wastewater. Previous methods for removing Hg2+ from wastewater, such as ion exchange, chemical flocculation, sulfide precipitation, reduction and adsorption, almost possess the defects of high operation cost, limited raw materials, especially not suitable for treating low concentration wastewater and not helpful for the recovery [3-8].
Complexation–ultrafiltration (C-UF), a promising method with low energy requirements and high removal efficiency, is of great significance in treating wastewater containing heavy metals [9]. In the process of C-UF, metals are bounded with polymers to form macromolecular complexes that can be trapped by ultrafiltration membrane so as to achieve the purpose of removing metals from wastewater [10]. Nevertheless, the traditional C-UF method needs to consume large amounts of acids and alkalis in the subsequent dissociation of the polymer-metal complexes and recovery of metals. However, shear induced dissociation coupling with ultrafiltration (SID-UF) using RDM module, a dynamic membrane filtration system for providing shear force, reducing membrane fouling and producing higher permeate flux [11], can directly recover both metals and complexants at neutral condition according to the difference of the shear stabilities of polymer-metal complexes, avoiding the superfluous consumption of acid and alkali. SID-UF is especially suitable for the treatment of low concentration solutions, which can be considered a promising alternative owing to its high selectivity, low cost and without secondary pollution [12, 13].
Copolymer of acrylic acid and maleic acid (PMA), an excellent water soluble polymer, was used as complexant for removing Hg2+ by C-UF. The effects of rotation speed, pH and P/M (mass ratio of PMA to metal ions) on the Hg2+ interception efficiency were investigated. Furthermore, the shear stability of PMA-Hg complex was studied, and the critical shear rate (γc), the smallest shear rate when the complex starts to dissociate, was calculated. What is more, the recovery of Hg2+ and complexants was simultaneously fulfilled by SID-UF.
2 Experimental
2.1 Equipment, membrane and chemicals
The laboratory apparatus, called RDM module, is displayed in Figure 1. The volume of column-shaped feed tank is 4 L, and the volume flow of the feed is 30 L/h. If there is no special explanation, it is adjusted by a peristaltic pump. The feed port situates from the center of the upper metal plate 70 mm, and the outlet for discharging intercepted liquid is located at the center of the lower metal plate. In the course of the experiment, the permeate is gathered into a small beaker and weighed timely, and synchronously the retentate is sent back to the feed tank. The main component of RDM module is a six-rectangular-blade metal disk in a cylindrical shell. The six-blade rotating metal disk with radius 83 mm and thickness 4 mm, can be speeded up to 3000 r/min around the shaft driven by an electric motor. The effective area of the planar ultrafiltration membrane with the outer radius of 0.088 and the inner radius of 0.005 m is 0.0253 m2, and the planar ultrafiltration membrane is placed on the lower metal plate of the shell.
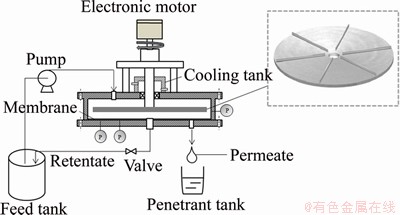
Figure 1 Scheme of filtration apparatus
Copolymer of acrylic acid and maleic acid (PMA) with MW (molecular weight) 70 kDa is from Shenyang Xingqi Daily Chemicals Plant, China, used as the complexant. The PES (polyether sulfone) ultrafiltration membrane with MWCO (molecular weight cut-off) 20 kDa, is purchased from Shanghai Yuling Filter Equipment Co, Ltd., China. Mercury nitrate purchased from Tianjin Guangfu Fine Chemical Research Institute is applied for preparing Hg2+ dilute solution of 10 mg/L. The pH is regulated by sodium hydroxide and hydrochloric acid, both of which are 0.1 mol/L. All of the experimental solutions in this process are prepared by pure water, and all experiments are performed at 25 °C.
2.2 Pretreatment experiment of PMA
The chemical structure of PMA is shown in Figure 2, and the carboxyl group is the only characteristic functional group. Although the PMA average MW is 70 kDa, there exists a little amount of small fractions which can pass through the membrane. Thus, the original PMA should be pretreated by continuous diafiltration to remove the low molecules. The PMA solution is pretreated by the PES hollow ultrafiltration membrane until no polymer is detected in the permeate [14, 15].
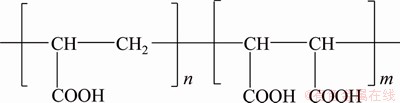
Figure 2 Chemical structure of PMA
2.3 Ultrafiltration process
The PMA solution, of which the initial Hg2+ concentration is 10 mg/L, is pumped into the RDM system by means of the peristaltic pump, and the temperature is maintained 25 °C. Then, the permeate solution is sampled to determine the mercury concentration using ICP-OES. The interception of Hg2+ can be calculated with following formula:

where Cf and Cp are the Hg2+ concentration (mg/L) in the feed and permeate, respectively.
3 Results and discussion
3.1 Selection of suitable pH and P/M
The variation of Hg2+ interception (R) with P/M and pH at rotation speed of 400 r/min is shown in Figure 3, which represents that pH and P/M have significant effects on removal efficiency of Hg2+. The interception rises from 23% to 99.7% with pH increasing from 3.0 to 7.0 at P/M 6. The phenomenon can be explained as the strong impact on the competitive complexation of hydrogen ions in dilute solution. Only small amounts of Hg2+ complexed with carboxyl group can be rejected by the membrane due to the protonation of carboxylate ions at low pH, while the degree of deprotonation increases at high pH, promoting the formation of PMA-Hg complex [16]. However, precipitation of hydroxide appears when the pH is too high, causing severe membrane fouling [17]. Thus, pH 7.0 is chosen as the suitable one, and the highest interception can be reached according to Figure 3.
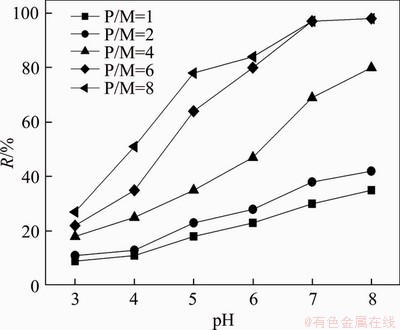
Figure 3 Effect of pH on interception of Hg2+ at diverse P/M
The permeability coefficient F [L/(m2·kPa·h)] is interpreted as the flux of permeate per unit trans- membrane pressure and is expressed as follows:
(2)
Rt=Rm+Rf+Rc (3)
where ΔP represents the trans-membrane pressure (kPa); J is the flux of permeate; μ is the solution dynamic viscosity (Pa·s), which can be detected by Ukrainian viscometer; Rm and Rt are the membrane intrinsic resistance and the total resistance (m-1), respectively, and the former can be measured through pure water test; Rc and Rf are the concentration polarization and the membrane fouling resistance (m-1), respectively, which can be both omitted at the experimental conditions because of the fierce shear action at the membrane surface produced by the high rotation blades [18].
The influence of P/M on Hg2+ interception and F at pH 7.0 and 400 r/min is displayed in Figure 4. The interception increases with P/M while F decreases softly, which can be explained as follows: more complexation sites for Hg2+ can be provided at greater P/M, improving the interception, but the viscosity of the solution also increases with P/M, causing the decline of the permeate flux simultaneously. Therefore, P/M 6 is adopted as the suitable operating condition.
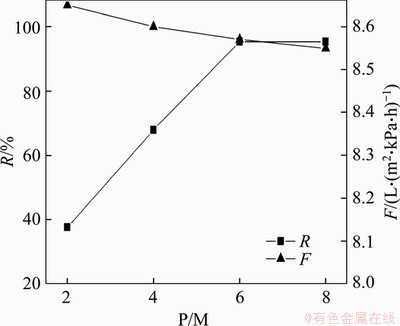
Figure 4 Effect of P/M on the interception of Hg2+ and permeability coefficient
3.2 Radial distribution of shear rate on membrane surface
The formulas for calculating the shear rate for turbulent and laminar flow conditions in the rotating disk device are as follows [18]:
(4)
(5)
where γmt and γmL are the shear rate on the membrane surface for turbulent and laminar flow states (s-1), respectively; and υ is the kinematic viscosity (m2/s); r represents the radius (m); ω is the angular velocity (rad/s) of the shaft connected to the metal disk; k is the velocity coefficient, determined by peripheral pressure at different rotation speeds. The relation between k and peripheral pressure (gage pressure) can be presented as follows:
P=0.0055ρk2N2r2+P0 (6)
where P is the pressure at the membrane surface (kPa, gauge pressure); the rotation speed N (r/min) can be controlled by the electric motor; ρ indicates the density of the solution (kg/m3); P0, the pressure on the axis of symmetry (kPa, gage pressure), is the same as the value of peripheral pressure at 0 r/min.
Figure 5 reflects the impact of N on pressures based on formula (6), showing that the pressure at three different locations on the membrane surface increases with the rising of N. k values obtained at the three positions of r=0.029, 0.059 and 0.088 m are 0.788, 0.800 and 0.799, respectively, which is consistent with the previous studies [19]. Thus, as for the six-blade rotating metal disk, the average 0.796 is selected as the suitable k.
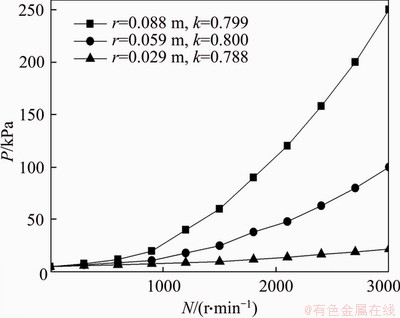
Figure 5 Variations of pressures on membrane surface with rotation speed
The radial distribution of shear rate on the membrane surface is calculated by Eqs. (4) and (5), shown in Figure 6. The shear rate increases from 0 to 5.78×105 s-1 when the rotation speed increases from 0 to 3000 r/min. The shear rate varies with the radial position at a given rotation speed, which is similar to the previous findings [20].
3.3 Shear stability of PMA-Hg complex
The PMA-metal complexes may dissociate under the action of larger shear force, leading to the decline of interception [12]. Therefore, it is of great importance to study the stability of PMA-Hg complex under external shear field to improve the interception as well as the recovery of PMA.
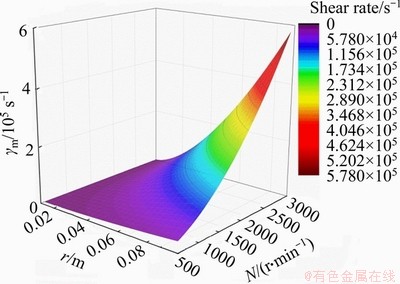
Figure 6 Radial distribution of shear rate on membrane surface
Figure 7(a) shows the variation of Hg2+ interception with rotation speed from 0 to 2500 r/min at P/M 6 and various pHs. The critical rotation speed (Nc) increases with pH from 5.0 to 7.0. The PMA-Hg complex would dissociate and the interception starts to decrease obviously as soon as the rotation speed exceeds Nc. On the contrary, the complex may keep stable when the rotation speeds are below 1380, 1630 and 1890 r/min at pH 5.0, 6.0 and 7.0, respectively. That is to say, the shear stability of PMA-Hg complex improves with pH [16]. The effect of rotation speed and P/M on the interception is revealed in Figure 7(b). At pH 7.0, the interception increases from 48.1% to 99.7% with P/M from 2 to 6. However, Nc maintains 1890 r/min independent of P/M, indicating that P/M has no effect on the shear stability of the PMA-Hg.
The shear stability of PMA has been demonstrated in previous study [16]. The results have shown that the interception of PMA can be maintained almost 100% even at a high rotation speed, which can explain that the shear force produced by the rotating disk can only break the binding bond between the metals and the polymers rather than the chain of the polymers.
3.4 Calculation of critical shear rate
The critical shear rate γc of PMA-Hg complex, the smallest shear rate at which PMA-Hg complex begins to dissociate, can be obtained by following algebraic expression, deduced by TANG et al [19]:
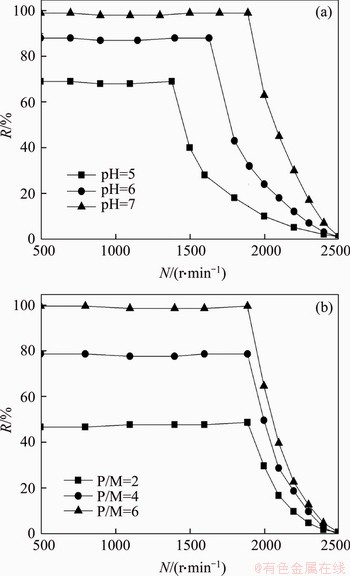
Figure 7 Effect of rotation speed on interception of Hg2+ at diverse pHs (a) and P/M (b)
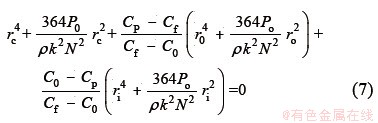
where Cp and C0 are the Hg2+ concentrations of the permeate at dynamic and rest on the rotating disk, respectively; ri and ro are the inner and outer membrane radius, which are 0.005 and 0.088 m, respectively; rc is the critical shear radius. The critical shear rate can be calculated according to critical shear radius (rc) according to Eq. (5).
Under the condition of diverse pHs, the relation between rc and N is shown in Figure 8. rc reduces with N but increases with pH. Besides, rc is non-existent or rc is greater than the outer radius of the membrane when rotation speed is at the low level, and PMA-Hg complex can keep stable. Nc and γc remain constant at certain pH. The algebraic relation between rc and N at diverse pHs can be calculated by Eq. (7) as shown in Table 1, visually demonstrating that γc values of PMA-Hg complex are 1.42×105, 1.91×105 and 2.50×105 s-1 at pH 5.0, 6.0 and 7.0, respectively. The increase of pH may improve the shear stability of PMA-Hg complex obviously and the PMA-Hg complex can withstand the maximum shear force at pH 7.0, which can give guidance to the selection of the industrial delivery pumps and the rotation speed of centrifugal pumps. Furthermore, the relation between Nc and the shear stability of PMA-Hg complex can lay a foundation for the recovery of Hg2+ and the polymer.
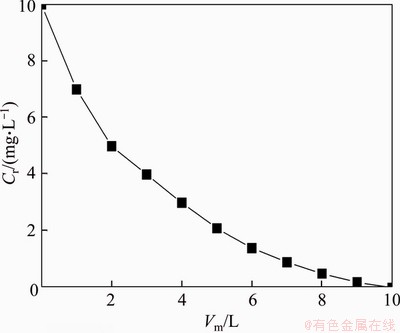
Figure 8 Relationship of the critical radius and rotation speed
Table 1 γc of PMA-Hg complex and correlations of rc and N at diverse pH
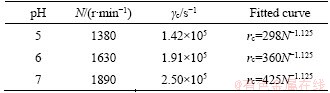
3.5 Recovery of Hg2+ and PMA
The recovery of Hg2+ and PMA is fulfilled by SID-UF from PMA-Hg complex solution at pH 7.0 and P/M 6. Along the process of recovery, the permeate is discharged continuously and the same volume of pure water is added into feed tank simultaneously to keep the feed stock volume constant. The relation between Hg2+ concentration in the retentate (Cr) and the supplemental water volume (Vm) is displayed in Figure 9. Suitable rotation speed, greater than Nc, should be used for the dissociation of PMA-Hg complex so that Hg2+ can be recovered from PMA-Hg complex solution. 2400 r/min is chosen as the suitable rotation speed for the recovery of Hg2+ and PMA considering the dissociation rate of PMA-Hg complex and energy consumption. Hg2+ is almost completely removed when Vm arrives at 9, and the PMA is regenerated in the retentate.
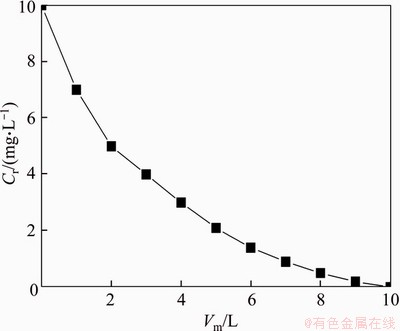
Figure 9 Variation of Hg2+ concentration in retentate with supplemental water volume
In order to demonstrate the complexation property of the regenerated PMA, the influence of cycle times of PMA on the interception of Hg2+ is obtained as shown in Figure 10. After conducting the regenerated PMA to reuse through the C-UF process at the optimal operating conditions about 10 cycle times, the interception is found to be 99.2% by 10 times of regeneration compared with the original 99.7%, proving that the regenerated PMA has almost the same complexation property as the originate. In the regenerated PMA, there is very little amount of PMA-Hg complexes, leading to a slight drop of interception. Therefore, SID-UF can fulfill the recovery of Hg2+ and PMA through controlling the rotation speed to dissociate the PMA-Hg complex at neutral condition by RDM, avoiding the superfluous consumption of acid and alkali.
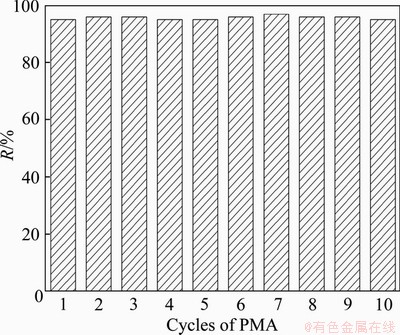
Figure 10 Variation of interception of Hg2+ with cycles of PMA
4 Conclusions
Copolymer of acrylic acid and maleic acid (PMA) was successfully applied to remove Hg2+ from dilute solutions using complexation- ultrafiltration (C-UF) by means of RDM. Effects of P/M (mass ratio of PMA to metal ions), pH and rotation speed (N) on the interception of Hg2+ were investigated, and the suitable pH and P/M were 7.0, 6, respectively, at which the interception was almost 99.7% when the rotation speed was lower than 1890 r/min, the critical rotation speed of PMA-Hg complex. In addition, the shear stability of PMA-Hg complex was explored using RDM, and the radial distribution of shear rate on the membrane surface as well as the critical shear rate was calculated. The results show that the critical shear rates, the smallest shear rates at which PMA-Hg complex begins to dissociate, are 1.42×105, 1.91×105 and 2.50×105 s-1 at pH 5.0, 6.0, 7.0, respectively. The recovery of Hg2+ and PMA is successfully achieved by SID-UF, and the regenerated PMA was proved to have almost the same complexation property as the original.
Contributors
ZHOU Han: Doing the experiment and writing the manuscript; CHEN Yu-xin: Doing part of the experiment; QIU Yun-ren: Giving guidance, reviewing.
Conflict of interest
ZHOU Han, CHEN Yu-xin and QIU Yun-ren declare that they have no conflict of interest.
References
[1] HADAVIFAR M, BAHRAMIFAR N, YOUNESI H. Adsorption of mercury ions from synthetic and real wastewater dilute solution by functionalized multi-walled carbon nanotube with both amino and thiolated groups [J]. Chemical Engineering Journal, 2014, 237: 217-228. DOI: 10.1016/j.cej.2013.10.014.
[2] WU Wen-qin, JIA Ming-ming, WANG Zhong-zheng, ZHANG Wen, ZHANG Qi. Simultaneous voltammetric determination of cadmium(II), lead(II), mercury(II), zinc(II), and copper(II) using a glassy carbon electrode modified with magnetite (Fe3O4) nanoparticles and fluorinated multiwalled carbon nanotubes[J]. Microchimica Acta, 2019, 186(2): 1-10. DOI: 10.1007/s00604-018-3216-5.
[3] DIL EBRAHIM A, GHAEDI M, ASFARAM A. The performance of nanorods material as adsorbent for removal of azo dyes and heavy metal ions: Application of ultrasound wave, optimization and modeling [J]. Ultrasonics Sonochemistry, 2017, 34: 792-802. DOI: 10.1016/j.ultsonch. 2016.07.015.
[4] ARSHADI M, MOUSAVINIA F, KHALAFI-NEZHAD A. Adsorption of mercury ions from wastewater by a hyperbranched and multi-functionalized dendrimer modified mixed-oxides nanoparticles [J]. Journal of Colloid and Interface Science, 2017, 505: 293-306. DOI: 10.1016/j.jcis.2017.05.052.
[5] LONE S, YOON D, LEE H, CHEONG I. Gelatin-chitosan hydrogel particles for efficient removal of Hg2+ from wastewater [J]. Environmental Science: Water Research and Technology, 2019, 5(1): 83-90. DOI: 10.1039/ C8EW00678D.
[6] LIU Yang-shuo, LI Ming, HE Chi-yang. Removal of Cr(VI) and Hg(II) ions from wastewater by novel β-CD/MGO- SO3H composite [J]. Colloids and Surfaces A: Physicochemical and Engineering Aspects, 2017, 512: 129-136. DOI: 10.1016/j.colsurfa.2016.10.025.
[7] KAHRIZI H, BAFKAR A, FARASATI M. Effect of nanotechnology on heavy metal removal from dilute solution [J]. Journal of Central South University, 2016, 23(10): 2526-2535. DOI: 10.1007/s11771-016-3313-8.
[8] LI Yan, SUN Ping. Experimental research on mercury- containing wastewater by fly ash [J]. Applied Mechanics and Materials, 2014, 456: 559-563. DOI: 10.4028/www. scientific.net/AMM.456.559.
[9] ZENG Jian-xian, YE Hong-qi, HUANG Nian-dong, LIU Jun-feng, ZHENG Li-feng. Selective separation of Hg(II) and Cd(II) from dilute solutions by complexation- ultrafiltration process [J]. Chemosphere, 2009, 76(5): 706-710. DOI: 10.1016/j.chemosphere.2009.05.019.
[10] CHEN Liang, QIU Yun-ren. Removal of Cd(II) from dilute dilute solutions by complexation–ultrafiltration using rotating disk membrane and the shear stability of PAA–Cd complex [J]. Chinese Journal of Chemical Engineering, 2019, 27(3): 519-527. DOI: 10.1016/j.cjche. 2018.06.026.
[11] WU Su-en, HWANG Kuo-jen, CHENG Tung-wen, TUNG Kuo-lun. Structural design of a rotating disk dynamic microfilter in improving filtration performance for fine particle removal [J]. Journal of the Taiwan Institute of Chemical Engineers, 2019, 94: 43-52. DOI: 10.1016/ j.jtice.2018.04.032.
[12] GAO Jing, QIU Yun-ren, HOU Ben, ZHANG Qiang, ZHANG Xiao-dong. Treatment of wastewater containing nickel by complexation-ultrafiltration using sodium polyacrylate and the stability of PAA-Ni complex in the shear field [J]. Chemical Engineering Journal, 2018, 334: 1878-1885. DOI: 10.1016/j.cej.2017.11.087.
[13] GAO Jing, QIU Yun-ren, LI Mao-lin, LE Hui-shang. Removal of Co(II) from dilute solution by complexation- ultrafiltration and shear stability of PAA-Co complex [J]. Transactions of Nonferrous Metals Society of China, 2019, 29(6): 1346-1352. DOI: 10.1016/S1003- 6326(19)65041-7.
[14] LE Hui-shang, QIU Yun-ren, TANG Shu-yun. Removal of Sn2+ using copolymer of maleic acid-acrylic acid (PMA) by complexation-ultrafiltration and regeneration of PMA using shear induced dissociation and ultrafiltration [J]. Desalination and Water Treatment, 2019, 160: 41-49. DOI: 10.5004/dwt.2019.24242.
[15] XU Jing-yuan, TANG Shu-yun, QIU Yun-ren. Pretreatment of poly (acrylic acid) sodium by continuous diafiltration and time revolution of filtration potential [J]. Journal of Central South University, 2019, 26(3): 577-586. DOI: 10.1007/ s11771-019-4029-3.
[16] ENNIGROU D J, SIK ALI M B, DHAHBI M. Removal of heavy metals from dilute solution by polyacrylic acid enhanced ultrafiltration [J]. Desalination and Water Treatment, 2015, 56(10): 2682-2688.
[17] BASSYOUNI M, ABDEL-AZIZ M H, ZOROMBA M S, ABEDL-HAMID S M S, DRIOLI E. A review of polymeric nanocomposite membranes for water purification [J]. Journal of Industrial and Engineering Chemistry, 2019, 73: 19-46. DOI: 10.1016/j.jiec.2019. 01.045.
[18] TANG Shu-yun, QIU Yun-ren. Removal of copper(II) ions from dilute solution s by complexation–ultrafiltration using rotating disk membrane and the shear stability of PAA–Cu complex [J]. Chemical Engineering Research and Design, 2018, 136: 712-720. DOI: 10.1016/j.cherd.2018.06.030.
[19] TANG Shu-yun, QIU Yun-ren. Removal of Zn(II) by complexation-ultrafiltration using rotating disk membrane and the shear stability of PAA-Zn complex [J]. Korean Journal of Chemical Engineering, 2018, 35(10): 2078-2085. DOI: 10.1007/s11814-018-0105-x.
[20] HWANG K J, WU S E. Disk structure on the performance of a rotating-disk dynamic filter: A case study on microalgae microfiltration [J]. Chemical Engineering Research and Design, 2015, 94: 44-51. DOI: 10.1016/j.cherd.2014. 12.009.
(Edited by YANG Hua)
中文导读
丙烯酸-马来酸共聚物(PMA)强化络合超滤处理含汞稀溶液及PMA-Hg络合物剪切稳定性
摘要:用丙烯酸-马来酸共聚物(PMA)作络合剂,通过旋转盘膜(RDM)采用络合-超滤处理含汞稀溶液。研究了溶液pH、P/M(PMA与金属离子质量比)和转速对汞离子截留率的影响。研究表明,在pH 7.0、P/M 6及旋转盘转速小于1890 r/min的条件下,汞离子的截留率可达到99.7%。此外,研究了PMA-Hg络合物在剪切场中的稳定性。当溶液pH 为7.0时,PMA-Hg络合物的临界转速(汞离子的截留率开始下降时的最小转速)为1890 r/min,临界剪切速率为2.50×105 s-1。此外,得到了在不同转速和pH条件下的临界半径。结果表明,临界半径随着转速的增大而减小,随着pH值的增加而增大。通过剪切诱导耦合超滤(SID-UF)方法实现了从PMA-Hg络合物溶液中分离、回收Hg2+和PMA。
关键词:络合-超滤;旋转盘膜;剪切诱导解络;剪切稳定性;废水处理
Foundation item: Project(21476265) supported by the National Natural Science Foundation of China
Received date: 2020-01-14; Accepted date: 2020-06-16
Corresponding author: QIU Yun-ren, PhD, Professor; Tel: +86-731-88879616; E-mail: csu_tian@csu.edu.cn; ORCID: https://orcid.org/ 0000-0003-1705-5051