
Surface tension of molten Ni-(Cr, Co, W) alloys and segregation of elements
XIAO Feng(肖 锋)1, LIU Lan-xiao(刘兰霄)1, YANG Ren-hui(杨仁辉)1,
ZHAO Hong-kai(赵红凯)1, FANG Liang(方 亮)2, ZHANG Chi(张 弛)1
1. Materials Interfacial Physical-Chemistry Research Institute, Chongqing Institute of Technology,
Chongqing 400050, China;2. Department of Applied Physics, Chongqing University, Chongqing 400044, China
Received 22 January 2008; accepted 26 May 2008
Abstract: Surface tension of molten Ni-(Cr, Co, W) alloys was measured at the temperature of 1 773-1 873 K in an Ar+3%H2 atmosphere using an improved sessile drop method. The segregation of Cr, Co and W in alloy was calculated and analyzed using Butler’s equation. The results show a good agreement between measured and calculated data. The surface tension of molten Ni-(Cr, Co, W) alloys decreases with increasing temperature. In Ni-(Cr, Co, W) alloys, the element with lower surface tension tends to segregate on the surface of molten alloy while that with higher surface tension tends to segregate inside of the molten alloy. The larger the differences in surface tension, atom radius and electron configuration between solvent and solute are, the more significant the segregation is. As a result, Ni segregates onto the surface and Co and W segregate inside the alloys.
Key words: Ni-Cr; Ni-Co; Ni-W; surface tension; sessile drop method; segregation
1 Introduction
Ni-based commercial alloys are widely used in high temperature and corrosive environments, such as gas turbine engines and heat exchangers. The thermophysical properties of molten alloys, such as surface tension, density and viscosity are necessary in casting process for getting their parts with excellent mechanical performance. The surface tension of molten metals and alloys dominates the thermal and hydrodynamic processes on the surface in the casting and welding processes, and their dependence on temperature results in the well-known Marangoni convection. There is particular interest in the surface tension of molten Ni-based alloys, such as Ni-Cr, Ni-Co and Ni-W, because they are used in the casting of critical components in gas-turbine engines. Because Ni-based alloys are very hard and difficult to be machined, the net-shape casting of the alloys is a cheap process. Therefore, it is essential to measure the thermophysical properties, such as surface tension, density and viscosity of molten Ni-based
alloys for obtaining low-cost net-shape casting ingot without inner porosity. With the advent of powerful mathematical modeling techniques for material phenomena, there is renewed interest in reliable data for the density of the Ni-based alloys. Up to now, although the surface tension of molten Ni-Cr [1-2], Ni-Cr-Al[1], Ni-Co[2-5], Ni-W[2], Ni-Si[2], Ni-Mo[2], Ni-Cu[2, 6-8], Ni-Ti[9-10], Ni-Zr[11], Ni-Fe [2-3, 12-16], Ni-Ge[17], Ni-C[10] and Ni-Au[18] alloys has been measured by several researchers using different methods, there is a large scatter in the available data due to systematic errors in different methods, impurities and surface-active elements such as oxygen and sulfur in the sample. The available data cannot be used in the mathematical modeling techniques for material phenomena. In order to obtain more accurate surface tension data for molten Ni-based alloys, the surface tensions of molten Ni-(Cr, Co, W) binary alloys were measured as a function of temperature in the range of 1 773-1 873 K on an alumina substrate in an Ar+3%H2 atmosphere in the present study.
2 Experimental
An improved sessile drop method[19-20] was adopted in this experiment. Fig.1 shows the experimental apparatus consisting of a vacuum chamber with a Ta cylindrical heater and five concentric Mo reflectors, a temperature program controller with a W-5%Ra/W-26%Rh thermocouple located close to the sample, an evacuating system including a rotary pump and a turbo molecular pump, two 10 mW He-Ne laser generators, a dropping device, two digital cameras and two band-pass filters, which were set between the camera and the sample to filtrate out the other light than He-Ne laser with a wavelength of 632 nm. The temperature at the place of the sample was calibrated using the melting-point of pure Ni.
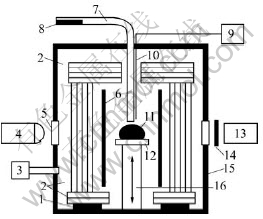
Fig.1 Schematic diagram of experimental apparatus: 1 Alumina support; 2 Mo reflector; 3 Evacuating system; 4 He-Ne laser generator; 5 Observation window; 6 Ta heater; 7 Alumina tube; 8 Sample before experiment; 9 Temperature controller; 10 Thermocouple; 11 Sample in experiment; 12 Substrate; 13 Digital camera; 14 Band-pass filter; 15 Chamber; 16 Sample elevator
Before the experiment, both the polycrystalline alumina substrate and the Ni or Ni-Co segment were ultrasonically cleaned 3 times in acetone for 3 min, then the alumina substrate was horizontally placed on the alumina support in the chamber while the Ni-based alloy specimen was placed in the sample container with a spring connector at the top of the dropping device outside the chamber. The chamber was first evacuated to a vacuum of about 5×10-4 Pa and then the temperature was elevated to the desired value. When the temperature reached the experimental one, the chamber was back-filled with a premixed Ar+3%H2 gas purified using platinum asbestos and magnesium perchlorate. After the temperature of the substrate was stable, the solid sample in the sample container was moved to the end of the alumina tube and molten down. By controlling the pressure difference in the chamber and the alumina tube, the molten sample was dropped smoothly onto the alumina substrate through a small hole machined at the bottom of the alumina tube.
A photo was taken as soon as the droplet separated from the bottom of the alumina tube. The surface tension was calculated by analyzing the photographs of the droplet using automatic image processing programs.
In the apparatus, two He-Ne laser generators and two high resolution photographic systems were used to record the shape of the droplet from two perpendicular directions at horizontal plane. As a result, it is easy to observe and find the distortion of droplet if it exists. The total error of the method is estimated as 84 mN/m[21].
3 Results and discussion
3.1 Surface tension of molten Ni-(Cr, Co, W) binary alloys
The surface tension of molten Ni-(Cr, Co, W) binary alloys measured in this study is shown in Fig.2 together with the values of Refs.[2-3].
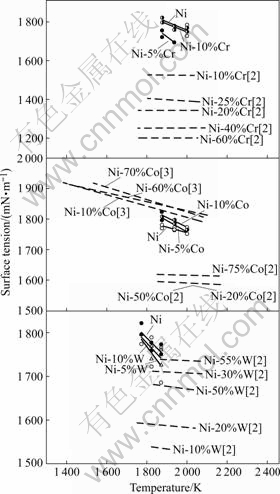
Fig.2 Temperature dependence of surface tension of molten Ni- (Cr, Co, W) binary alloys
For a certain alloy, the higher the temperature is, the more intensively the inner atoms thermally vibrate, and the weaker the interaction between atoms is. As a result, the surface tension of molten alloy decreases with increasing temperature.
Compared with that of MONMA et al[2] using sessile drop method in H2 atmosphere with MgO substrate, the surface tension of alloys is higher in this study. For example, the surface tension of Ni-10%Cr (mass fraction, %) and Ni-10%W in present work are much higher with the difference more than 200-220 mN/m. The purity of the alloy sample in the present work is 99.99% while that of MONMA et al[2] is 98% for Ni and 98%-99.9% for Cr, Co and W. An oxygen content of 7×10-6 corresponds roughly to a decrease of 30 mN/m in the surface tension of pure liquid Ni[8]. Thus, the impurity in sample such as oxygen and sulfur may cause the decrease in the surface tension of alloys.
The surface tension values of the Ni-10%Co alloy obtained in the present study are close to those of Ni-10%Co obtained by SEYHAN et al[3] using the oscillating droplet method although the surface tension values obtained by SEYHAN et al[3] are somewhat higher than those in this study. There are three reasons for this difference. The first is that a high-purity He-H2 atmosphere (99.9 999%) was used in the experiment of SEYHAN et al[3], while a He+3%H2 atmosphere (99.995%) was used in this experiment. The second is that any contact between the droplet and the substrate material and the contamination problem of specimens were avoided in the oscillating droplet method. It is possible to obtain somewhat higher surface tension values than that in the sessile drop method. The third is that the droplet is distorted from the spherical shape when the oscillating droplet method is used under terrestrial conditions. Consequently, the measured surface tension values tend to be larger[22].
The surface tension of molten metals and alloys could be represented as a function of temperature as follows[21]:
σ=σm+k(T-Tm) (1)
where σm, in mN/m, is the surface tension at liquidus temperature(Tm); k, in mN/(m×K), is the temperature coefficient of surface tension; and T is the temperature in Kelvin scale.
According to Eqn.(1), the recommended surface tension—temperature relationship was derived from linear regression of the surface tension data of molten Ni-based binary alloys measured in this work.
σNi=1 823-4.60×10-1(T-1 728) (2)
σNi-5Cr=1 784-9.00×10-1(T-1 725) (3)
σNi-10Cr=1 816-4.17×10-1(T-1 717) (4)
σNi-5Co=1 812-4.74×10-1(T-1 730) (5)
σNi-10Co=1 832-4.03×10-1(T-1 732) (6)
σNi-5W=1 802-5.80×10-1(T-1 743) (7)
σNi-10W=1 801-5.20×10-1(T-1 753) (8)
3.2 Calculation of surface tension of binary alloys using Butler model
Bulter’s equation[7] for surface tension is

where
and
are the chemical potentials of the surface, S, and the bulk, B, respectively. Eqn.(9) gives the surface tension as the difference of the chemical potentials of the surface, S, and the bulk, B, divided by a factor, giving the number of atoms per surface area.
The factor ai can be calculated from

where vi is the molar volume of pure metal and NA is the Avogadro constant. The molar volume of pure metal at different temperatures can be calculated from the molar mass and the temperature dependence of density.
The density of Ni is as follows[23]:

Because Cr, Co and W do not exist in the liquid state at the temperature lower than their melting points, it is difficult to strictly verify the surface tension in Eqn.(9). However, if one assumes that the relationship proposed by LEVIN et al [24] can be used to calculate the density of liquid Cr, Co and W in metastable state at the temperature lower than their melting points, the density of metastable Cr and Co can be calculated as follows[25]:

According to the density data of W at room temperature (19.3 g/cm3) and that at melting point (17.6 g/cm3)[26-27], the density of metastable W can be calculated as follows:

The procedure is now reduced to the problem of finding a model for the chemical potentials. For a binary alloy, the chemical potential can be split into two parts [7]:

One is responsible for the pure component, and the other is for all mixing effects. Then Eqn.(9) becomes

Because the first term is the surface tension of a pure metal, the surface tension of a binary alloy can also be

A very simple model can be made by taking the ideal solution model. Then,

where ciS and ciB are the concentrations of the different metals at surface and bulk, respectively. Eqn.(17) becomes

Namely, for a binary alloy,

The surface tension of pure Ni is taken from Ref.[21]:

Because Cr, Co and W do not exist in the liquid state at the temperature lower than their melting points, YAO et al[28] numerically predicted the surface tensions of undercooled liquid cobalt with the Monte Carlo method, where the surface tension was predicted. The surface tension and its relationship with temperature is

In the following calculations, the surface tensions of Cr and W at 1 773-1 873 K are substituted with that at melting point:

Now everything is determined. From the second equality in Eqn.(20) and c1S+c2S=1, one can calculate the surface concentrations c1S and c2S for known s1, s2, a1 and a2 as a function of the bulk concentration and the temperature. Then, from Eqn.(20), one can calculate the surface tension of Ni-10%(Cr, Co, W) binary alloys.
The calculation result of the temperature dependent on surface tension for the Ni-10%(Cr, Co, W) alloys is shown in Fig.3. It shows a good agreement between measurement and its deduction.
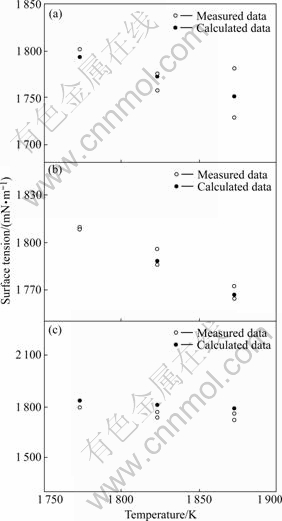
Fig.3 Temperature dependence of surface tension of molten Ni-10%(Cr, Co, W) alloys: (a) Ni-10%Cr; (b) Ni-10%Co;(c) Ni-10%W
The reasons for the difference are: 1) energy change of the system (mixing heat) not taken into account; 2) the measurement error; 3) the assumptions for a surface such as monolayer atom; 4) the differences in the energy of the Gibbs free energy of the surface.
3.3 Segregation of elements in molten alloys
As a melt, the alloy element does not distribute homogeneously in the melting bulk due to the different surface tensions of various elements from each other. Using Butler model, the element concentration on the surface and in the bulk can be calculated from the measured surface tension. The calculation result of the concentration dependent on the surface segregation of Cr, Co and W at 1 823 K is shown in Fig.4. Cr tends to segregate on the surface of molten alloys while Co and W tend to segregate inside molten alloy. Of the three alloy elements, segregation of W is the most serious. The segregation of elements may result from the differences in surface tension, atom radius and electron configuration between solvent and solute. The element with lower surface tension tends to segregate on the surface of molten alloy while the element with higher surface tension tends to segregate inside molten alloy. The larger the differences in surface tension, atom radius and electron configuration between solvent and solute are, the more significant the segregation is.
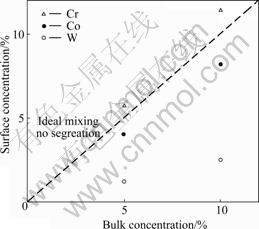
Fig.4 Surface segregation of Cr, Co and W at 1823 K
4 Conclusions
1) The surface tension of molten Ni-(Cr, Co, W) binary alloys was measured in the temperature range of 1 773-1 873 K using an improved sessile drop method with an alumina substrate in an Ar+3% H2 atmosphere. The surface tension of molten alloys decreases with increasing temperature. The calculated surface tension using Butler’s model is in good agreement with the measured data.
2) Cr tends to segregate on the surface of molten alloys while Co and W tend to segregate inside molten alloy. Of the three alloy elements, segregation of W is the most serious.
References
[1] KANETKAR C S, KACAR A S, STEFANESCU D M. The wetting characteristics and surface tension of some Ni-based alloys on yttria, hafnia, alumina, and zirconia substrates [J]. Metallurgical and Materials Transactions, 1988, 19A(7): 1833-1839.
[2] MONMA K, SUTO H. Experimental studies of surface tension of substitutional binary alloys [J]. J Japan Inst Metals, 1960, 24: 163-167.
[3] SEYHAN I, EGRY I. The surface tension of undercooled binary iron and nickel alloys and the effect of oxygen on the surface tension of Fe and Ni [J]. International Journal of Thermophysics, 1999, 20: 1017-1028.
[4] KEENE B J, MILLS K C, BROOKS R F. Surface properties of liquid metals and their effects on weldability [J]. Mater Sci Tech, 1985, 1(7): 568-571.
[5] OGINO K, TAIMATSU H, NAKATANI F. Surface tension of liquid Fe-Ni, Fe-Co and Ni-Co binary alloys and wettability of Al2O3 [J]. J Japan Inst Metals, 1982, 46: 1049-1054.
[6] ANUSIONWU B C, AKINLADE O, HUSSAIN L A. Assessment of size effect on the surface properties of some binary liquid alloys [J]. J of Alloys and Compounds, 1998, 278: 175-181.
[7] GORGES E, EGRY I. The surface tension of copper-nickel alloys [J]. J Mater Sci, 1995, 30: 2517-2520.
[8] NOGI K, CHUNG W B, MCLEAN A, Miller W A. Surface tension of liquid Fe-(Cu, Sn, Cr) and Ni-(Cu, Sn) binary alloys[J]. Mater Trans JIM, 1991, 32: 164-168.
[9] SAUERLAND S, LOHOFER G, EGRY I. Surface tension measurements on levitated liquid metal drops [J]. J Non-Crystalline Solids, 1993, 156/158: 833-836.
[10] ALLEN B C, KINGERY W D. Surface tension and contact angles in some liquid metal-solid ceramic systems at elevated temperatures [J]. Trans Metall Soc AIME, 1959, 215: 30-37.
[11] EGERY I, SCHWARTZ E, SZEKELY J, JACOBS G, LOHOEFER G, NEUHAUS P. Surface tension measurements on liquid metals in microgravity [J]. Metall Mater Trans, 1998, 29B: 1031-1035.
[12] JIMBO I, SHARAN A, CRAMB A W. Recent measurements of surface and interfacial tension in steel related systems [J]. Steelmaking Proceedings, 1993, 33: 485-494.
[13] POPEL S I, SHERGIN L M, TSAREVSKII B V. Temperature variation of the densities and surface tensions of iron-nickel melts [J]. Russian Journal of Physical Chemistry, 1969, 43: 1325-1328.
[14] SHARAN A, CRAMB A W. Surface tension and wettability study of Fe-Ni-O alloys [J]. Metall Mater Trans, 1997, 28B: 465-472.
[15] ECKLER K, EGRY I, HERLACH D M. Measurements of surface tension on levitated oscillating metallic drops [J]. Mater Sci Eng, 1991, A133: 718-721.
[16] LEE H K, FROHBERG M G, HAJRA J P. Surface tension measurements of liquid iron-nickel-sulphur ternary system using the electromagnetic oscillating droplet technique [J]. ISIJ International, 1993, 33: 833-838.
[17] NAIDICH Y V, ZABUGA V V. Concentration-capillary convection (marangoni effect) in liquid metals [J]. Izvestiya Akademii Nauk SSSR Metall, 1992, 4: 40-46.
[18] NIZHENKO V P, FLOKA L I, KHILYA G P. Surface properties of binary Au-(Fe, Co, Ni) melts [J]. Izvestiya Akademii Nauk SSSR Metall, 1991, 3: 43-46.
[19] NOGI K, OISHI K, OGINO K. Wettability of solid oxides by liquid pure metals [J]. Mater Trans JIM, 1989, 30: 137-145.
[20] FUJII H, NAKAE H, OKADA K. Interfacial reaction wetting in the boron nitride/molten aluminum system [J]. Acta Metall, 1993, 41: 2963-2971.
[21] XIAO Feng, FANG Liang, NOGI K. Surface tension and molten Ni and Ni-Co alloys [J]. Journal of Materials Science and Technology, 2005, 21(2): 201-206.
[22] FUJII H, MATUSMOTO T, HATA N. Surface tension of molten silicon measured by electromagnetic levitation method under microgravity [J]. Metall Mater Trans, 2000, 31A: 1585-1590.
[23] XIAO Feng. Density of liquid Ni-Cr alloy [J]. Journal of Materials Science and Technology, 2003, 19(1): 16-18.
[24] LEVIN E S, AYUSHINA G D. Study of some physico- chemical properties of Ni-Al melts [J]. Izvestiya Akademii Nauk SSSR Metall, 1971, 1: 227-229.
[25] XIAO Feng, MUKAI K, FANG Liang. Measurement and analyses of molten Ni-Co alloy density [J]. Trans Nonferrous Met Soc China, 2006, 16(6): 1263-1267.
[26] DAVID R L. CRC handbook of chemistry and physics 76th [M]. New York: CRC Press, 1995.
[27] LEE H G. Chemical thermodynamics for metals and materials [M]. London: Imperial College Press, 1999: 284.
[28] YAO W J, X J HAN, M CHEN, WEI B, GUO Z Y. Surface tension of undercooled liquid cobalt [J]. J Phys: Condens Matter, 2002, 14: 7479-7485.
Foundation item: Project(2004527) supported by Scientific Research Foundation for the Returned Overseas Chinese Scholars; Project(200594) supported by the Chongqing Bureau of Personal, China; Project(KJ070603) supported by the Municipal Education Commission of Chongqing, China; Project(2003ZD31) supported by Chongqing Institute of Technology, China; Project(CSTC2005BA4016-1) supported by Chongqing Science and Technology Commission, China
Corresponding author: XIAO Feng; Tel: +86-23-66966286; E-mail: xiaofeng@cqit.edu.cn
(Edited by LI Xiang-qun)